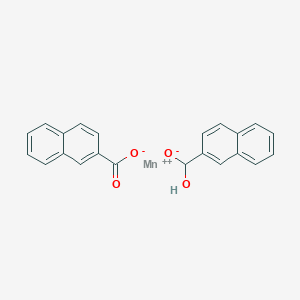
Naphthenische Säuren, Mangansalze
- Klicken Sie auf QUICK INQUIRY, um ein Angebot von unserem Expertenteam zu erhalten.
- Mit qualitativ hochwertigen Produkten zu einem WETTBEWERBSFÄHIGEN Preis können Sie sich mehr auf Ihre Forschung konzentrieren.
Übersicht
Beschreibung
Manganese naphthenate are a class of compounds formed by the reaction of naphthenic acids with manganese ions. Naphthenic acids are a complex mixture of cycloaliphatic carboxylic acids found in crude oil. These acids are known for their role in corrosion and environmental toxicity. When combined with manganese, they form manganese naphthenates, which are used in various industrial applications due to their unique properties.
Wissenschaftliche Forschungsanwendungen
Manganese naphthenates have a wide range of applications in scientific research:
Chemistry: Used as catalysts in organic synthesis and polymerization reactions.
Biology: Studied for their potential antimicrobial properties.
Medicine: Investigated for their role in drug delivery systems due to their ability to form stable complexes with various drugs.
Industry: Used as additives in lubricants, paints, and coatings to improve their properties.
Wirkmechanismus
Target of Action
Naphthenic acids, manganese salts, often referred to as manganese naphthenates, primarily target metal ions . They are widely used as hydrophobic sources of metal ions in diverse applications . The main fractions of naphthenic acids are carboxylic acids with a carbon backbone of 9 to 20 carbons .
Mode of Action
Naphthenic acids, manganese salts interact with their targets through a process where naphthenic acids occurring naturally in crude oil react with metal ions in the formation of water to produce organic salts . This process occurs as the reservoir fluid is depressurized during normal production .
Biochemical Pathways
Studies have shown that they can perturb gene networks related to metabolic processes, membrane integrity, and gut function .
Pharmacokinetics
It is known that the structure and composition of naphthenic acids, as well as environmental factors such as the presence of specific electron acceptors and trace metals, are important drivers in shaping their behavior .
Result of Action
The result of the action of naphthenic acids, manganese salts is the formation of organic salts . These salts can cause corrosion in oil processing and refining units, particularly in distillation units . They can also affect the performance and integrity of processes and facilities in the oilfield .
Action Environment
The action of naphthenic acids, manganese salts is influenced by various environmental factors. For example, the presence of specific electron acceptors, trace metals, and competition for substrates from non-naphthenic acid-degrading microbes are important drivers in shaping their behavior . Additionally, the pH and acid structure can modulate the interactions of naphthenic acids, affecting their interfacial behavior and emulsion stability .
Biochemische Analyse
Cellular Effects
It is known that they can have toxic effects, particularly on liver cells .
Molecular Mechanism
It is known that they can interact with various biomolecules, potentially influencing enzyme activity and gene expression .
Temporal Effects in Laboratory Settings
The effects of Naphthenic acids, manganese salts can change over time in laboratory settings. Factors such as evaporation and biodegradation can influence the compounds of Naphthenic acids .
Dosage Effects in Animal Models
The effects of Naphthenic acids, manganese salts can vary with different dosages in animal models. High concentrations can have toxic effects .
Metabolic Pathways
Naphthenic acids, manganese salts are involved in various metabolic pathways. The specific enzymes or cofactors they interact with are not well known .
Vorbereitungsmethoden
Synthetic Routes and Reaction Conditions: The preparation of manganese naphthenates typically involves the neutralization of naphthenic acids with manganese hydroxide or manganese carbonate. The reaction is carried out in a solvent such as toluene or xylene, under reflux conditions to ensure complete reaction. The general reaction can be represented as:
Naphthenic Acid+Manganese Hydroxide→Manganese Naphthenate+Water
Industrial Production Methods: In industrial settings, manganese naphthenates are produced by reacting naphthenic acids with manganese salts in large reactors. The process involves heating the mixture to around 150-200°C and maintaining the temperature until the reaction is complete. The product is then purified by distillation to remove any unreacted acids and solvents.
Types of Reactions:
Oxidation: Manganese naphthenates can undergo oxidation reactions, especially in the presence of strong oxidizing agents.
Reduction: These compounds can be reduced to manganese metal or lower oxidation states of manganese under specific conditions.
Substitution: Manganese naphthenates can participate in substitution reactions where the naphthenic acid moiety is replaced by other ligands.
Common Reagents and Conditions:
Oxidizing Agents: Hydrogen peroxide, potassium permanganate.
Reducing Agents: Hydrogen gas, sodium borohydride.
Solvents: Toluene, xylene, and other non-polar solvents.
Major Products:
Oxidation: Manganese dioxide and oxidized naphthenic acids.
Reduction: Manganese metal and reduced naphthenic acids.
Substitution: Various manganese complexes with different ligands.
Vergleich Mit ähnlichen Verbindungen
Cobalt Naphthenates: Similar to manganese naphthenates but with cobalt as the central metal ion.
Nickel Naphthenates: Contain nickel instead of manganese and have different catalytic properties.
Zinc Naphthenates: Used in similar applications but with different reactivity and stability profiles.
Uniqueness: Manganese naphthenates are unique due to their specific catalytic properties and their ability to form stable complexes with a wide range of ligands. They are also less toxic compared to some other metal naphthenates, making them more suitable for certain applications.
Biologische Aktivität
Manganese naphthenate is a manganese salt of naphthenic acid, primarily used as a drying agent in paints and coatings due to its catalytic properties. This compound has garnered attention for its biological activity, particularly in relation to its potential toxicity and therapeutic applications. This article delves into the biological activity of manganese naphthenate, supported by research findings, case studies, and data tables.
Manganese naphthenate exhibits unique properties that contribute to its biological activity. As a transition metal complex, it can participate in redox reactions, influencing various biochemical pathways. Manganese serves as an essential cofactor for several enzymes, including superoxide dismutase, which plays a crucial role in mitigating oxidative stress in cells.
Mechanisms of Action:
- Catalytic Activity: Manganese naphthenate acts as an effective catalyst in oxidation reactions, facilitating the conversion of organic compounds through free radical mechanisms .
- Neurotoxicity: Excessive exposure to manganese compounds, including manganese naphthenate, has been linked to neurotoxic effects similar to Parkinson's disease. This is primarily due to the generation of reactive oxygen species (ROS) and subsequent oxidative stress .
In Vitro Studies
Research has demonstrated that manganese naphthenate can induce cytotoxic effects in various cell lines. The extent of toxicity is influenced by factors such as concentration, exposure duration, and cellular context.
- Cytotoxicity: A study indicated that exposure to manganese naphthenate resulted in increased ROS production and cell death in neuronal cell lines .
- Neurotransmitter Disruption: Manganese exposure has been associated with alterations in neurotransmitter levels, potentially leading to neurological disorders .
In Vivo Studies
Animal studies have provided further insights into the biological effects of manganese naphthenate.
- Carcinogenicity: The National Toxicology Program (NTP) has reviewed various studies indicating that manganese compounds may have carcinogenic potential under certain conditions. However, specific data on manganese naphthenate remains limited .
- Neurological Effects: Animal models exposed to manganese have shown signs of neurotoxicity, including behavioral changes and histopathological alterations in brain tissue .
Case Studies
- Occupational Exposure: A study examining workers exposed to manganese naphthenate found elevated levels of manganese in blood samples, correlating with neurological symptoms such as tremors and cognitive impairment .
- Therapeutic Applications: Research into the use of manganese nanoparticles suggests potential applications in cancer therapy due to their ability to enhance radiotherapeutic efficiency by acting as radiosensitizers .
Data Table: Summary of Biological Effects
Eigenschaften
IUPAC Name |
hydroxy(naphthalen-2-yl)methanolate;manganese(2+);naphthalene-2-carboxylate |
Source
|
---|---|---|
Source | PubChem | |
URL | https://pubchem.ncbi.nlm.nih.gov | |
Description | Data deposited in or computed by PubChem | |
InChI |
InChI=1S/C11H9O2.C11H8O2.Mn/c2*12-11(13)10-6-5-8-3-1-2-4-9(8)7-10;/h1-7,11-12H;1-7H,(H,12,13);/q-1;;+2/p-1 |
Source
|
Source | PubChem | |
URL | https://pubchem.ncbi.nlm.nih.gov | |
Description | Data deposited in or computed by PubChem | |
InChI Key |
BFWHHDQLPCRKOU-UHFFFAOYSA-M |
Source
|
Source | PubChem | |
URL | https://pubchem.ncbi.nlm.nih.gov | |
Description | Data deposited in or computed by PubChem | |
Canonical SMILES |
C1=CC=C2C=C(C=CC2=C1)C(O)[O-].C1=CC=C2C=C(C=CC2=C1)C(=O)[O-].[Mn+2] |
Source
|
Source | PubChem | |
URL | https://pubchem.ncbi.nlm.nih.gov | |
Description | Data deposited in or computed by PubChem | |
Molecular Formula |
C22H16MnO4 |
Source
|
Source | PubChem | |
URL | https://pubchem.ncbi.nlm.nih.gov | |
Description | Data deposited in or computed by PubChem | |
Molecular Weight |
399.3 g/mol |
Source
|
Source | PubChem | |
URL | https://pubchem.ncbi.nlm.nih.gov | |
Description | Data deposited in or computed by PubChem | |
CAS No. |
1336-93-2 |
Source
|
Record name | Naphthenic acids, manganese salts | |
Source | ChemIDplus | |
URL | https://pubchem.ncbi.nlm.nih.gov/substance/?source=chemidplus&sourceid=0001336932 | |
Description | ChemIDplus is a free, web search system that provides access to the structure and nomenclature authority files used for the identification of chemical substances cited in National Library of Medicine (NLM) databases, including the TOXNET system. | |
Haftungsausschluss und Informationen zu In-Vitro-Forschungsprodukten
Bitte beachten Sie, dass alle Artikel und Produktinformationen, die auf BenchChem präsentiert werden, ausschließlich zu Informationszwecken bestimmt sind. Die auf BenchChem zum Kauf angebotenen Produkte sind speziell für In-vitro-Studien konzipiert, die außerhalb lebender Organismen durchgeführt werden. In-vitro-Studien, abgeleitet von dem lateinischen Begriff "in Glas", beinhalten Experimente, die in kontrollierten Laborumgebungen unter Verwendung von Zellen oder Geweben durchgeführt werden. Es ist wichtig zu beachten, dass diese Produkte nicht als Arzneimittel oder Medikamente eingestuft sind und keine Zulassung der FDA für die Vorbeugung, Behandlung oder Heilung von medizinischen Zuständen, Beschwerden oder Krankheiten erhalten haben. Wir müssen betonen, dass jede Form der körperlichen Einführung dieser Produkte in Menschen oder Tiere gesetzlich strikt untersagt ist. Es ist unerlässlich, sich an diese Richtlinien zu halten, um die Einhaltung rechtlicher und ethischer Standards in Forschung und Experiment zu gewährleisten.