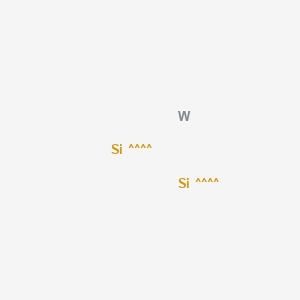
Tungsten disilicide
- Klicken Sie auf QUICK INQUIRY, um ein Angebot von unserem Expertenteam zu erhalten.
- Mit qualitativ hochwertigen Produkten zu einem WETTBEWERBSFÄHIGEN Preis können Sie sich mehr auf Ihre Forschung konzentrieren.
Übersicht
Beschreibung
Tungsten disilicide (WSi₂) is an intermetallic compound belonging to the transition-metal silicide family. It exhibits a tetragonal crystal structure (C11b type) and is characterized by high thermal stability, electrical conductivity, and oxidation resistance. With a melting point of 2160°C and a density of 9.3 g/cm³ (9300 kg/m³), WSi₂ is widely used in microelectronics, high-temperature coatings, and structural ceramics . Its resistivity ranges between 60–80 μΩ·cm, making it suitable for integrated circuit interconnects and gate electrodes .
Vorbereitungsmethoden
Synthetic Routes and Reaction Conditions: Tungsten silicide can be synthesized through several methods, including:
Chemical Vapor Deposition (CVD): This method involves the reaction of tungsten hexafluoride (WF(_6)) with silane (SiH(_4)) or silicon at high temperatures.
Solid-State Reaction: This involves the direct reaction of tungsten and silicon powders at high temperatures, typically above 1250°C.
Ion-Beam Mixing: A tungsten film is deposited on a silicon substrate, followed by ion implantation and rapid thermal annealing to form a smooth tungsten silicide layer.
Industrial Production Methods: In industrial settings, tungsten silicide is often produced using the CVD method due to its ability to create uniform thin films essential for microelectronic applications. The process involves the reduction of tungsten hexafluoride with hydrogen or silane, followed by deposition on a silicon wafer .
Types of Reactions:
Oxidation: Tungsten silicide can undergo oxidation at high temperatures, forming a protective layer of silicon dioxide (SiO(_2)) and tungsten trioxide (WO(_3)).
Common Reagents and Conditions:
Oxidation: Typically occurs at temperatures above 450°C in the presence of oxygen or air.
Reduction: Hydrogen gas is commonly used as a reducing agent at temperatures above 700°C.
Major Products:
Oxidation: Silicon dioxide (SiO(_2)) and tungsten trioxide (WO(_3)).
Reduction: Tungsten (W) and silicon (Si).
Wissenschaftliche Forschungsanwendungen
Microelectronics
Conductive Material
WSi₂ serves as a crucial conductive material in microelectronics. Its low resistivity (60–80 μΩ cm) makes it an excellent choice for contact materials in semiconductor devices. It is often used as a shunt over polysilicon lines to enhance conductivity and signal speed, facilitating faster electronic communication .
Barrier Layer
In addition to its use as a contact material, this compound acts as a barrier layer between silicon and other metals. This application is vital in preventing diffusion between silicon and metal contacts, thereby improving the reliability of microelectronic devices .
Thin Films for MEMS
WSi₂ is extensively utilized in microelectromechanical systems (MEMS) as thin films for the fabrication of microscale circuits. Its ability to be plasma-etched using gases like nitrogen trifluoride allows for precise patterning required in MEMS technology .
Thermoelectric Devices
Recent advancements have highlighted the potential of this compound in thermoelectric applications. A groundbreaking study demonstrated the first successful transverse thermoelectric conversion using WSi₂, indicating its capability to convert heat into electricity efficiently . This property positions WSi₂ as a promising candidate for developing advanced thermoelectric devices that can be used in sensors and energy harvesting systems.
Case Study: Transverse Thermoelectric Conversion
- Research Team : Tokyo University of Science
- Publication : PRX Energy, November 2024
- Findings : The study established that WSi₂ can effectively measure temperature and heat flow through transverse thermoelectric effects, paving the way for new sensor technologies .
High-Temperature Applications
Oxidation-Resistant Coatings
WSi₂ is recognized for its high emissivity and oxidation resistance, making it suitable for high-temperature applications such as heat shields and thermal protection systems. These properties are particularly advantageous in aerospace applications where materials are subjected to extreme conditions .
Application Area | Specific Use Case | Benefits |
---|---|---|
Microelectronics | Contact material and barrier layer | Low resistivity; prevents diffusion |
Thermoelectric Devices | Heat-to-electricity conversion | Efficient energy harvesting |
High-Temperature Materials | Oxidation-resistant coatings | Suitable for extreme environments |
Synthesis and Characterization
The synthesis of this compound can be achieved through various methods, including chemical vapor deposition (CVD) and thermal treatment at high temperatures. The optimal synthesis conditions typically involve heating mixtures of tungsten and silicon in an inert atmosphere .
Wirkmechanismus
The mechanism by which tungsten silicide exerts its effects is primarily related to its ability to form stable, low-resistivity contacts and protective coatings. The formation of a tungsten silicide layer at the tungsten-silicon interface occurs through a solid-state reaction at high temperatures, resulting in a stable and conductive layer . This layer enhances the performance and longevity of microelectronic devices and protective coatings.
Vergleich Mit ähnlichen Verbindungen
Comparison with Similar Transition-Metal Silicides
Physical and Thermal Properties
WSi₂ is compared with titanium disilicide (TiSi₂) , molybdenum disilicide (MoSi₂) , and tantalum disilicide (TaSi₂) . Key data are summarized in Table 1.
Table 1: Physical Properties of Selected Silicides
Property | WSi₂ | TiSi₂ | MoSi₂ | TaSi₂ | |
---|---|---|---|---|---|
Melting Point (°C) | 2160 | 1500 | 2030 | 2200 | |
Density (kg/m³) | 9300 | 4000 | 6200 | 9140 | |
Vickers Hardness (GPa) | 13.1 | 8.6 | 12.0 | 15.6 | |
Resistivity (μΩ·cm) | 60–80 | 15–25 | 21–40 | 35–50 |
- Melting Points : TaSi₂ exhibits the highest melting point (2200°C), followed closely by WSi₂ (2160°C). TiSi₂ has the lowest (1500°C), limiting its high-temperature applications .
- Density : WSi₂ is denser than MoSi₂ and TiSi₂ but slightly less dense than TaSi₂ .
- Hardness : TaSi₂ is the hardest (15.6 GPa), while TiSi₂ is the softest (8.6 GPa). WSi₂ and MoSi₂ show intermediate values .
Mechanical and Oxidation Behavior
- Mechanical Strength: In the MoSi₂-WSi₂ system, composites with 30–50 wt% WSi₂ exhibit lower porosity and higher flexural strength compared to pure MoSi₂.
- Oxidation Resistance :
- WSi₂ forms a protective SiO₂ layer at temperatures up to 800–1000°C , similar to MoSi₂. However, MoSi₂ is prone to "pesting" (catastrophic oxidation) below 500°C, while WSi₂ is less susceptible .
- TaSi₂ coatings remain stable up to 800°C but degrade faster than WSi₂ at higher temperatures due to SiO₂ volatility .
Research Findings and Challenges
- Composite Systems : MoSi₂-WSi₂ composites show promise for high-temperature ceramics but require advanced sintering techniques (e.g., SHS or plasma spraying) to achieve homogeneity .
- Oxidation Limits : While WSi₂ outperforms TiSi₂ and MoSi₂ in oxidation resistance above 800°C, its protective SiO₂ layer degrades above 1200°C, necessitating hybrid coatings (e.g., Si-MoSi₂) for prolonged service .
Biologische Aktivität
Tungsten disilicide (WSi2) is a compound with significant applications in various fields, particularly in electronics and materials science due to its unique properties. However, its biological activity has garnered attention in recent years, prompting investigations into its potential effects on living organisms. This article explores the biological activity of this compound, summarizing relevant research findings, case studies, and data tables that illustrate its interactions with biological systems.
This compound is a silicide of tungsten characterized by the formula WSi2. It is known for its high melting point, electrical conductivity, and thermal stability. These properties make it suitable for applications in semiconductor technology and as a material for high-temperature environments.
Table 1: Properties of this compound
Property | Value |
---|---|
Chemical Formula | WSi2 |
Melting Point | 2,600 °C |
Density | 9.3 g/cm³ |
Electrical Conductivity | High |
Thermal Conductivity | Moderate |
Toxicological Studies
Research indicates that this compound may exhibit varying degrees of toxicity depending on exposure levels and routes. A study highlighted the effects of tungsten compounds on biological systems, noting that tungsten can accumulate in tissues and potentially lead to adverse health effects.
- Absorption and Distribution : In animal studies, tungsten was found to be absorbed primarily through the gastrointestinal tract. The biological half-life was approximately 10 hours, with significant accumulation observed in the liver and kidneys .
- Effects on Organs : A notable study involving Wistar rats demonstrated that tungsten exposure could lead to increased incidences of mammary carcinoma when combined with carcinogenic agents .
Case Study: In Vivo Effects
A significant investigation involved administering this compound to rats to observe its effects on organ systems. The study revealed:
- Liver Effects : After administration, the highest concentration of tungsten was detected in the liver (0.03% of the administered dose), suggesting a potential hepatotoxic effect .
- Kidney Concentration : The kidney showed a rapid decrease in tungsten concentration compared to other tissues, indicating a possible mechanism for detoxification or excretion .
The mechanisms through which this compound exerts its biological effects are not fully understood but may involve oxidative stress pathways. Tungsten compounds have been shown to induce oxidative stress in cells, potentially leading to cellular damage and apoptosis.
- Oxidative Stress : Tungsten exposure has been associated with increased reactive oxygen species (ROS) production, which can damage cellular components such as DNA and lipids .
- Cell Membrane Disruption : The sharp edges of tungsten particles may disrupt cell membranes directly, leading to cell death .
Potential Therapeutic Applications
Despite concerns regarding toxicity, some studies suggest that this compound may have therapeutic potential due to its antibacterial properties. Research indicates that certain tungsten compounds can exhibit bactericidal activity against various pathogens.
Table 2: Antibacterial Activity of Tungsten Compounds
Pathogen | Minimum Inhibitory Concentration (MIC) |
---|---|
Escherichia coli | 25 µg/mL |
Staphylococcus aureus | 15 µg/mL |
Pseudomonas aeruginosa | 30 µg/mL |
This antibacterial effect could be harnessed for biomedical applications, particularly in developing coatings for medical devices or wound dressings.
Q & A
Basic Research Questions
Q. What are the key structural and electronic properties of tungsten disilicide relevant to microelectronics applications?
this compound (WSi₂) exhibits a tetragonal crystal structure with a density of 9.3 g/cm³ and a resistivity of 60–80 μΩ·cm, making it suitable as a conductive shunt over polysilicon lines to enhance signal speed. Its high melting point (2,160°C) and stability under high-temperature processing conditions are critical for semiconductor manufacturing. Characterization techniques include X-ray diffraction (XRD) for phase identification and scanning electron microscopy (SEM) for microstructural analysis .
Q. What standard synthesis methods are used for this compound thin films in academic research?
WSi₂ thin films are typically deposited via chemical vapor deposition (CVD) using tungsten hexafluoride (WF₆) and monosilane (SiH₄) or dichlorosilane (SiH₂Cl₂) as precursors. Process parameters such as temperature (optimized at ~1,000°C), gas flow ratios, and substrate selection significantly influence film stoichiometry and conductivity. Post-deposition annealing may be required to achieve desired crystallinity .
Q. How is the phase purity of this compound verified in experimental studies?
Phase purity is assessed using XRD to compare observed diffraction patterns with reference data (e.g., JCPDS files). For composite materials, energy-dispersive X-ray spectroscopy (EDS) coupled with SEM can confirm elemental composition. Cross-referencing with Raman spectroscopy or resistivity measurements ensures consistency with literature values .
Advanced Research Questions
Q. How do precursor gas ratios during CVD affect the stoichiometry and electrical properties of WSi₂ films?
Variations in WF₆:SiH₄ ratios can lead to non-stoichiometric films (e.g., W-rich or Si-rich phases), altering resistivity. Systematic studies involve tuning gas flows while monitoring film composition via EDS and electrical properties via four-point probe measurements. Excess Si may form insulating SiO₂ layers at interfaces, requiring optimization to balance conductivity and thermal stability .
Q. What experimental strategies resolve discrepancies in reported resistivity values for WSi₂?
Discrepancies often arise from differences in deposition conditions (e.g., precursor purity, substrate roughness) or measurement techniques (e.g., four-point probe vs. van der Pauw method). Researchers should replicate synthesis protocols from conflicting studies and perform cross-characterization (XRD, TEM) to identify impurities or crystallographic defects .
Q. What mechanisms drive the thermal degradation of WSi₂-based heterostructures in harsh environments?
In situ degradation studies reveal that selenium loss and silicon migration from substrates can initiate decomposition pathways, forming secondary phases like this compound. Techniques such as in situ transmission electron microscopy (TEM) under controlled atmospheres and post-mortem X-ray photoelectron spectroscopy (XPS) are critical to mapping degradation kinetics .
Q. How do alumina (Al₂O₃) or zirconia (ZrO₂) reinforcements influence the high-temperature stability of WSi₂ composites?
Incorporating 40–60 vol% Al₂O₃/ZrO₂ particles into WSi₂ matrices improves oxidation resistance by forming protective oxide layers. Researchers sinter composites at 1,600°C and analyze phase stability via XRD, while nanoindentation and creep tests quantify mechanical enhancements. SEM micrographs reveal particle distribution and interfacial bonding .
Q. What challenges arise when integrating WSi₂ into graphene-based heterostructures for nanoelectronics?
Interfacial reactions during CVD synthesis (e.g., carbon diffusion or silicide formation) can degrade graphene’s electronic properties. Mitigation strategies include using sacrificial buffer layers or low-temperature deposition. Electrical characterization (e.g., Hall effect measurements) and Raman spectroscopy assess interfacial quality .
Q. Methodological Guidance
Q. How should researchers design experiments to study WSi₂’s oxidation resistance under operational conditions?
- Controlled Atmosphere Testing : Expose samples to air or O₂ at 800–1,200°C and track mass gain/loss via thermogravimetric analysis (TGA).
- Surface Analysis : Use XPS to identify oxide phases (e.g., WO₃, SiO₂) and cross-sectional TEM to measure oxide layer thickness.
- Comparative Studies : Benchmark against MoSi₂, which forms self-passivating SiO₂ layers at high temperatures .
Q. What statistical approaches are suitable for analyzing variability in WSi₂ thin-film deposition data?
- Design of Experiments (DoE) : Use factorial designs to isolate effects of temperature, pressure, and gas ratios on film properties.
- Multivariate Regression : Corporate process parameters and characterization data (e.g., resistivity, thickness) to predict optimal conditions.
- Error Analysis : Calculate confidence intervals for repeated measurements to distinguish systematic vs. random errors .
Eigenschaften
CAS-Nummer |
12039-88-2 |
---|---|
Molekularformel |
Si2W |
Molekulargewicht |
240.01 g/mol |
InChI |
InChI=1S/2Si.W |
InChI-Schlüssel |
WQJQOUPTWCFRMM-UHFFFAOYSA-N |
SMILES |
[Si].[Si].[W] |
Kanonische SMILES |
[Si]#[W]#[Si] |
Key on ui other cas no. |
12039-88-2 |
Herkunft des Produkts |
United States |
Haftungsausschluss und Informationen zu In-Vitro-Forschungsprodukten
Bitte beachten Sie, dass alle Artikel und Produktinformationen, die auf BenchChem präsentiert werden, ausschließlich zu Informationszwecken bestimmt sind. Die auf BenchChem zum Kauf angebotenen Produkte sind speziell für In-vitro-Studien konzipiert, die außerhalb lebender Organismen durchgeführt werden. In-vitro-Studien, abgeleitet von dem lateinischen Begriff "in Glas", beinhalten Experimente, die in kontrollierten Laborumgebungen unter Verwendung von Zellen oder Geweben durchgeführt werden. Es ist wichtig zu beachten, dass diese Produkte nicht als Arzneimittel oder Medikamente eingestuft sind und keine Zulassung der FDA für die Vorbeugung, Behandlung oder Heilung von medizinischen Zuständen, Beschwerden oder Krankheiten erhalten haben. Wir müssen betonen, dass jede Form der körperlichen Einführung dieser Produkte in Menschen oder Tiere gesetzlich strikt untersagt ist. Es ist unerlässlich, sich an diese Richtlinien zu halten, um die Einhaltung rechtlicher und ethischer Standards in Forschung und Experiment zu gewährleisten.