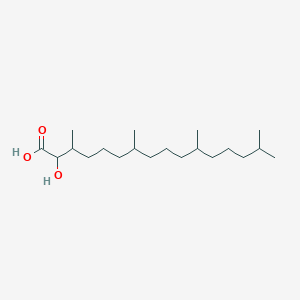
2-Hydroxyphytanic acid
Übersicht
Beschreibung
2-Hydroxyphytanic acid is a 20-carbon, methyl-branched hydroxy fatty acid. It is an intermediate in the degradation of phytanic acid and accumulates in patients with peroxisomal disorders .
Wissenschaftliche Forschungsanwendungen
2-Hydroxyphytanic acid has several scientific research applications:
Zukünftige Richtungen
The degradation of 2-Hydroxyphytanic acid suggests the existence of two pathways for decarboxylation of this compound . The first one, starting from this compound, involves the formation of 2-ketophytanic acid with only a small amount of pristanic acid being formed . The second pathway, which starts from 2-hydroxyphytanoyl-CoA, does not involve 2-ketophytanic acid and generates higher amounts of pristanic acid . The first pathway, which is peroxisomally localized, was found to be deficient in Zellweger syndrome, whereas the second pathway, localized in microsomes, was normally active . It is concluded that the second pathway is predominant under in vivo conditions .
Vorbereitungsmethoden
2-Hydroxyphytanic acid can be synthesized through the alpha-oxidation of phytanic acid. This process involves the conversion of phytanic acid to this compound by bacterial cytochrome P450 . In human liver, the degradation of this compound involves its conversion to 2-ketophytanic acid and pristanic acid . The reaction conditions typically include the presence of ATP, magnesium ions, and coenzyme A, which enhance the formation of pristanic acid .
Analyse Chemischer Reaktionen
2-Hydroxyphytanic acid undergoes several types of chemical reactions:
Oxidation: It can be oxidized to form 2-ketophytanic acid and pristanic acid.
Decarboxylation: This reaction leads to the formation of pristanic acid.
Hydroxylation: The alpha-hydroxylation of phytanic acid results in the formation of this compound.
Common reagents and conditions used in these reactions include NAD+, NADP+, and specific enzymes such as phytanate oxidase . The major products formed from these reactions are 2-ketophytanic acid and pristanic acid .
Wirkmechanismus
The mechanism of action of 2-hydroxyphytanic acid involves its conversion to pristanic acid through alpha-oxidation. This process is catalyzed by phytanate oxidase, which hydroxylates phytanic acid to form this compound . The this compound is then decarboxylated to form pristanic acid and carbon dioxide . This pathway is crucial in the metabolism of branched-chain fatty acids and is deficient in certain peroxisomal disorders .
Vergleich Mit ähnlichen Verbindungen
2-Hydroxyphytanic acid is similar to other hydroxy fatty acids such as:
Phytanic acid: A precursor in the alpha-oxidation pathway.
Pristanic acid: A product of the decarboxylation of this compound.
Hexadecanoic acid: A straight-chain fatty acid that shares structural similarities with this compound.
The uniqueness of this compound lies in its role as an intermediate in the alpha-oxidation of phytanic acid and its accumulation in peroxisomal disorders .
Eigenschaften
IUPAC Name |
2-hydroxy-3,7,11,15-tetramethylhexadecanoic acid | |
---|---|---|
Source | PubChem | |
URL | https://pubchem.ncbi.nlm.nih.gov | |
Description | Data deposited in or computed by PubChem | |
InChI |
InChI=1S/C20H40O3/c1-15(2)9-6-10-16(3)11-7-12-17(4)13-8-14-18(5)19(21)20(22)23/h15-19,21H,6-14H2,1-5H3,(H,22,23) | |
Source | PubChem | |
URL | https://pubchem.ncbi.nlm.nih.gov | |
Description | Data deposited in or computed by PubChem | |
InChI Key |
CGKMKXBKVBXUGK-UHFFFAOYSA-N | |
Source | PubChem | |
URL | https://pubchem.ncbi.nlm.nih.gov | |
Description | Data deposited in or computed by PubChem | |
Canonical SMILES |
CC(C)CCCC(C)CCCC(C)CCCC(C)C(C(=O)O)O | |
Source | PubChem | |
URL | https://pubchem.ncbi.nlm.nih.gov | |
Description | Data deposited in or computed by PubChem | |
Molecular Formula |
C20H40O3 | |
Source | PubChem | |
URL | https://pubchem.ncbi.nlm.nih.gov | |
Description | Data deposited in or computed by PubChem | |
DSSTOX Substance ID |
DTXSID60933062 | |
Record name | 2-Hydroxy-3,7,11,15-tetramethylhexadecanoic acid | |
Source | EPA DSSTox | |
URL | https://comptox.epa.gov/dashboard/DTXSID60933062 | |
Description | DSSTox provides a high quality public chemistry resource for supporting improved predictive toxicology. | |
Molecular Weight |
328.5 g/mol | |
Source | PubChem | |
URL | https://pubchem.ncbi.nlm.nih.gov | |
Description | Data deposited in or computed by PubChem | |
CAS No. |
14721-68-7 | |
Record name | 2-Hydroxy-3,7,11,15-tetramethylhexadecanoic acid | |
Source | CAS Common Chemistry | |
URL | https://commonchemistry.cas.org/detail?cas_rn=14721-68-7 | |
Description | CAS Common Chemistry is an open community resource for accessing chemical information. Nearly 500,000 chemical substances from CAS REGISTRY cover areas of community interest, including common and frequently regulated chemicals, and those relevant to high school and undergraduate chemistry classes. This chemical information, curated by our expert scientists, is provided in alignment with our mission as a division of the American Chemical Society. | |
Explanation | The data from CAS Common Chemistry is provided under a CC-BY-NC 4.0 license, unless otherwise stated. | |
Record name | 2-Hydroxyphytanic acid | |
Source | ChemIDplus | |
URL | https://pubchem.ncbi.nlm.nih.gov/substance/?source=chemidplus&sourceid=0014721687 | |
Description | ChemIDplus is a free, web search system that provides access to the structure and nomenclature authority files used for the identification of chemical substances cited in National Library of Medicine (NLM) databases, including the TOXNET system. | |
Record name | 2-Hydroxy-3,7,11,15-tetramethylhexadecanoic acid | |
Source | EPA DSSTox | |
URL | https://comptox.epa.gov/dashboard/DTXSID60933062 | |
Description | DSSTox provides a high quality public chemistry resource for supporting improved predictive toxicology. | |
Retrosynthesis Analysis
AI-Powered Synthesis Planning: Our tool employs the Template_relevance Pistachio, Template_relevance Bkms_metabolic, Template_relevance Pistachio_ringbreaker, Template_relevance Reaxys, Template_relevance Reaxys_biocatalysis model, leveraging a vast database of chemical reactions to predict feasible synthetic routes.
One-Step Synthesis Focus: Specifically designed for one-step synthesis, it provides concise and direct routes for your target compounds, streamlining the synthesis process.
Accurate Predictions: Utilizing the extensive PISTACHIO, BKMS_METABOLIC, PISTACHIO_RINGBREAKER, REAXYS, REAXYS_BIOCATALYSIS database, our tool offers high-accuracy predictions, reflecting the latest in chemical research and data.
Strategy Settings
Precursor scoring | Relevance Heuristic |
---|---|
Min. plausibility | 0.01 |
Model | Template_relevance |
Template Set | Pistachio/Bkms_metabolic/Pistachio_ringbreaker/Reaxys/Reaxys_biocatalysis |
Top-N result to add to graph | 6 |
Feasible Synthetic Routes
Q1: What is the role of 2-hydroxyphytanic acid in phytanic acid metabolism?
A: this compound is a crucial intermediate formed during the alpha-oxidation of phytanic acid. The pathway involves the initial alpha-hydroxylation of phytanic acid to form this compound. [] This step is followed by the conversion of this compound to pristanic acid through decarboxylation. [] These reactions occur within peroxisomes, cellular organelles crucial for various metabolic processes. []
Q2: How does the accumulation of this compound occur, and what are the associated diseases?
A2: The accumulation of this compound in plasma is a hallmark of specific peroxisomal disorders. These include:
- Rhizomelic Chondrodysplasia Punctata (RCDP): This severe disorder involves a deficiency in the enzyme responsible for this compound decarboxylation. Consequently, this compound builds up in the body. []
- Generalized Peroxisomal Disorders: These disorders affect multiple peroxisomal functions, including this compound decarboxylation and pristanic acid beta-oxidation, leading to the accumulation of both this compound and phytanic acid. []
- Single Peroxisomal Beta-Oxidation Enzyme Deficiencies: While not directly impacting this compound metabolism, deficiencies in enzymes responsible for pristanic acid beta-oxidation can indirectly hinder phytanic acid alpha-oxidation, leading to a buildup of this compound. []
Q3: What differentiates Refsum's disease from other disorders involving this compound metabolism?
A: Unlike RCDP and other disorders, Refsum's disease is characterized by a defect specifically in phytanic acid alpha-hydroxylase, the enzyme responsible for the initial conversion of phytanic acid to this compound. [, ] As a result, individuals with Refsum's disease accumulate phytanic acid but have normal or only slightly elevated levels of this compound. []
Q4: What is the significance of the discovery of 2-oxophytanic acid in this compound metabolism?
A: Research has identified 2-oxophytanic acid as another metabolite in the pathway. [, ] It is formed through the oxidation of L-2-hydroxyphytanic acid by a peroxisomal oxidase enzyme found in the rat kidney. [, ] This finding indicates an alternative route for this compound metabolism.
Q5: What are the subcellular locations involved in the different pathways of this compound decarboxylation?
A5: There are two identified pathways for this compound decarboxylation:
- Peroxisomal Pathway: This pathway, deficient in Zellweger syndrome, primarily involves the conversion of this compound to 2-ketophytanic acid, with a small amount of pristanic acid also being produced. [, ]
- Microsomal Pathway: This pathway, found to be functional in Zellweger syndrome, favors the conversion of 2-hydroxyphytanoyl-CoA to pristanic acid without involving 2-ketophytanic acid. [] This pathway appears to be the dominant route for this compound decarboxylation under normal physiological conditions. []
Q6: Can this compound be produced from phytanic acid by sources other than human cells?
A: Yes, research has shown that a bacterial cytochrome P450 enzyme from Sphingomonas paucimobilis can catalyze the alpha-hydroxylation of phytanic acid to form this compound. [, ] This finding suggests a potential role for bacterial enzymes in phytanic acid metabolism.
Q7: How is the production of formate and CO2 related to 2-hydroxyphytanoyl-CoA in phytanic acid alpha-oxidation?
A: Studies using purified rat liver peroxisomes have shown that the formation of 2-hydroxyphytanoyl-CoA, rather than the free acid, is associated with an increased production of formate and CO2. [] This observation supports the role of 2-hydroxyphytanoyl-CoA as a true intermediate in the alpha-oxidation pathway and highlights the importance of peroxisomes in this process.
Q8: How does 2-hydroxyacyl-CoA lyase deficiency (HACL1) differ from other peroxisomal disorders?
A: Mice deficient in HACL1, the enzyme responsible for cleaving 2-hydroxyphytanoyl-CoA into pristanal and formyl-CoA, do not exhibit severe phenotypes like those observed in other alpha-oxidation enzyme deficiencies, such as Refsum disease. [] Despite accumulating phytanic and 2-hydroxyphytanic acids in the liver, HACL1-deficient mice show no apparent clinical symptoms when fed a high-phytol diet. []
Q9: What compensatory mechanisms are activated in response to HACL1 deficiency?
A9: Proteomic analysis of HACL1-deficient mice revealed a significant upregulation of proteins involved in:
- PPAR signaling: This suggests an adaptive response to maintain lipid homeostasis. []
- Peroxisome proliferation: This indicates an attempt to increase the capacity for fatty acid oxidation. []
- Omega-oxidation: This alternative pathway for fatty acid degradation is activated to compensate for the blockage in alpha-oxidation. []
Q10: What are the implications of the upregulation of specific cytochrome P450 enzymes in HACL1 deficiency?
A10: The proteomic analysis of HACL1-deficient mice revealed:
Haftungsausschluss und Informationen zu In-Vitro-Forschungsprodukten
Bitte beachten Sie, dass alle Artikel und Produktinformationen, die auf BenchChem präsentiert werden, ausschließlich zu Informationszwecken bestimmt sind. Die auf BenchChem zum Kauf angebotenen Produkte sind speziell für In-vitro-Studien konzipiert, die außerhalb lebender Organismen durchgeführt werden. In-vitro-Studien, abgeleitet von dem lateinischen Begriff "in Glas", beinhalten Experimente, die in kontrollierten Laborumgebungen unter Verwendung von Zellen oder Geweben durchgeführt werden. Es ist wichtig zu beachten, dass diese Produkte nicht als Arzneimittel oder Medikamente eingestuft sind und keine Zulassung der FDA für die Vorbeugung, Behandlung oder Heilung von medizinischen Zuständen, Beschwerden oder Krankheiten erhalten haben. Wir müssen betonen, dass jede Form der körperlichen Einführung dieser Produkte in Menschen oder Tiere gesetzlich strikt untersagt ist. Es ist unerlässlich, sich an diese Richtlinien zu halten, um die Einhaltung rechtlicher und ethischer Standards in Forschung und Experiment zu gewährleisten.