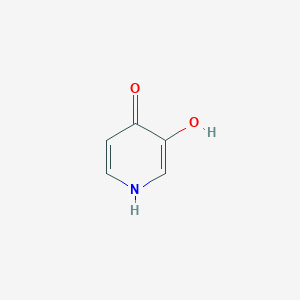
3,4-Pyridindiol
Übersicht
Beschreibung
pyridine-3,4-diol, also known as pyridine-3,4-diol, is an organic compound with the molecular formula C5H5NO2. It is a derivative of pyridine, characterized by the presence of two hydroxyl groups at the 3 and 4 positions on the pyridine ring.
Wissenschaftliche Forschungsanwendungen
Wirkmechanismus
Target of Action
Pyridine-3,4-diol, also known as 3,4-Dihydroxypyridine, is a versatile compound with various applications. It has been used as a precursor for palladium-catalyzed cross-coupling reactions . The primary targets of this compound are the enzymes involved in these reactions.
Mode of Action
It is known that it can easily be converted into bis(perfluoroalkanesulfonates), which are substrates for the construction of extended π-systems using palladium-catalyzed coupling reactions .
Biochemical Pathways
Pyridine-3,4-diol is an intermediate in the metabolism of 4-hydroxypyridine by Agrobacterium sp . It is converted into 1 mol of pyruvate, 2 mol of formate, and 1 mol of NH3 at pH 7.0 . The oxidation of pyridine-3,4-diol by crude extracts at pH 8.5 required 1 mol of O2 per mol of substrate, produced 1 mol of acid, and led to the formation of formate and a new compound with an extinction maximum of 285 nm .
Pharmacokinetics
It is known that the compound has a very low murine bone marrow toxicity, probably related to the absence of an sh-group . This suggests that it may have favorable ADME (Absorption, Distribution, Metabolism, and Excretion) properties.
Result of Action
The result of the action of pyridine-3,4-diol is the formation of extended π-systems through palladium-catalyzed coupling reactions . Fluorescence measurements of the biscoupled products showed a maximum of emission in the violet region of the spectrum . In addition, it has been shown to inhibit iodination of human thyroglobulin and interfere with mitogenic activation of human lymphocytes .
Action Environment
The action of pyridine-3,4-diol can be influenced by environmental factors such as pH. For instance, the oxidation of pyridine-3,4-diol by crude extracts requires a pH of 8.5
Biochemische Analyse
Biochemical Properties
Pyridine-3,4-diol interacts with various enzymes, proteins, and other biomolecules. For instance, it is converted by extracts into 1mol of pyruvate, 2mol of formate, and 1mol of NH3 at pH7.0 . This interaction highlights the significant role of Pyridine-3,4-diol in biochemical reactions.
Cellular Effects
The effects of Pyridine-3,4-diol on various types of cells and cellular processes are profound. It has been shown to inhibit iodination of human thyroglobulin and interfere with mitogenic activation of human lymphocytes . This indicates that Pyridine-3,4-diol can influence cell function, including impacts on cell signaling pathways, gene expression, and cellular metabolism.
Molecular Mechanism
At the molecular level, Pyridine-3,4-diol exerts its effects through binding interactions with biomolecules and changes in gene expression. For instance, it is involved in the oxidation process that requires 1mol of O2/mol of substrate, leading to the formation of formate and a new compound .
Temporal Effects in Laboratory Settings
In laboratory settings, the effects of Pyridine-3,4-diol change over time. It is involved in the metabolism of 4-hydroxypyridine, which is converted into 1mol of pyruvate, 2mol of formate, and 1mol of NH3 at pH7.0 . This indicates the product’s stability, degradation, and long-term effects on cellular function.
Metabolic Pathways
Pyridine-3,4-diol is involved in the metabolic pathways of 4-hydroxypyridine metabolism by Agrobacterium sp . It interacts with enzymes to be converted into pyruvate, formate, and NH3, indicating its significant role in metabolic flux and metabolite levels.
Vorbereitungsmethoden
Synthetic Routes and Reaction Conditions: pyridine-3,4-diol can be synthesized through the oxidation of pyridine. A common method involves the use of hydrogen peroxide or other oxidizing agents to achieve this transformation. The reaction typically requires controlled conditions to ensure the selective oxidation of pyridine to 3,4-dihydroxypyridine .
Industrial Production Methods: In industrial settings, the production of 3,4-dihydroxypyridine may involve large-scale oxidation processes using similar oxidizing agents. The reaction conditions are optimized to maximize yield and purity, often involving the use of catalysts and specific temperature and pressure conditions to facilitate the reaction .
Analyse Chemischer Reaktionen
Types of Reactions: pyridine-3,4-diol undergoes various chemical reactions, including:
Oxidation: The compound can be further oxidized to form quinones or other oxidized derivatives.
Reduction: Reduction reactions can convert 3,4-dihydroxypyridine to its corresponding dihydropyridine derivatives.
Substitution: The hydroxyl groups can participate in substitution reactions, leading to the formation of various substituted pyridine derivatives.
Common Reagents and Conditions:
Oxidation: Hydrogen peroxide, potassium permanganate, or other strong oxidizing agents.
Reduction: Sodium borohydride or other reducing agents.
Substitution: Halogenating agents, alkylating agents, or acylating agents under appropriate conditions.
Major Products Formed:
Oxidation: Quinones and other oxidized derivatives.
Reduction: Dihydropyridine derivatives.
Substitution: Substituted pyridine derivatives with various functional groups.
Vergleich Mit ähnlichen Verbindungen
1,2-Dihydroxypyridine: Another hydroxylated pyridine derivative with different substitution patterns.
1,4-Dihydroxypyridine: Known for its use in the synthesis of various pharmaceutical compounds.
Pyridine-3,4-diol: Another name for 3,4-dihydroxypyridine, highlighting its structural similarity.
Uniqueness: pyridine-3,4-diol is unique due to its specific substitution pattern, which imparts distinct chemical and biological properties. Its ability to inhibit thyroid hormone synthesis and modulate immune responses sets it apart from other hydroxylated pyridine derivatives .
Eigenschaften
IUPAC Name |
3-hydroxy-1H-pyridin-4-one | |
---|---|---|
Source | PubChem | |
URL | https://pubchem.ncbi.nlm.nih.gov | |
Description | Data deposited in or computed by PubChem | |
InChI |
InChI=1S/C5H5NO2/c7-4-1-2-6-3-5(4)8/h1-3,8H,(H,6,7) | |
Source | PubChem | |
URL | https://pubchem.ncbi.nlm.nih.gov | |
Description | Data deposited in or computed by PubChem | |
InChI Key |
ZCUUVWCJGRQCMZ-UHFFFAOYSA-N | |
Source | PubChem | |
URL | https://pubchem.ncbi.nlm.nih.gov | |
Description | Data deposited in or computed by PubChem | |
Canonical SMILES |
C1=CNC=C(C1=O)O | |
Source | PubChem | |
URL | https://pubchem.ncbi.nlm.nih.gov | |
Description | Data deposited in or computed by PubChem | |
Molecular Formula |
C5H5NO2 | |
Source | PubChem | |
URL | https://pubchem.ncbi.nlm.nih.gov | |
Description | Data deposited in or computed by PubChem | |
DSSTOX Substance ID |
DTXSID50274234 | |
Record name | 3,4-Dihydroxypyridine | |
Source | EPA DSSTox | |
URL | https://comptox.epa.gov/dashboard/DTXSID50274234 | |
Description | DSSTox provides a high quality public chemistry resource for supporting improved predictive toxicology. | |
Molecular Weight |
111.10 g/mol | |
Source | PubChem | |
URL | https://pubchem.ncbi.nlm.nih.gov | |
Description | Data deposited in or computed by PubChem | |
CAS No. |
10182-48-6, 1121-23-9 | |
Record name | 3,4-Pyridinediol | |
Source | CAS Common Chemistry | |
URL | https://commonchemistry.cas.org/detail?cas_rn=10182-48-6 | |
Description | CAS Common Chemistry is an open community resource for accessing chemical information. Nearly 500,000 chemical substances from CAS REGISTRY cover areas of community interest, including common and frequently regulated chemicals, and those relevant to high school and undergraduate chemistry classes. This chemical information, curated by our expert scientists, is provided in alignment with our mission as a division of the American Chemical Society. | |
Explanation | The data from CAS Common Chemistry is provided under a CC-BY-NC 4.0 license, unless otherwise stated. | |
Record name | 3-Hydroxypyridin-4-one | |
Source | CAS Common Chemistry | |
URL | https://commonchemistry.cas.org/detail?cas_rn=1121-23-9 | |
Description | CAS Common Chemistry is an open community resource for accessing chemical information. Nearly 500,000 chemical substances from CAS REGISTRY cover areas of community interest, including common and frequently regulated chemicals, and those relevant to high school and undergraduate chemistry classes. This chemical information, curated by our expert scientists, is provided in alignment with our mission as a division of the American Chemical Society. | |
Explanation | The data from CAS Common Chemistry is provided under a CC-BY-NC 4.0 license, unless otherwise stated. | |
Record name | 3-Hydroxy-4-pyridone | |
Source | ChemIDplus | |
URL | https://pubchem.ncbi.nlm.nih.gov/substance/?source=chemidplus&sourceid=0001121239 | |
Description | ChemIDplus is a free, web search system that provides access to the structure and nomenclature authority files used for the identification of chemical substances cited in National Library of Medicine (NLM) databases, including the TOXNET system. | |
Record name | 3,4-Dihydroxypyridine | |
Source | ChemIDplus | |
URL | https://pubchem.ncbi.nlm.nih.gov/substance/?source=chemidplus&sourceid=0010182486 | |
Description | ChemIDplus is a free, web search system that provides access to the structure and nomenclature authority files used for the identification of chemical substances cited in National Library of Medicine (NLM) databases, including the TOXNET system. | |
Record name | 3,4-Dihydroxypyridine | |
Source | EPA DSSTox | |
URL | https://comptox.epa.gov/dashboard/DTXSID50274234 | |
Description | DSSTox provides a high quality public chemistry resource for supporting improved predictive toxicology. | |
Record name | 3,4-Dihydroxypyridine | |
Source | European Chemicals Agency (ECHA) | |
URL | https://echa.europa.eu/information-on-chemicals | |
Description | The European Chemicals Agency (ECHA) is an agency of the European Union which is the driving force among regulatory authorities in implementing the EU's groundbreaking chemicals legislation for the benefit of human health and the environment as well as for innovation and competitiveness. | |
Explanation | Use of the information, documents and data from the ECHA website is subject to the terms and conditions of this Legal Notice, and subject to other binding limitations provided for under applicable law, the information, documents and data made available on the ECHA website may be reproduced, distributed and/or used, totally or in part, for non-commercial purposes provided that ECHA is acknowledged as the source: "Source: European Chemicals Agency, http://echa.europa.eu/". Such acknowledgement must be included in each copy of the material. ECHA permits and encourages organisations and individuals to create links to the ECHA website under the following cumulative conditions: Links can only be made to webpages that provide a link to the Legal Notice page. | |
Record name | 3,4-PYRIDINEDIOL | |
Source | FDA Global Substance Registration System (GSRS) | |
URL | https://gsrs.ncats.nih.gov/ginas/app/beta/substances/UW0C50CU4H | |
Description | The FDA Global Substance Registration System (GSRS) enables the efficient and accurate exchange of information on what substances are in regulated products. Instead of relying on names, which vary across regulatory domains, countries, and regions, the GSRS knowledge base makes it possible for substances to be defined by standardized, scientific descriptions. | |
Explanation | Unless otherwise noted, the contents of the FDA website (www.fda.gov), both text and graphics, are not copyrighted. They are in the public domain and may be republished, reprinted and otherwise used freely by anyone without the need to obtain permission from FDA. Credit to the U.S. Food and Drug Administration as the source is appreciated but not required. | |
Retrosynthesis Analysis
AI-Powered Synthesis Planning: Our tool employs the Template_relevance Pistachio, Template_relevance Bkms_metabolic, Template_relevance Pistachio_ringbreaker, Template_relevance Reaxys, Template_relevance Reaxys_biocatalysis model, leveraging a vast database of chemical reactions to predict feasible synthetic routes.
One-Step Synthesis Focus: Specifically designed for one-step synthesis, it provides concise and direct routes for your target compounds, streamlining the synthesis process.
Accurate Predictions: Utilizing the extensive PISTACHIO, BKMS_METABOLIC, PISTACHIO_RINGBREAKER, REAXYS, REAXYS_BIOCATALYSIS database, our tool offers high-accuracy predictions, reflecting the latest in chemical research and data.
Strategy Settings
Precursor scoring | Relevance Heuristic |
---|---|
Min. plausibility | 0.01 |
Model | Template_relevance |
Template Set | Pistachio/Bkms_metabolic/Pistachio_ringbreaker/Reaxys/Reaxys_biocatalysis |
Top-N result to add to graph | 6 |
Feasible Synthetic Routes
A: 3,4-Dihydroxypyridine (3,4-DHP) exists both as a natural and synthetic compound. In nature, it is found as a product of mimosine degradation by certain rumen bacteria. [, ] It can also be synthesized through various chemical methods. []
A: Research suggests that 3,4-DHP exhibits goitrogenic activity, meaning it can interfere with thyroid hormone production. [, , ] While its mechanism is not fully elucidated, it has been shown to inhibit the iodination of human thyroglobulin, a crucial step in thyroid hormone synthesis. [] Unlike conventional antithyroid agents, 3,4-DHP lacks a sulfhydryl group, potentially impacting its toxicity profile. []
A: In the rumen of some ruminant animals, mimosine, a non-protein amino acid found in plants like Leucaena, is degraded to 3,4-DHP. [, , , ] This conversion is significant because 3,4-DHP is a goitrogen, potentially leading to leucaena toxicosis in animals that lack specific ruminal bacteria capable of further degrading it. [, , , ]
A: Synergistes jonesii is a bacterial species residing in the rumen of certain ruminants. It plays a crucial role in detoxifying 3,4-DHP, thereby protecting the animal from leucaena toxicosis. [, ] The presence of S. jonesii in the rumen is geographically variable, explaining why leucaena toxicity is more prevalent in certain regions. [, ]
A: Researchers have developed cultural tests to identify the presence of 3,4-DHP-degrading bacteria, like Synergistes jonesii, in rumen fluid samples. [] These tests are valuable in assessing the susceptibility of ruminants to leucaena toxicosis and the potential need for introducing DHP-degrading bacteria into their rumen. []
A: 3,4-DHP exists in equilibrium with its tautomeric form, 3-hydroxypyridin-4-one. [, ] This tautomerism influences its chemical properties and interactions with biological systems. [, ] The presence of hydroxyl groups and the nitrogen atom in its structure are crucial for its binding to enzymes and receptors, ultimately dictating its biological effects. [, ]
A: Trimethylsilylation of mimosine, a precursor to 3,4-DHP, can lead to the formation of dehydroalanine as an artifact. [] This finding has significant implications for GC-MS based metabolomics studies, where it could lead to misinterpretation of results. [] Researchers need to be aware of this potential artifact and implement appropriate controls and data analysis methods.
A: 3,4-DHP can act as a ligand in coordination chemistry, forming complexes with metal ions. [, , , ] This property has been exploited in developing heterogeneous catalysts for organic reactions, particularly palladium-catalyzed cross-coupling reactions. [, , ] The ability of 3,4-DHP to form stable complexes with metals makes it a promising candidate for designing new materials with tailored properties.
A: Researchers have synthesized nano-Fe3O4 particles functionalized with 3,4-DHP. [, ] These nanoparticles have been successfully employed as catalysts in organic synthesis, highlighting the potential of 3,4-DHP in nanomaterial applications. [, ] The magnetic properties of these nanoparticles enable their easy recovery and reusability, making them attractive for sustainable catalytic processes.
A: Research shows that 3,4-DHP can participate in the self-assembly of boron-based macrocycles and dendrimers. [] This self-assembly process relies on the formation of dative bonds between boronate esters and the nitrogen atom of 3,4-DHP. [] The ability to control the assembly process through the choice of building blocks opens avenues for designing novel materials with unique properties.
A: Studies have demonstrated that 3,4-DHP can serve as a precursor for the biosynthesis of L-mimosine in plants. [] This process involves the enzymatic condensation of 3,4-DHP with O-acetyl-L-serine, catalyzed by the enzyme beta-(pyrazol-1-yl)-L-alanine/L-cysteine synthase (beta-PA/CSase). [] This finding provides insights into the metabolic pathways of plant secondary metabolites and their potential applications in biotechnology.
A: While rumen microbes are known to degrade mimosine into 3,4-DHP, research suggests the existence of an alternative degradation pathway in Leucaena seedlings. [] This pathway, mediated by a specific C-N lyase, bypasses 3,4-DHP formation, directly cleaving mimosine into other products. [] Further investigation into this alternative pathway could provide valuable insights for mitigating mimosine toxicity.
Haftungsausschluss und Informationen zu In-Vitro-Forschungsprodukten
Bitte beachten Sie, dass alle Artikel und Produktinformationen, die auf BenchChem präsentiert werden, ausschließlich zu Informationszwecken bestimmt sind. Die auf BenchChem zum Kauf angebotenen Produkte sind speziell für In-vitro-Studien konzipiert, die außerhalb lebender Organismen durchgeführt werden. In-vitro-Studien, abgeleitet von dem lateinischen Begriff "in Glas", beinhalten Experimente, die in kontrollierten Laborumgebungen unter Verwendung von Zellen oder Geweben durchgeführt werden. Es ist wichtig zu beachten, dass diese Produkte nicht als Arzneimittel oder Medikamente eingestuft sind und keine Zulassung der FDA für die Vorbeugung, Behandlung oder Heilung von medizinischen Zuständen, Beschwerden oder Krankheiten erhalten haben. Wir müssen betonen, dass jede Form der körperlichen Einführung dieser Produkte in Menschen oder Tiere gesetzlich strikt untersagt ist. Es ist unerlässlich, sich an diese Richtlinien zu halten, um die Einhaltung rechtlicher und ethischer Standards in Forschung und Experiment zu gewährleisten.