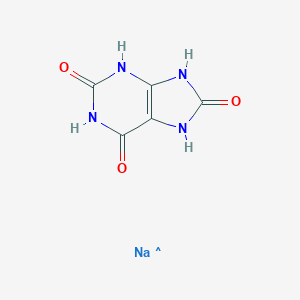
Natriumsalz der Harnsäure
Übersicht
Beschreibung
Uric acid sodium salt is a chemical compound with significant importance in various scientific fields. It is known for its unique structure and properties, which make it valuable in research and industrial applications.
Wissenschaftliche Forschungsanwendungen
Uric acid sodium salt has numerous applications in scientific research:
Chemistry: It is used as a precursor in the synthesis of various purine derivatives and as a reagent in organic synthesis.
Biology: It plays a role in studying enzyme mechanisms and metabolic pathways involving purine compounds.
Medicine: It is investigated for its potential therapeutic effects and as a model compound in drug development.
Industry: It is used in the production of pharmaceuticals, agrochemicals, and other fine chemicals.
Wirkmechanismus
Target of Action
Uric acid sodium salt, also known as 1H-Purine-2,6,8(3H)-trione, 7,9-dihydro-, sodium salt, NCGC00181049-01, or CID 16220071, is the sodium salt of uric acid . It is primarily targeted at the synovial fluid in the body, particularly in conditions such as gout .
Mode of Action
Uric acid sodium salt interacts with its targets by precipitating calcium oxalate from urine . It also reverses the inhibitory effects of reactive oxygen and nitrogen metabolites on gelatinase A activity . This interaction leads to changes in the metabolic processes within the body.
Biochemical Pathways
The biochemical pathways affected by uric acid sodium salt involve the metabolic breakdown of purine nucleotides . The enzyme xanthine oxidase (XO) catalyzes the formation of uric acid from xanthine and hypoxanthine . Uric acid sodium salt can influence these pathways, leading to changes in uric acid levels in the body.
Pharmacokinetics
The pharmacokinetics of uric acid sodium salt involve its absorption, distribution, metabolism, and excretion (ADME). It is known that uric acid, the parent compound of uric acid sodium salt, is a product of the metabolic breakdown of purine nucleotides and is a normal component of urine . This suggests that uric acid sodium salt may be excreted through urine.
Result of Action
The action of uric acid sodium salt results in molecular and cellular effects such as the precipitation of calcium oxalate from urine . It also reverses the inhibitory effects of reactive oxygen and nitrogen metabolites on gelatinase A activity . Excess sodium urate in soft tissues results in a condition referred to as tophi .
Action Environment
The action, efficacy, and stability of uric acid sodium salt can be influenced by various environmental factors. For instance, in gout, uric acid sodium salt is observed in synovial fluid . Therefore, the physiological environment, such as the presence of synovial fluid, can influence the action of uric acid sodium salt. Additionally, lifestyle factors like diet and physical activity can also impact the levels of uric acid in the body .
Biochemische Analyse
Biochemical Properties
Uric acid sodium salt is involved in several biochemical reactions. It has been found to precipitate calcium oxalate from urine . This property of uric acid sodium salt has been linked to the formation of calcium oxalate stones, a common type of kidney stone .
Cellular Effects
Uric acid sodium salt has significant effects on various types of cells and cellular processes. In the context of gout, a form of inflammatory arthritis, uric acid sodium salt crystals have been observed in synovial fluid . These crystals are known to induce inflammation, leading to the painful symptoms associated with gout .
Molecular Mechanism
The molecular mechanism of action of uric acid sodium salt involves its interaction with various biomolecules. It has been shown to bind iron(III), a process that has been studied for its potential role in inflammation . Additionally, uric acid sodium salt has been shown to reverse the inhibitory effects of reactive oxygen and nitrogen metabolites on gelatinase A activity .
Metabolic Pathways
Uric acid sodium salt is involved in the metabolic pathway related to the formation of calcium oxalate stones. It precipitates calcium oxalate from urine, a process that is a key step in the formation of these stones .
Transport and Distribution
Given its solubility in water and its role in various biochemical reactions, it is likely that it can be transported and distributed within cells and tissues via standard biochemical transport mechanisms .
Subcellular Localization
Given its biochemical properties and its role in various cellular processes, it is likely that it can be found in various subcellular compartments where it exerts its effects .
Vorbereitungsmethoden
Synthetic Routes and Reaction Conditions
The synthesis of Uric acid sodium salt typically involves the reaction of purine derivatives with sodium hydroxide. The reaction conditions often include controlled temperatures and pH levels to ensure the desired product is obtained with high purity and yield.
Industrial Production Methods
Industrial production of this compound may involve large-scale synthesis using automated reactors and continuous flow systems. These methods ensure consistent quality and efficiency in production, meeting the demands of various industries.
Analyse Chemischer Reaktionen
Types of Reactions
Uric acid sodium salt undergoes several types of chemical reactions, including:
Oxidation: This reaction involves the addition of oxygen or the removal of hydrogen, leading to the formation of oxidized products.
Reduction: This reaction involves the addition of hydrogen or the removal of oxygen, resulting in reduced products.
Substitution: This reaction involves the replacement of one atom or group of atoms with another, leading to the formation of substituted products.
Common Reagents and Conditions
Common reagents used in these reactions include oxidizing agents like potassium permanganate, reducing agents like sodium borohydride, and various nucleophiles for substitution reactions. The conditions for these reactions vary but often include specific temperatures, solvents, and catalysts to achieve the desired outcomes.
Major Products Formed
The major products formed from these reactions depend on the specific reagents and conditions used. For example, oxidation may yield purine-2,6,8-trione derivatives, while reduction may produce dihydropurine derivatives.
Vergleich Mit ähnlichen Verbindungen
Similar Compounds
1H-Purine-2,6,8(3H)-trione: A closely related compound with similar structure and properties.
7,9-Dihydro-1H-purine-6,8-dione: Another purine derivative with comparable chemical behavior.
3,7-Dihydro-1,3,7-trimethyl-1H-purine-2,6-dione:
Uniqueness
Uric acid sodium salt is unique due to its specific sodium salt form, which imparts different solubility and reactivity characteristics compared to its non-sodium counterparts. This uniqueness makes it valuable in specific applications where these properties are advantageous.
Biologische Aktivität
Uric acid sodium salt, also known as sodium urate, is the sodium salt of uric acid and plays a significant role in various biological processes. This compound is particularly known for its involvement in gout, a form of inflammatory arthritis caused by the accumulation of monosodium urate crystals in joints. This article explores the biological activity of uric acid sodium salt, highlighting its biochemical properties, physiological effects, and implications in health and disease.
Uric acid sodium salt has the chemical formula and is characterized by its solubility in water. It is formed when uric acid reacts with sodium ions, resulting in a compound that can precipitate under certain physiological conditions.
Biochemical Actions
- Precipitation and Crystal Formation : Sodium urate can precipitate from body fluids, particularly synovial fluid in joints, leading to gouty arthritis. The presence of these crystals triggers an inflammatory response characterized by pain and swelling .
- Reactive Oxygen Species (ROS) Modulation : Sodium urate has been shown to reverse the inhibitory effects of reactive oxygen and nitrogen metabolites on gelatinase A activity, suggesting a role in modulating oxidative stress .
- Calcium Oxalate Interaction : In vitro studies indicate that sodium urate can precipitate calcium oxalate from urine, which has implications for kidney stone formation .
Physiological Effects
The physiological effects of uric acid sodium salt are multifaceted:
- Inflammation : Elevated levels of sodium urate are associated with increased inflammation. In animal models, intra-articular administration of sodium urate has been used to induce synovitis, mimicking gout pathology .
- Antioxidant Properties : Uric acid acts as an antioxidant; however, its role is complex as elevated levels can lead to pro-inflammatory states .
- Impact on Blood Pressure : Research indicates a correlation between high dietary sodium intake and serum uric acid levels. Specifically, studies show that increased sodium intake can lead to reduced serum uric acid concentrations in the short term but may have long-term implications for hypertension .
Case Study 1: Gout and Inflammation
A study investigated the effects of sodium urate on inflammation in a rat model subjected to hemorrhagic shock. The results indicated that uric acid released from dying cells could act as a mediator of organ inflammation and apoptosis post-shock, emphasizing its pro-inflammatory potential .
Case Study 2: Dietary Sodium Impact
A clinical trial assessed the relationship between dietary sodium intake and serum uric acid levels among 90 subjects. The findings revealed that transitioning from a low-salt diet (3 g/day) to a high-salt diet (18 g/day) resulted in significant decreases in plasma uric acid levels, demonstrating the influence of dietary factors on uric acid metabolism .
Data Table
Eigenschaften
IUPAC Name |
sodium;2,6-dioxo-3,7-dihydropurin-8-olate | |
---|---|---|
Details | Computed by Lexichem TK 2.7.0 (PubChem release 2021.05.07) | |
Source | PubChem | |
URL | https://pubchem.ncbi.nlm.nih.gov | |
Description | Data deposited in or computed by PubChem | |
InChI |
InChI=1S/C5H4N4O3.Na/c10-3-1-2(7-4(11)6-1)8-5(12)9-3;/h(H4,6,7,8,9,10,11,12);/q;+1/p-1 | |
Details | Computed by InChI 1.0.6 (PubChem release 2021.05.07) | |
Source | PubChem | |
URL | https://pubchem.ncbi.nlm.nih.gov | |
Description | Data deposited in or computed by PubChem | |
InChI Key |
NAFSTSRULRIERK-UHFFFAOYSA-M | |
Details | Computed by InChI 1.0.6 (PubChem release 2021.05.07) | |
Source | PubChem | |
URL | https://pubchem.ncbi.nlm.nih.gov | |
Description | Data deposited in or computed by PubChem | |
Canonical SMILES |
C12=C(NC(=O)NC1=O)N=C(N2)[O-].[Na+] | |
Details | Computed by OEChem 2.3.0 (PubChem release 2021.05.07) | |
Source | PubChem | |
URL | https://pubchem.ncbi.nlm.nih.gov | |
Description | Data deposited in or computed by PubChem | |
Molecular Formula |
C5H3N4NaO3 | |
Details | Computed by PubChem 2.1 (PubChem release 2021.05.07) | |
Source | PubChem | |
URL | https://pubchem.ncbi.nlm.nih.gov | |
Description | Data deposited in or computed by PubChem | |
Molecular Weight |
190.09 g/mol | |
Details | Computed by PubChem 2.1 (PubChem release 2021.05.07) | |
Source | PubChem | |
URL | https://pubchem.ncbi.nlm.nih.gov | |
Description | Data deposited in or computed by PubChem | |
CAS No. |
1198-77-2 | |
Record name | 1H-Purine-2,6,8(3H)-trione, 7,9-dihydro-, sodium salt (1:1) | |
Source | EPA Chemicals under the TSCA | |
URL | https://www.epa.gov/chemicals-under-tsca | |
Description | EPA Chemicals under the Toxic Substances Control Act (TSCA) collection contains information on chemicals and their regulations under TSCA, including non-confidential content from the TSCA Chemical Substance Inventory and Chemical Data Reporting. | |
Record name | 7,9-dihydro-1H-purine-2,6,8(3H)-trione, monosodium salt | |
Source | European Chemicals Agency (ECHA) | |
URL | https://echa.europa.eu/substance-information/-/substanceinfo/100.013.489 | |
Description | The European Chemicals Agency (ECHA) is an agency of the European Union which is the driving force among regulatory authorities in implementing the EU's groundbreaking chemicals legislation for the benefit of human health and the environment as well as for innovation and competitiveness. | |
Explanation | Use of the information, documents and data from the ECHA website is subject to the terms and conditions of this Legal Notice, and subject to other binding limitations provided for under applicable law, the information, documents and data made available on the ECHA website may be reproduced, distributed and/or used, totally or in part, for non-commercial purposes provided that ECHA is acknowledged as the source: "Source: European Chemicals Agency, http://echa.europa.eu/". Such acknowledgement must be included in each copy of the material. ECHA permits and encourages organisations and individuals to create links to the ECHA website under the following cumulative conditions: Links can only be made to webpages that provide a link to the Legal Notice page. | |
Retrosynthesis Analysis
AI-Powered Synthesis Planning: Our tool employs the Template_relevance Pistachio, Template_relevance Bkms_metabolic, Template_relevance Pistachio_ringbreaker, Template_relevance Reaxys, Template_relevance Reaxys_biocatalysis model, leveraging a vast database of chemical reactions to predict feasible synthetic routes.
One-Step Synthesis Focus: Specifically designed for one-step synthesis, it provides concise and direct routes for your target compounds, streamlining the synthesis process.
Accurate Predictions: Utilizing the extensive PISTACHIO, BKMS_METABOLIC, PISTACHIO_RINGBREAKER, REAXYS, REAXYS_BIOCATALYSIS database, our tool offers high-accuracy predictions, reflecting the latest in chemical research and data.
Strategy Settings
Precursor scoring | Relevance Heuristic |
---|---|
Min. plausibility | 0.01 |
Model | Template_relevance |
Template Set | Pistachio/Bkms_metabolic/Pistachio_ringbreaker/Reaxys/Reaxys_biocatalysis |
Top-N result to add to graph | 6 |
Feasible Synthetic Routes
Q1: How does uric acid sodium salt influence the immune response?
A: Research suggests uric acid sodium salt can exert differential effects on humoral and cellular immune responses. In a study using BALB/c mice, uric acid sodium salt as an adjuvant did not enhance antibody response to trichosanthin (TCS) antigen. In fact, it significantly reduced the response compared to TCS alone []. Conversely, it enhanced delayed-type hypersensitivity (DTH) and lymphocyte proliferation, indicating a stimulatory effect on cellular immunity [].
Q2: What are the potential therapeutic applications of uric acid sodium salt in gouty arthritis?
A: While uric acid sodium salt injection is a common method for inducing gouty arthritis in animal models [, , ], research suggests potential therapeutic avenues for managing gouty arthritis. A study investigating pricking bloodletting therapy in rats with gouty arthritis found that treatment led to increased interleukin-10 (IL-10) expression in the ankle joint []. This increase correlated with a reduction in joint swelling, suggesting a possible anti-inflammatory role of IL-10 mediated by the therapy []. Additionally, aqueous extracts of Sigesbeckia orientalis have shown promise in reducing inflammation in gouty arthritis models, potentially by inhibiting the JNK signaling pathway [].
Q3: Does uric acid sodium salt play a role in periodontal inflammation?
A: Emerging research suggests a potential link between uric acid sodium salt and periodontal inflammation. One study found that gingipains, proteases produced by the bacterium Porphyromonas gingivalis (a key player in periodontitis), can increase uric acid production in THP-1 macrophages []. This increase is mediated by upregulation and activation of xanthine oxidoreductase, the enzyme responsible for uric acid production []. Furthermore, uric acid sodium salt was shown to induce inflammation in human keratinocytes, suggesting its potential role as a mediator of inflammation in periodontal tissues [].
Q4: Can dietary interventions impact uric acid metabolism in broilers?
A: Studies in broilers provide insights into uric acid metabolism manipulation. Supplementation with allopurinol, a xanthine oxidase inhibitor, significantly reduced uric acid concentrations in the liver of broilers [, ]. Conversely, inosine supplementation increased total xanthine oxidoreductase activity in the liver [], highlighting the interplay between dietary components and uric acid metabolism.
Haftungsausschluss und Informationen zu In-Vitro-Forschungsprodukten
Bitte beachten Sie, dass alle Artikel und Produktinformationen, die auf BenchChem präsentiert werden, ausschließlich zu Informationszwecken bestimmt sind. Die auf BenchChem zum Kauf angebotenen Produkte sind speziell für In-vitro-Studien konzipiert, die außerhalb lebender Organismen durchgeführt werden. In-vitro-Studien, abgeleitet von dem lateinischen Begriff "in Glas", beinhalten Experimente, die in kontrollierten Laborumgebungen unter Verwendung von Zellen oder Geweben durchgeführt werden. Es ist wichtig zu beachten, dass diese Produkte nicht als Arzneimittel oder Medikamente eingestuft sind und keine Zulassung der FDA für die Vorbeugung, Behandlung oder Heilung von medizinischen Zuständen, Beschwerden oder Krankheiten erhalten haben. Wir müssen betonen, dass jede Form der körperlichen Einführung dieser Produkte in Menschen oder Tiere gesetzlich strikt untersagt ist. Es ist unerlässlich, sich an diese Richtlinien zu halten, um die Einhaltung rechtlicher und ethischer Standards in Forschung und Experiment zu gewährleisten.