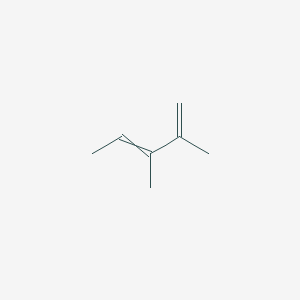
2,3-Dimethyl-1,3-pentadiene
Übersicht
Beschreibung
2,3-Dimethyl-1,3-pentadiene is a chemical compound with the molecular formula C7H12 . It has a molecular weight of 96.17 g/mol . The IUPAC name for this compound is (3E)-2,3-dimethylpenta-1,3-diene .
Molecular Structure Analysis
The molecular structure of this compound can be represented by the SMILES notationC/C=C(\C)/C(=C)C
. This compound has a total of 7 heavy atoms . Chemical Reactions Analysis
While specific reactions involving this compound are not detailed in the search results, it’s known that 1,3-dienes like this compound can undergo various reactions such as Diels-Alder reactions .Physical And Chemical Properties Analysis
This compound has a molecular weight of 96.17 g/mol . It has a complexity of 96.6 and a XLogP3-AA value of 3.3 . This compound does not have any hydrogen bond donors or acceptors .Wissenschaftliche Forschungsanwendungen
Polymerization and Material Science : 2,3-Dimethyl-1,3-pentadiene has been studied for its polymerization properties. Ricci et al. (2007) synthesized a highly stereoregular polymer using 3-methyl-1,3-pentadiene with a specific catalyst system. They characterized this polymer using various techniques and found it to have a syndiotactic E-1,2 structure. This research contributes to our understanding of diene polymerization mechanisms (Ricci et al., 2007).
Catalysis and Green Chemistry : Wu et al. (2009) developed a route to immobilize Pd nanoparticles for the hydrogenation of 2,4-dimethyl-1,3-pentadiene. They found that the selectivity of the reaction was significantly enhanced by a polymer coating, and the catalysts were very stable due to the cross-linked polymers. This study highlights the potential applications of these catalysts in green chemistry (Wu et al., 2009).
Chemical Reactions and Mechanisms : The reaction of this compound with other compounds has been studied to understand its chemical behavior. For instance, Podda et al. (1983) investigated the reaction of catechol with 2,4-dimethyl-2,3-pentadiene, finding the structure of the reaction product using various techniques. This study contributes to the knowledge of mass spectrometric synthesis (Podda et al., 1983).
Sorption and Polymerization Studies : Low (1977) studied the sorption of several alkenes, including 2,3-dimethyl-1,3-butadiene, by reactive silica. They found that these adsorbates formed chemisorbed species and polymers, suggesting the role of reactive silica as initiators for free-radical-initiated polymerizations (Low, 1977).
Safety and Hazards
Eigenschaften
IUPAC Name |
2,3-dimethylpenta-1,3-diene | |
---|---|---|
Source | PubChem | |
URL | https://pubchem.ncbi.nlm.nih.gov | |
Description | Data deposited in or computed by PubChem | |
InChI |
InChI=1S/C7H12/c1-5-7(4)6(2)3/h5H,2H2,1,3-4H3 | |
Source | PubChem | |
URL | https://pubchem.ncbi.nlm.nih.gov | |
Description | Data deposited in or computed by PubChem | |
InChI Key |
PCCCQOGUVCNYOI-UHFFFAOYSA-N | |
Source | PubChem | |
URL | https://pubchem.ncbi.nlm.nih.gov | |
Description | Data deposited in or computed by PubChem | |
Canonical SMILES |
CC=C(C)C(=C)C | |
Source | PubChem | |
URL | https://pubchem.ncbi.nlm.nih.gov | |
Description | Data deposited in or computed by PubChem | |
Molecular Formula |
C7H12 | |
Source | PubChem | |
URL | https://pubchem.ncbi.nlm.nih.gov | |
Description | Data deposited in or computed by PubChem | |
DSSTOX Substance ID |
DTXSID101015875 | |
Record name | 2,3-dimethyl-1,3-pentadiene | |
Source | EPA DSSTox | |
URL | https://comptox.epa.gov/dashboard/DTXSID101015875 | |
Description | DSSTox provides a high quality public chemistry resource for supporting improved predictive toxicology. | |
Molecular Weight |
96.17 g/mol | |
Source | PubChem | |
URL | https://pubchem.ncbi.nlm.nih.gov | |
Description | Data deposited in or computed by PubChem | |
CAS RN |
1113-56-0 | |
Record name | 2,3-Dimethyl-1,3-pentadiene | |
Source | CAS Common Chemistry | |
URL | https://commonchemistry.cas.org/detail?cas_rn=1113-56-0 | |
Description | CAS Common Chemistry is an open community resource for accessing chemical information. Nearly 500,000 chemical substances from CAS REGISTRY cover areas of community interest, including common and frequently regulated chemicals, and those relevant to high school and undergraduate chemistry classes. This chemical information, curated by our expert scientists, is provided in alignment with our mission as a division of the American Chemical Society. | |
Explanation | The data from CAS Common Chemistry is provided under a CC-BY-NC 4.0 license, unless otherwise stated. | |
Record name | 2,3-dimethyl-1,3-pentadiene | |
Source | EPA DSSTox | |
URL | https://comptox.epa.gov/dashboard/DTXSID101015875 | |
Description | DSSTox provides a high quality public chemistry resource for supporting improved predictive toxicology. | |
Retrosynthesis Analysis
AI-Powered Synthesis Planning: Our tool employs the Template_relevance Pistachio, Template_relevance Bkms_metabolic, Template_relevance Pistachio_ringbreaker, Template_relevance Reaxys, Template_relevance Reaxys_biocatalysis model, leveraging a vast database of chemical reactions to predict feasible synthetic routes.
One-Step Synthesis Focus: Specifically designed for one-step synthesis, it provides concise and direct routes for your target compounds, streamlining the synthesis process.
Accurate Predictions: Utilizing the extensive PISTACHIO, BKMS_METABOLIC, PISTACHIO_RINGBREAKER, REAXYS, REAXYS_BIOCATALYSIS database, our tool offers high-accuracy predictions, reflecting the latest in chemical research and data.
Strategy Settings
Precursor scoring | Relevance Heuristic |
---|---|
Min. plausibility | 0.01 |
Model | Template_relevance |
Template Set | Pistachio/Bkms_metabolic/Pistachio_ringbreaker/Reaxys/Reaxys_biocatalysis |
Top-N result to add to graph | 6 |
Feasible Synthetic Routes
Haftungsausschluss und Informationen zu In-Vitro-Forschungsprodukten
Bitte beachten Sie, dass alle Artikel und Produktinformationen, die auf BenchChem präsentiert werden, ausschließlich zu Informationszwecken bestimmt sind. Die auf BenchChem zum Kauf angebotenen Produkte sind speziell für In-vitro-Studien konzipiert, die außerhalb lebender Organismen durchgeführt werden. In-vitro-Studien, abgeleitet von dem lateinischen Begriff "in Glas", beinhalten Experimente, die in kontrollierten Laborumgebungen unter Verwendung von Zellen oder Geweben durchgeführt werden. Es ist wichtig zu beachten, dass diese Produkte nicht als Arzneimittel oder Medikamente eingestuft sind und keine Zulassung der FDA für die Vorbeugung, Behandlung oder Heilung von medizinischen Zuständen, Beschwerden oder Krankheiten erhalten haben. Wir müssen betonen, dass jede Form der körperlichen Einführung dieser Produkte in Menschen oder Tiere gesetzlich strikt untersagt ist. Es ist unerlässlich, sich an diese Richtlinien zu halten, um die Einhaltung rechtlicher und ethischer Standards in Forschung und Experiment zu gewährleisten.