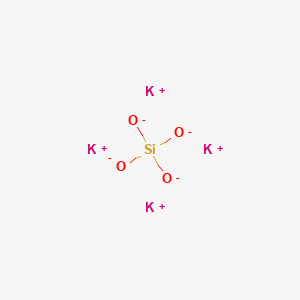
Kaliumorthosilikat
Übersicht
Beschreibung
Potassium orthosilicate is an inorganic compound with the chemical formula K₄SiO₄. It is a member of the silicate family, which includes various salts and esters of orthosilicic acid. Potassium orthosilicate is known for its stability and is commonly used in various industrial applications due to its unique chemical properties.
Wissenschaftliche Forschungsanwendungen
Chemistry: Potassium orthosilicate is used as a precursor in the synthesis of other silicate compounds. It is also employed in the preparation of silica nanoparticles and as a reagent in various chemical reactions.
Biology and Medicine: In biological research, potassium orthosilicate is studied for its potential role in bone health and collagen synthesis. It is also investigated for its effects on skin, hair, and nails.
Industry: Potassium orthosilicate is widely used in the construction industry as a binder for cement and other construction materials. It is also used in the production of mineral paints and coatings, as well as in industrial cleaning agents due to its alkaline properties.
Wirkmechanismus
Target of Action
Potassium orthosilicate, also known as ortho-silicic acid (H4SiO4), is a major form of bioavailable silicon for both humans and animals . It primarily targets the connective tissues in the body, playing a crucial role in bone mineralization, collagen synthesis, skin, hair, and nails health . It has also been associated with atherosclerosis, Alzheimer’s disease, immune system enhancement, and other disorders or pharmacological effects .
Mode of Action
Potassium orthosilicate interacts with its targets by releasing ortho-silicic acid, a bioavailable form of silicon . This compound exhibits profound adsorption and absorption properties, which might be attributed to specific structural characteristics . The interaction of ortho-silicic acid with its targets leads to various changes, including enhanced bone mineralization and collagen synthesis .
Biochemical Pathways
The exact biochemical pathways affected by potassium orthosilicate remain largely unknown. It is known that silicon plays a role in various physiological processes, including photosynthetic co2 fixation and the utilization of photosynthates . Potassium orthosilicate might influence these processes by modulating the availability and utilization of silicon.
Pharmacokinetics
The pharmacokinetics of potassium orthosilicate involve its release of ortho-silicic acid, which is a bioavailable form of silicon . Despite the substantial water insolubility of potassium orthosilicate, it releases a small but significant equilibrium concentration of ortho-silicic acid in contact with water and physiological fluids . This property contributes to the compound’s bioavailability.
Result of Action
The action of potassium orthosilicate results in various molecular and cellular effects. These include enhanced bone mineralization, improved collagen synthesis, and better health of skin, hair, and nails . Additionally, it has been associated with the enhancement of the immune system and potential therapeutic effects for conditions like atherosclerosis and Alzheimer’s disease .
Action Environment
The action, efficacy, and stability of potassium orthosilicate can be influenced by various environmental factors. For instance, the presence of water and physiological fluids is crucial for the release of ortho-silicic acid . Moreover, the compound’s action might be affected by the pH of the environment
Biochemische Analyse
Biochemical Properties
Potassium orthosilicate plays a significant role in biochemical reactions. It has been associated with bone mineralization, collagen synthesis, skin, hair, and nails health, atherosclerosis, Alzheimer disease, immune system enhancement, and other disorders or pharmacological effects . It interacts with various enzymes, proteins, and other biomolecules, influencing their function and activity .
Cellular Effects
Potassium orthosilicate has profound effects on various types of cells and cellular processes. It influences cell function by impacting cell signaling pathways, gene expression, and cellular metabolism . For instance, it has been associated with the biosynthesis of collagen and glycosaminoglycan, which are needed for bone formation .
Molecular Mechanism
At the molecular level, potassium orthosilicate exerts its effects through binding interactions with biomolecules, enzyme inhibition or activation, and changes in gene expression
Dosage Effects in Animal Models
The effects of potassium orthosilicate can vary with different dosages in animal models. Studies have shown that it can have beneficial effects at certain dosages, but may also have toxic or adverse effects at high doses .
Metabolic Pathways
Potassium orthosilicate is involved in various metabolic pathways. It interacts with enzymes and cofactors, and can influence metabolic flux or metabolite levels
Transport and Distribution
Potassium orthosilicate is transported and distributed within cells and tissues in ways that are still being studied. It is known to interact with certain transporters or binding proteins, and can influence its localization or accumulation .
Subcellular Localization
The subcellular localization of potassium orthosilicate and its effects on activity or function are areas of active research. It is believed that it may be directed to specific compartments or organelles through targeting signals or post-translational modifications .
Vorbereitungsmethoden
Synthetic Routes and Reaction Conditions: Potassium orthosilicate can be synthesized by reacting potassium hydroxide with silica. The reaction typically occurs at high temperatures, around 1300°C, to ensure complete conversion. The general reaction is as follows: [ \text{SiO}_2 + 2\text{KOH} \rightarrow \text{K}_2\text{SiO}_3 + \text{H}_2\text{O} ]
Industrial Production Methods: In industrial settings, potassium orthosilicate is produced by melting potassium carbonate with silica sand. This process involves heating the mixture to high temperatures, resulting in the formation of potassium orthosilicate and carbon dioxide: [ \text{K}_2\text{CO}_3 + \text{SiO}_2 \rightarrow \text{K}_2\text{SiO}_3 + \text{CO}_2 ]
Analyse Chemischer Reaktionen
Types of Reactions: Potassium orthosilicate undergoes various chemical reactions, including:
Oxidation: Potassium orthosilicate can be oxidized to form potassium silicate.
Reduction: It can be reduced under specific conditions to yield different silicate compounds.
Substitution: Potassium orthosilicate can participate in substitution reactions where other cations replace potassium ions.
Common Reagents and Conditions:
Acids: When treated with acids, potassium orthosilicate decomposes to form silica and the corresponding potassium salts.
Alkaline Conditions: Under alkaline conditions, it remains stable and can form complex silicate structures.
Major Products:
Silica: One of the primary products formed from the decomposition of potassium orthosilicate.
Potassium Salts: Various potassium salts are formed depending on the reaction conditions and reagents used.
Vergleich Mit ähnlichen Verbindungen
Sodium Orthosilicate: Similar in structure and properties but contains sodium instead of potassium.
Tetraethyl Orthosilicate: Used in organic synthesis and the manufacture of aerogels.
Tetramethyl Orthosilicate: An alternative to tetraethyl orthosilicate with similar applications but produces toxic methanol upon decomposition.
Uniqueness: Potassium orthosilicate is unique due to its specific applications in the construction industry and its role in biological research. Its stability under alkaline conditions and ability to form complex silicate structures make it a valuable compound in various fields.
Biologische Aktivität
Potassium orthosilicate (K4O4Si) is a compound that has garnered attention for its potential biological activities, particularly in agricultural and medicinal applications. This article explores its biological activity, including its effects on plant growth, stress tolerance, and potential therapeutic uses.
Potassium orthosilicate is characterized by its silicate structure, which allows it to interact with various biological systems. It is soluble in water and can provide both potassium and silicate ions, essential for plant growth and development.
Effects on Plant Growth
1. Enhancing Growth Parameters:
Research indicates that potassium orthosilicate positively influences various growth parameters in plants. For instance, it has been shown to:
- Promote root length and overall vegetative growth.
- Enhance chlorophyll production, which is crucial for photosynthesis.
- Improve nutrient uptake (nitrogen, phosphorus, potassium) and translocation to sink organs, ultimately increasing yield attributes such as pod number and seed weight .
2. Stress Tolerance:
Potassium orthosilicate plays a significant role in enhancing plants' resilience to abiotic stresses, particularly salinity. Studies have demonstrated that:
- Its application reduces osmotic stress by improving ionic balance within plant cells.
- It enhances the activity of antioxidant enzymes (catalase, peroxidase, superoxide dismutase), which mitigate oxidative damage during stress conditions .
The biological activity of potassium orthosilicate can be attributed to several mechanisms:
- Nutrient Availability: By solubilizing potassium and silicate ions, it enhances their availability for plant absorption.
- Enzymatic Activity: It boosts the activity of soil enzymes (urease and dehydrogenase), which are essential for nutrient cycling in the soil ecosystem .
- Cellular Mechanisms: Potassium orthosilicate has been observed to modulate cellular processes such as osmoregulation and stress response pathways.
Case Studies
Case Study 1: Salinity Stress Mitigation
In a study involving faba bean plants subjected to saline irrigation, the application of potassium orthosilicate significantly reduced the concentration of osmolytes (free amino acids and proline) compared to control treatments. This reduction indicates a lessened stress response due to improved physiological conditions facilitated by the compound .
Case Study 2: Yield Improvement
A field trial demonstrated that the application of potassium orthosilicate resulted in a notable increase in yield-related traits in crops like maize and cucumber. The results showed enhanced pod numbers and seed weights compared to untreated controls, highlighting its effectiveness as a growth promoter .
Comparative Analysis
The following table summarizes the biological activities of potassium orthosilicate compared to other potassium sources:
Property/Effect | Potassium Orthosilicate | Other Potassium Sources |
---|---|---|
Nutrient Uptake | High | Moderate |
Stress Tolerance | High | Low |
Enzymatic Activity Boost | Significant | Variable |
Yield Improvement | Significant | Moderate |
Research Findings
Recent studies have highlighted the multifaceted benefits of potassium orthosilicate:
Eigenschaften
IUPAC Name |
tetrapotassium;silicate | |
---|---|---|
Source | PubChem | |
URL | https://pubchem.ncbi.nlm.nih.gov | |
Description | Data deposited in or computed by PubChem | |
InChI |
InChI=1S/4K.O4Si/c;;;;1-5(2,3)4/q4*+1;-4 | |
Source | PubChem | |
URL | https://pubchem.ncbi.nlm.nih.gov | |
Description | Data deposited in or computed by PubChem | |
InChI Key |
RLQWHDODQVOVKU-UHFFFAOYSA-N | |
Source | PubChem | |
URL | https://pubchem.ncbi.nlm.nih.gov | |
Description | Data deposited in or computed by PubChem | |
Canonical SMILES |
[O-][Si]([O-])([O-])[O-].[K+].[K+].[K+].[K+] | |
Source | PubChem | |
URL | https://pubchem.ncbi.nlm.nih.gov | |
Description | Data deposited in or computed by PubChem | |
Molecular Formula |
K4O4Si | |
Source | PubChem | |
URL | https://pubchem.ncbi.nlm.nih.gov | |
Description | Data deposited in or computed by PubChem | |
Molecular Weight |
248.48 g/mol | |
Source | PubChem | |
URL | https://pubchem.ncbi.nlm.nih.gov | |
Description | Data deposited in or computed by PubChem | |
CAS No. |
1312-76-1, 14293-88-0 | |
Record name | Potassium polysilicate | |
Source | ChemIDplus | |
URL | https://pubchem.ncbi.nlm.nih.gov/substance/?source=chemidplus&sourceid=0001312761 | |
Description | ChemIDplus is a free, web search system that provides access to the structure and nomenclature authority files used for the identification of chemical substances cited in National Library of Medicine (NLM) databases, including the TOXNET system. | |
Record name | Potassium orthosilicate | |
Source | ChemIDplus | |
URL | https://pubchem.ncbi.nlm.nih.gov/substance/?source=chemidplus&sourceid=0014293880 | |
Description | ChemIDplus is a free, web search system that provides access to the structure and nomenclature authority files used for the identification of chemical substances cited in National Library of Medicine (NLM) databases, including the TOXNET system. | |
Record name | Potassium orthosilicate | |
Source | European Chemicals Agency (ECHA) | |
URL | https://echa.europa.eu/information-on-chemicals | |
Description | The European Chemicals Agency (ECHA) is an agency of the European Union which is the driving force among regulatory authorities in implementing the EU's groundbreaking chemicals legislation for the benefit of human health and the environment as well as for innovation and competitiveness. | |
Explanation | Use of the information, documents and data from the ECHA website is subject to the terms and conditions of this Legal Notice, and subject to other binding limitations provided for under applicable law, the information, documents and data made available on the ECHA website may be reproduced, distributed and/or used, totally or in part, for non-commercial purposes provided that ECHA is acknowledged as the source: "Source: European Chemicals Agency, http://echa.europa.eu/". Such acknowledgement must be included in each copy of the material. ECHA permits and encourages organisations and individuals to create links to the ECHA website under the following cumulative conditions: Links can only be made to webpages that provide a link to the Legal Notice page. | |
Record name | POTASSIUM ORTHOSILICATE | |
Source | FDA Global Substance Registration System (GSRS) | |
URL | https://gsrs.ncats.nih.gov/ginas/app/beta/substances/55R0S6LC84 | |
Description | The FDA Global Substance Registration System (GSRS) enables the efficient and accurate exchange of information on what substances are in regulated products. Instead of relying on names, which vary across regulatory domains, countries, and regions, the GSRS knowledge base makes it possible for substances to be defined by standardized, scientific descriptions. | |
Explanation | Unless otherwise noted, the contents of the FDA website (www.fda.gov), both text and graphics, are not copyrighted. They are in the public domain and may be republished, reprinted and otherwise used freely by anyone without the need to obtain permission from FDA. Credit to the U.S. Food and Drug Administration as the source is appreciated but not required. | |
Synthesis routes and methods
Procedure details
Q1: What are the structural characteristics of Potassium Orthosilicate?
A2: While the provided research abstracts [, ] don't delve into the detailed structural characteristics of Potassium Orthosilicate, they do provide some information. The molecular formula for Potassium Orthosilicate is K4SiO4. Further research in chemical databases and literature would be needed to obtain comprehensive information on its molecular weight, spectroscopic data, and other structural properties.
Haftungsausschluss und Informationen zu In-Vitro-Forschungsprodukten
Bitte beachten Sie, dass alle Artikel und Produktinformationen, die auf BenchChem präsentiert werden, ausschließlich zu Informationszwecken bestimmt sind. Die auf BenchChem zum Kauf angebotenen Produkte sind speziell für In-vitro-Studien konzipiert, die außerhalb lebender Organismen durchgeführt werden. In-vitro-Studien, abgeleitet von dem lateinischen Begriff "in Glas", beinhalten Experimente, die in kontrollierten Laborumgebungen unter Verwendung von Zellen oder Geweben durchgeführt werden. Es ist wichtig zu beachten, dass diese Produkte nicht als Arzneimittel oder Medikamente eingestuft sind und keine Zulassung der FDA für die Vorbeugung, Behandlung oder Heilung von medizinischen Zuständen, Beschwerden oder Krankheiten erhalten haben. Wir müssen betonen, dass jede Form der körperlichen Einführung dieser Produkte in Menschen oder Tiere gesetzlich strikt untersagt ist. Es ist unerlässlich, sich an diese Richtlinien zu halten, um die Einhaltung rechtlicher und ethischer Standards in Forschung und Experiment zu gewährleisten.