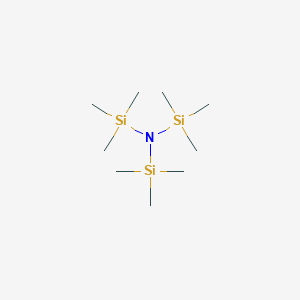
Tris(trimethylsilyl)amine
Übersicht
Beschreibung
Tris(trimethylsilyl)amine is the simplest tris(trialkylsilyl)amine, in which all three hydrogen atoms of the ammonia are replaced by trimethylsilyl groups . It has been of scientific interest as a stable intermediate in chemical nitrogen fixation .
Synthesis Analysis
Early attempts to prepare tris(trimethylsilyl)amine from ammonia and trimethylchlorosilane were unsuccessful even at temperatures of 500 °C and in the presence of the base pyridine . The reaction of ammonia and trimethylchlorosilane stops at the stage of the doubly silylated product bis(trimethylsilyl)amine . Tris(trimethylsilyl)amine is obtained by reaction of the sodium salt of hexamethyldisilazane with trimethylchlorosilane in 80% yield .Molecular Structure Analysis
The molecular formula of Tris(trimethylsilyl)amine is C9H27NSi3 . The IUPAC name is [bis(trimethylsilyl)amino]-dimethylsilyl]methane . The molecular weight is 233.57 g/mol .Physical And Chemical Properties Analysis
Tris(trimethylsilyl)amine is a colorless, crystalline or waxy solid which is stable to water and bases . It has a molecular weight of 233.57 g/mol . It has no hydrogen bond donor count and has a hydrogen bond acceptor count of 1 .Wissenschaftliche Forschungsanwendungen
Synthetic Building Block
Tris(trimethylsilyl)amine is used as a synthetic building block in various chemical reactions . For instance, it can form a nitridoantimone cubane-type cluster almost quantitatively at –60 °C when combined with antimony trichloride .
Intermediate in Chemical Nitrogen Fixation
Tris(trimethylsilyl)amine has been in the center of scientific interest as a stable intermediate in chemical nitrogen fixation . This process involves the conversion of atmospheric nitrogen (N2) into organic substrates under normal conditions .
Enantioselective Hydrosilylation
In the field of organic chemistry, Tris(trimethylsilyl)amine is used in enantioselective hydrosilylation . This process involves the addition of silicon-hydrogen bonds to unsaturated bonds, such as carbon-carbon double bonds, in a manner that creates a new chiral center.
Trifluoromethylation of Ketones
Tris(trimethylsilyl)amine can be used in the trifluoromethylation of ketones . This process involves the addition of a trifluoromethyl group (CF3) to a ketone, which can be useful in the synthesis of various pharmaceuticals and agrochemicals .
Production of Organo-Metalloid Compounds
Tris(trimethylsilyl)amine is used in the production of organo-metalloid compounds . These compounds have applications in various fields, including materials science, medicine, and electronics .
Stable to Water and Bases
Tris(trimethylsilyl)amine is a colorless, crystalline or waxy solid which is stable to water and bases . This property makes it useful in various chemical reactions where stability is required .
Safety and Hazards
Wirkmechanismus
- Tris(trimethylsilyl)amine has a straightforward structure: three trimethylsilyl (-Si(CH₃)₃) groups attached to a central nitrogen atom. It’s the simplest tris(trialkylsilyl)amine, where all three hydrogen atoms of ammonia are replaced by these silyl groups .
- One notable application involves its use in radical-based reactions. For instance, it generates silyl radicals when combined with electron donor-acceptor (EDA) complexes, driving alkylation processes . These radicals can add to double bonds, leading to diverse products.
- In synthetic chemistry, it plays a role in constructing complex molecules. For example, from antimony trichloride and tris(trimethylsilyl)amine, a nitridoantimone cubane-type cluster can be formed .
- Distribution : It remains stable in the presence of water and bases but can be cleaved by alcohols or acids, releasing ammonia .
- Environmental factors, such as solvent polarity and temperature, influence its reactivity. For example, it reacts with trimethylchlorosilane to form tris(trimethylsilyl)amine .
Target of Action
Mode of Action
Biochemical Pathways
Pharmacokinetics (ADME)
Action Environment
Eigenschaften
IUPAC Name |
[[bis(trimethylsilyl)amino]-dimethylsilyl]methane | |
---|---|---|
Source | PubChem | |
URL | https://pubchem.ncbi.nlm.nih.gov | |
Description | Data deposited in or computed by PubChem | |
InChI |
InChI=1S/C9H27NSi3/c1-11(2,3)10(12(4,5)6)13(7,8)9/h1-9H3 | |
Source | PubChem | |
URL | https://pubchem.ncbi.nlm.nih.gov | |
Description | Data deposited in or computed by PubChem | |
InChI Key |
PEGHITPVRNZWSI-UHFFFAOYSA-N | |
Source | PubChem | |
URL | https://pubchem.ncbi.nlm.nih.gov | |
Description | Data deposited in or computed by PubChem | |
Canonical SMILES |
C[Si](C)(C)N([Si](C)(C)C)[Si](C)(C)C | |
Source | PubChem | |
URL | https://pubchem.ncbi.nlm.nih.gov | |
Description | Data deposited in or computed by PubChem | |
Molecular Formula |
C9H27NSi3 | |
Source | PubChem | |
URL | https://pubchem.ncbi.nlm.nih.gov | |
Description | Data deposited in or computed by PubChem | |
DSSTOX Substance ID |
DTXSID2061802 | |
Record name | Silanamine, 1,1,1-trimethyl-N,N-bis(trimethylsilyl)- | |
Source | EPA DSSTox | |
URL | https://comptox.epa.gov/dashboard/DTXSID2061802 | |
Description | DSSTox provides a high quality public chemistry resource for supporting improved predictive toxicology. | |
Molecular Weight |
233.57 g/mol | |
Source | PubChem | |
URL | https://pubchem.ncbi.nlm.nih.gov | |
Description | Data deposited in or computed by PubChem | |
Product Name |
Tris(trimethylsilyl)amine | |
CAS RN |
1586-73-8 | |
Record name | 1,1,1-Trimethyl-N,N-bis(trimethylsilyl)silanamine | |
Source | CAS Common Chemistry | |
URL | https://commonchemistry.cas.org/detail?cas_rn=1586-73-8 | |
Description | CAS Common Chemistry is an open community resource for accessing chemical information. Nearly 500,000 chemical substances from CAS REGISTRY cover areas of community interest, including common and frequently regulated chemicals, and those relevant to high school and undergraduate chemistry classes. This chemical information, curated by our expert scientists, is provided in alignment with our mission as a division of the American Chemical Society. | |
Explanation | The data from CAS Common Chemistry is provided under a CC-BY-NC 4.0 license, unless otherwise stated. | |
Record name | Silanamine, 1,1,1-trimethyl-N,N-bis(trimethylsilyl)- | |
Source | ChemIDplus | |
URL | https://pubchem.ncbi.nlm.nih.gov/substance/?source=chemidplus&sourceid=0001586738 | |
Description | ChemIDplus is a free, web search system that provides access to the structure and nomenclature authority files used for the identification of chemical substances cited in National Library of Medicine (NLM) databases, including the TOXNET system. | |
Record name | Tris(trimethylsilyl)amine | |
Source | DTP/NCI | |
URL | https://dtp.cancer.gov/dtpstandard/servlet/dwindex?searchtype=NSC&outputformat=html&searchlist=252162 | |
Description | The NCI Development Therapeutics Program (DTP) provides services and resources to the academic and private-sector research communities worldwide to facilitate the discovery and development of new cancer therapeutic agents. | |
Explanation | Unless otherwise indicated, all text within NCI products is free of copyright and may be reused without our permission. Credit the National Cancer Institute as the source. | |
Record name | Silanamine, 1,1,1-trimethyl-N,N-bis(trimethylsilyl)- | |
Source | EPA Chemicals under the TSCA | |
URL | https://www.epa.gov/chemicals-under-tsca | |
Description | EPA Chemicals under the Toxic Substances Control Act (TSCA) collection contains information on chemicals and their regulations under TSCA, including non-confidential content from the TSCA Chemical Substance Inventory and Chemical Data Reporting. | |
Record name | Silanamine, 1,1,1-trimethyl-N,N-bis(trimethylsilyl)- | |
Source | EPA DSSTox | |
URL | https://comptox.epa.gov/dashboard/DTXSID2061802 | |
Description | DSSTox provides a high quality public chemistry resource for supporting improved predictive toxicology. | |
Record name | 1,1,1-trimethyl-N,N-bis(trimethylsilyl)silylamine | |
Source | European Chemicals Agency (ECHA) | |
URL | https://echa.europa.eu/substance-information/-/substanceinfo/100.014.951 | |
Description | The European Chemicals Agency (ECHA) is an agency of the European Union which is the driving force among regulatory authorities in implementing the EU's groundbreaking chemicals legislation for the benefit of human health and the environment as well as for innovation and competitiveness. | |
Explanation | Use of the information, documents and data from the ECHA website is subject to the terms and conditions of this Legal Notice, and subject to other binding limitations provided for under applicable law, the information, documents and data made available on the ECHA website may be reproduced, distributed and/or used, totally or in part, for non-commercial purposes provided that ECHA is acknowledged as the source: "Source: European Chemicals Agency, http://echa.europa.eu/". Such acknowledgement must be included in each copy of the material. ECHA permits and encourages organisations and individuals to create links to the ECHA website under the following cumulative conditions: Links can only be made to webpages that provide a link to the Legal Notice page. | |
Record name | NONAMETHYLTRISILAZANE | |
Source | FDA Global Substance Registration System (GSRS) | |
URL | https://gsrs.ncats.nih.gov/ginas/app/beta/substances/SVA5FGS9US | |
Description | The FDA Global Substance Registration System (GSRS) enables the efficient and accurate exchange of information on what substances are in regulated products. Instead of relying on names, which vary across regulatory domains, countries, and regions, the GSRS knowledge base makes it possible for substances to be defined by standardized, scientific descriptions. | |
Explanation | Unless otherwise noted, the contents of the FDA website (www.fda.gov), both text and graphics, are not copyrighted. They are in the public domain and may be republished, reprinted and otherwise used freely by anyone without the need to obtain permission from FDA. Credit to the U.S. Food and Drug Administration as the source is appreciated but not required. | |
Retrosynthesis Analysis
AI-Powered Synthesis Planning: Our tool employs the Template_relevance Pistachio, Template_relevance Bkms_metabolic, Template_relevance Pistachio_ringbreaker, Template_relevance Reaxys, Template_relevance Reaxys_biocatalysis model, leveraging a vast database of chemical reactions to predict feasible synthetic routes.
One-Step Synthesis Focus: Specifically designed for one-step synthesis, it provides concise and direct routes for your target compounds, streamlining the synthesis process.
Accurate Predictions: Utilizing the extensive PISTACHIO, BKMS_METABOLIC, PISTACHIO_RINGBREAKER, REAXYS, REAXYS_BIOCATALYSIS database, our tool offers high-accuracy predictions, reflecting the latest in chemical research and data.
Strategy Settings
Precursor scoring | Relevance Heuristic |
---|---|
Min. plausibility | 0.01 |
Model | Template_relevance |
Template Set | Pistachio/Bkms_metabolic/Pistachio_ringbreaker/Reaxys/Reaxys_biocatalysis |
Top-N result to add to graph | 6 |
Feasible Synthetic Routes
Q & A
Q1: What is the molecular formula and weight of Tris(trimethylsilyl)amine?
A1: Tris(trimethylsilyl)amine has the molecular formula C9H27NSi3 and a molecular weight of 249.60 g/mol.
Q2: What spectroscopic techniques have been used to characterize Tris(trimethylsilyl)amine?
A2: Researchers have utilized various spectroscopic methods to elucidate the structure of Tris(trimethylsilyl)amine. Key techniques include:
- Nuclear Magnetic Resonance (NMR) Spectroscopy: Both proton and deuterium NMR have provided insights into the dynamic behavior of the molecule in solid state. [] This includes understanding the rotation of methyl and trimethylsilyl groups, as well as the overall molecular motion. []
- Infrared (IR) Spectroscopy: IR spectroscopy has been instrumental in identifying characteristic vibrational frequencies associated with specific bonds within the molecule, such as N-Si and N-H bonds. [, , ]
- Electron Diffraction: Electron diffraction studies in the gas phase have been crucial in determining the planar structure of the Si-N bonds in the molecule. []
- Photoelectron Spectroscopy: Photoelectron spectroscopy has been employed to investigate the electronic structure and bonding properties of Tris(trimethylsilyl)amine and related silylamines. []
Q3: What is the significance of Tris(trimethylsilyl)amine in dinitrogen fixation research?
A3: Tris(trimethylsilyl)amine serves as a key product in the catalytic conversion of dinitrogen (N2) to more useful nitrogen-containing compounds. Several transition metal complexes, including those of titanium [], rhodium [], cobalt [, , , ], and rhenium [], have demonstrated the ability to catalyze this transformation, with Tris(trimethylsilyl)amine as a quantifiable product. [, , , , , , ]
Q4: How does the choice of transition metal influence the catalytic silylation of dinitrogen using Tris(trimethylsilyl)amine?
A4: Research indicates that the identity of the transition metal significantly impacts the catalytic efficiency for dinitrogen silylation. For instance, cobalt complexes generally outperform iron counterparts in terms of turnover number, highlighting the influence of the metal center on reaction outcomes. [, , , ]
Q5: Beyond dinitrogen fixation, what other synthetic applications utilize Tris(trimethylsilyl)amine?
A5: Tris(trimethylsilyl)amine exhibits versatility in organic synthesis:
- Amide Base Precursor: It acts as a precursor for generating highly reactive amide bases in situ. When combined with fluoride sources like tetramethylammonium fluoride (TMAF) or cesium fluoride (CsF), it generates amide bases capable of deprotonating heteroarenes [, ] and facilitating various coupling reactions. [, ]
- Borane Chemistry: Tris(trimethylsilyl)amine reacts with monohaloboranes, leading to the formation of (trimethylsilyl)aminoboranes. [, ]
- Selenide Synthesis: It is employed in the preparation of selenenyl azides and triselenenamides by reacting with selenenyl chlorides. []
Q6: Are there any examples where Tris(trimethylsilyl)amine participates in the formation of unusual chemical bonds?
A6: Yes, Tris(trimethylsilyl)amine has been used in the synthesis of tellurium nitride chloride, demonstrating its utility in accessing compounds with unique Te-N bonds. []
Q7: Has there been research exploring the effects of structural modifications on the reactivity of Tris(trimethylsilyl)amine?
A7: While Tris(trimethylsilyl)amine is the most widely studied compound in its class, researchers have investigated the properties of related silylamines. For instance, comparisons have been drawn between hexamethyldisiloxane and Tris(trimethylsilyl)amine, examining the impact of structural variations on their respective properties. [] This exploration provides valuable insights into structure-activity relationships within this class of compounds.
Haftungsausschluss und Informationen zu In-Vitro-Forschungsprodukten
Bitte beachten Sie, dass alle Artikel und Produktinformationen, die auf BenchChem präsentiert werden, ausschließlich zu Informationszwecken bestimmt sind. Die auf BenchChem zum Kauf angebotenen Produkte sind speziell für In-vitro-Studien konzipiert, die außerhalb lebender Organismen durchgeführt werden. In-vitro-Studien, abgeleitet von dem lateinischen Begriff "in Glas", beinhalten Experimente, die in kontrollierten Laborumgebungen unter Verwendung von Zellen oder Geweben durchgeführt werden. Es ist wichtig zu beachten, dass diese Produkte nicht als Arzneimittel oder Medikamente eingestuft sind und keine Zulassung der FDA für die Vorbeugung, Behandlung oder Heilung von medizinischen Zuständen, Beschwerden oder Krankheiten erhalten haben. Wir müssen betonen, dass jede Form der körperlichen Einführung dieser Produkte in Menschen oder Tiere gesetzlich strikt untersagt ist. Es ist unerlässlich, sich an diese Richtlinien zu halten, um die Einhaltung rechtlicher und ethischer Standards in Forschung und Experiment zu gewährleisten.