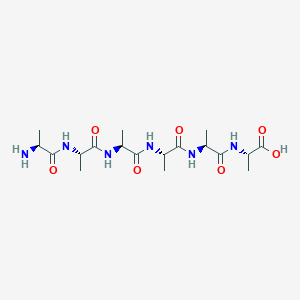
H-Ala-Ala-Ala-Ala-Ala-Ala-OH
- Klicken Sie auf QUICK INQUIRY, um ein Angebot von unserem Expertenteam zu erhalten.
- Mit qualitativ hochwertigen Produkten zu einem WETTBEWERBSFÄHIGEN Preis können Sie sich mehr auf Ihre Forschung konzentrieren.
Übersicht
Beschreibung
H-Ala-Ala-Ala-Ala-Ala-Ala-OH, also known as hexaalanine, is a peptide composed of six alanine residues linked together. Alanine is a nonpolar, aliphatic amino acid that plays a crucial role in protein structure and function. Hexaalanine is often used in scientific research to study peptide behavior, protein folding, and interactions due to its simplicity and repetitive structure.
Wissenschaftliche Forschungsanwendungen
Hexaalanine has several applications in scientific research:
Protein Folding Studies: Used as a model peptide to study the principles of protein folding and stability.
Peptide-Protein Interactions: Investigating how peptides interact with proteins, which is crucial for understanding biological processes and drug design.
Biomaterials: Serving as a building block for designing novel biomaterials with specific properties.
Drug Delivery: Exploring its potential as a carrier for drug delivery systems due to its biocompatibility and simplicity.
Wirkmechanismus
Target of Action
H-Ala-Ala-Ala-Ala-Ala-Ala-OH, a nonpolar hexapeptide, is primarily absorbed by human intestinal Caco-2 cells . The transport of alanine (Ala), like proton/amino acid symport, can lead to cytoplasmic acidification .
Mode of Action
The peptide interacts with its targets, the Caco-2 cells, through a process similar to proton/amino acid symport . This interaction leads to cytoplasmic acidification, which is a significant change in the cellular environment .
Pharmacokinetics
It’s known that the compound is absorbed by caco-2 cells , indicating its bioavailability in the human body.
Result of Action
The primary result of the action of this compound is the acidification of the cytoplasm of Caco-2 cells . This change in the cellular environment can have various downstream effects, potentially influencing cellular functions and signaling pathways.
Vorbereitungsmethoden
Synthetic Routes and Reaction Conditions
Hexaalanine can be synthesized using solid-phase peptide synthesis (SPPS), a common method for creating peptides. The process involves the sequential addition of protected amino acids to a growing peptide chain anchored to a solid resin. The typical steps include:
Attachment of the first amino acid: The first alanine residue is attached to the resin.
Deprotection: The protecting group on the amino acid is removed to expose the reactive amine group.
Coupling: The next alanine residue, protected at the amine group, is coupled to the growing chain using a coupling reagent like HBTU or DIC.
Repetition: Steps 2 and 3 are repeated until the desired peptide length is achieved.
Cleavage: The completed peptide is cleaved from the resin and deprotected to yield the final product.
Industrial Production Methods
Industrial production of hexaalanine follows similar principles but on a larger scale. Automated peptide synthesizers are often used to increase efficiency and consistency. The process involves optimizing reaction conditions, such as temperature, solvent, and reagent concentrations, to maximize yield and purity.
Analyse Chemischer Reaktionen
Types of Reactions
Hexaalanine can undergo various chemical reactions, including:
Hydrolysis: Breaking down the peptide into individual alanine residues using acidic or basic conditions.
Oxidation: Oxidizing agents can modify the side chains of alanine residues, although alanine is relatively resistant to oxidation due to its nonpolar nature.
Substitution: Introducing different functional groups to the peptide chain through nucleophilic substitution reactions.
Common Reagents and Conditions
Hydrolysis: Typically performed using hydrochloric acid or sodium hydroxide at elevated temperatures.
Oxidation: Hydrogen peroxide or other oxidizing agents can be used under controlled conditions.
Substitution: Reagents like alkyl halides or acyl chlorides can be used in the presence of a base to facilitate substitution reactions.
Major Products
Hydrolysis: Produces individual alanine residues.
Oxidation: Can result in modified alanine residues with altered side chains.
Substitution: Yields peptides with new functional groups attached to the alanine residues.
Vergleich Mit ähnlichen Verbindungen
Similar Compounds
H-Ala-Ala-Ala-OH: A tripeptide with three alanine residues, used in similar studies but with different structural properties.
H-Ala-Ala-Ala-Ala-OH: A tetrapeptide with four alanine residues, offering a balance between simplicity and complexity.
H-Ala-Ala-Ala-Ala-Ala-OH: A pentapeptide with five alanine residues, providing insights into peptide behavior with an additional residue.
Uniqueness
Hexaalanine’s uniqueness lies in its length and repetitive structure, making it an ideal model for studying peptide behavior and protein folding. Its simplicity allows for controlled experiments, while its length provides sufficient complexity to mimic real protein interactions.
Eigenschaften
IUPAC Name |
(2S)-2-[[(2S)-2-[[(2S)-2-[[(2S)-2-[[(2S)-2-[[(2S)-2-aminopropanoyl]amino]propanoyl]amino]propanoyl]amino]propanoyl]amino]propanoyl]amino]propanoic acid |
Source
|
---|---|---|
Source | PubChem | |
URL | https://pubchem.ncbi.nlm.nih.gov | |
Description | Data deposited in or computed by PubChem | |
InChI |
InChI=1S/C18H32N6O7/c1-7(19)13(25)20-8(2)14(26)21-9(3)15(27)22-10(4)16(28)23-11(5)17(29)24-12(6)18(30)31/h7-12H,19H2,1-6H3,(H,20,25)(H,21,26)(H,22,27)(H,23,28)(H,24,29)(H,30,31)/t7-,8-,9-,10-,11-,12-/m0/s1 |
Source
|
Source | PubChem | |
URL | https://pubchem.ncbi.nlm.nih.gov | |
Description | Data deposited in or computed by PubChem | |
InChI Key |
ZLHDFPIXRRJBKM-ZNSCXOEOSA-N |
Source
|
Source | PubChem | |
URL | https://pubchem.ncbi.nlm.nih.gov | |
Description | Data deposited in or computed by PubChem | |
Canonical SMILES |
CC(C(=O)NC(C)C(=O)NC(C)C(=O)NC(C)C(=O)NC(C)C(=O)NC(C)C(=O)O)N |
Source
|
Source | PubChem | |
URL | https://pubchem.ncbi.nlm.nih.gov | |
Description | Data deposited in or computed by PubChem | |
Isomeric SMILES |
C[C@@H](C(=O)N[C@@H](C)C(=O)N[C@@H](C)C(=O)N[C@@H](C)C(=O)N[C@@H](C)C(=O)N[C@@H](C)C(=O)O)N |
Source
|
Source | PubChem | |
URL | https://pubchem.ncbi.nlm.nih.gov | |
Description | Data deposited in or computed by PubChem | |
Molecular Formula |
C18H32N6O7 |
Source
|
Source | PubChem | |
URL | https://pubchem.ncbi.nlm.nih.gov | |
Description | Data deposited in or computed by PubChem | |
Molecular Weight |
444.5 g/mol |
Source
|
Source | PubChem | |
URL | https://pubchem.ncbi.nlm.nih.gov | |
Description | Data deposited in or computed by PubChem | |
Haftungsausschluss und Informationen zu In-Vitro-Forschungsprodukten
Bitte beachten Sie, dass alle Artikel und Produktinformationen, die auf BenchChem präsentiert werden, ausschließlich zu Informationszwecken bestimmt sind. Die auf BenchChem zum Kauf angebotenen Produkte sind speziell für In-vitro-Studien konzipiert, die außerhalb lebender Organismen durchgeführt werden. In-vitro-Studien, abgeleitet von dem lateinischen Begriff "in Glas", beinhalten Experimente, die in kontrollierten Laborumgebungen unter Verwendung von Zellen oder Geweben durchgeführt werden. Es ist wichtig zu beachten, dass diese Produkte nicht als Arzneimittel oder Medikamente eingestuft sind und keine Zulassung der FDA für die Vorbeugung, Behandlung oder Heilung von medizinischen Zuständen, Beschwerden oder Krankheiten erhalten haben. Wir müssen betonen, dass jede Form der körperlichen Einführung dieser Produkte in Menschen oder Tiere gesetzlich strikt untersagt ist. Es ist unerlässlich, sich an diese Richtlinien zu halten, um die Einhaltung rechtlicher und ethischer Standards in Forschung und Experiment zu gewährleisten.