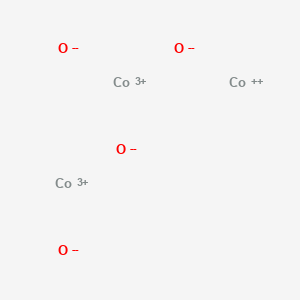
Cobalt oxide
Übersicht
Beschreibung
Cobalt oxide refers to a group of inorganic compounds primarily composed of cobalt and oxygen. The three principal oxides are:
Cobalt(II) oxide (CoO): Also known as cobaltous oxide, it is a grayish-green crystalline solid with a rock-salt structure .
Cobalt(III) oxide (Co₂O₃): Known as cobaltic oxide, it is a black powder unstable at high temperatures .
Cobalt(II,III) oxide (Co₃O₄): Often termed cobaltosic oxide, this mixed-valence compound (Co²⁺ and Co³⁺) is the most stable and industrially relevant form. It exhibits a spinel structure and decomposes to CoO at temperatures above 900°C .
This compound nanoparticles (NPs) are widely used in catalysis, energy storage, and electronics due to their redox activity, thermal stability, and magnetic properties .
Vorbereitungsmethoden
Sol-Gel Synthesis of Cobalt Oxide Nanoparticles
Synthesis Procedure
The sol-gel method involves the formation of a colloidal suspension (sol) that transitions into a gel-like network, followed by calcination to yield crystalline nanoparticles. A representative protocol involves dissolving cobalt acetate (0.3 M) in deionized water, followed by dropwise addition of oxalic acid (0.3 M) under continuous stirring. The resulting pink precipitate is washed, dried at 100°C, and calcined at 200–400°C to produce black Co3O4 nanoparticles .
Influence of Calcination Temperature
Calcination temperature critically impacts crystallinity and phase purity. X-ray diffraction (XRD) analysis of samples calcined at 200°C, 300°C, and 400°C confirmed cubic-phase Co3O4 (JCPDS 43-1003), with peak intensities sharpening at higher temperatures, indicating improved crystallinity . Magnetic susceptibility measurements revealed a value of +7380 × 10⁻⁶ cm³/mol, consistent with bulk Co3O4 .
Table 1: Effect of Calcination Temperature on Crystallite Size and Phase Purity
Temperature (°C) | Crystallite Size (nm) | Phase Identified |
---|---|---|
200 | 12 ± 2 | Cubic Co3O4 |
300 | 18 ± 3 | Cubic Co3O4 |
400 | 25 ± 4 | Cubic Co3O4 |
Advantages and Limitations
The sol-gel method offers precise control over stoichiometry and particle size distribution. However, it requires careful pH adjustment to prevent premature precipitation and organic residue formation .
Precipitation Method for Scalable Synthesis
Optimization of Reaction Parameters
A study optimizing antibacterial efficacy varied cobalt nitrate (0.2–0.6 M) and KOH (1–3 M) concentrations, with stirring times of 30–90 minutes . Higher cobalt nitrate (0.6 M) and KOH (3 M) concentrations reduced Gram-positive bacterial survival to 4.40 log10 CFU/mL, attributed to increased nanoparticle surface reactivity .
Table 2: Impact of Synthesis Parameters on Antibacterial Activity
Cobalt Nitrate (M) | KOH (M) | Stirring Time (min) | Bacterial Survival (log10 CFU/mL) |
---|---|---|---|
0.2 | 1 | 30 | 6.42 (Gram+), 6.76 (Gram–) |
0.6 | 3 | 90 | 4.40 (Gram+), 4.75 (Gram–) |
Mechanism of Nanoparticle Formation
In alkaline conditions, Co²⁺ ions react with OH⁻ to form Co(OH)₂, which dehydrates at elevated temperatures to Co3O4 . Prolonged stirring (90 minutes) enhances homogeneity, while excess KOH promotes smaller particle sizes via Ostwald ripening inhibition .
Precursor-Dependent Synthesis and Supported Catalysts
Role of Precursor Chemistry
This compound supported on γ-Al2O3 was synthesized using nitrate, acetate, citrate, and acetylacetonate precursors . Nitrate-derived samples exhibited reducible Co3O4 phases, whereas citrate precursors formed non-reducible CoAl2O4 spinels due to stronger metal-support interactions .
Table 3: Precursor Effects on Reducibility and Phase Composition
Precursor | Reduction Onset (°C) | Phase Identified | Reducibility (%) |
---|---|---|---|
Cobalt Nitrate | 220 | Co3O4 | 99.5 |
Cobalt Citrate | 320 | CoAl2O4 | <10 |
Thermal Decomposition Pathways
Thermogravimetric analysis of cobalt acetate decomposition revealed a three-step process: dehydration (50–150°C), acetate ligand oxidation (150–300°C), and Co3O4 formation (>300°C) . Supported catalysts calcined at 450°C showed 11.29 wt% cobalt dispersion, with XRD confirming absence of bulk Co3O4 phases .
Hydrothermal and Alternative Methods
Electrospinning and Combustion Synthesis
Electrospinning produces fibrous this compound structures by calcining polymer-cobalt salt composites, enabling high surface-area morphologies . Solution combustion synthesis, using fuels like glycine, offers rapid, energy-efficient nanoparticle production but risks impurity incorporation .
Comparative Analysis of Synthesis Methods
Table 4: Method Comparison for this compound Nanoparticle Synthesis
Method | Particle Size (nm) | Crystallinity | Energy Efficiency | Scalability |
---|---|---|---|---|
Sol-Gel | 12–25 | High | Moderate | Laboratory |
Precipitation | 5–30 | Moderate | High | Industrial |
Hydrothermal | 10–50 | High | Low | Pilot-scale |
Analyse Chemischer Reaktionen
Cobalt oxide undergoes various chemical reactions:
Oxidation-Reduction Reactions: The compound can be reduced to cobalt(II) oxide at high temperatures.
Catalytic Reactions: The catalytic properties of cobalt tetraoxide are influenced by the arrangement of surface metal and oxygen atoms.
Reactions with Acids and Alkalis: This compound is soluble in acids and alkalis with degradation, forming corresponding cobalt salts.
Wissenschaftliche Forschungsanwendungen
Energy Storage Applications
Cobalt oxide is extensively studied as an electrode material in energy storage devices such as supercapacitors and lithium-ion batteries. Its high conductivity and electrochemical stability make it suitable for these applications.
Table 1: Properties of this compound in Energy Storage
Property | Value |
---|---|
Conductivity | High |
Specific Capacity | Up to 1200 mAh/g |
Cycle Stability | > 1000 cycles |
Voltage Window | 0-4.5 V |
Case Study: Lithium-Ion Batteries
Research has shown that this compound nanoparticles can enhance the performance of lithium-ion batteries by improving charge/discharge rates and cycle life. For instance, a study demonstrated that incorporating this compound into the anode materials significantly increased the overall battery efficiency, achieving a power conversion efficiency of up to 12% when used as a dopant in hybrid solar cells .
Catalytic Applications
This compound serves as an effective catalyst in various chemical reactions, including oxidation processes and environmental remediation.
Table 2: Catalytic Properties of this compound
Reaction Type | Catalyst Type | Efficiency |
---|---|---|
Water Oxidation | This compound Nanoparticles | High yield |
CO Oxidation | Supported Cobalt Catalysts | > 90% conversion |
Decomposition of Organic Pollutants | This compound Films | Significant reduction |
Case Study: Environmental Remediation
this compound has been utilized to degrade organic pollutants in wastewater treatment. A study indicated that this compound nanoparticles effectively catalyzed the degradation of dyes in industrial effluents, demonstrating their potential for environmental cleanup .
Biomedical Applications
This compound nanoparticles are being explored for various biomedical applications due to their antibacterial properties and biocompatibility.
Table 3: Biomedical Applications of this compound
Application | Description | Efficacy |
---|---|---|
Antibacterial Agents | Inhibits bacterial growth | Effective against multiple strains |
Drug Delivery Systems | Enhances drug uptake | Improved bioavailability |
Imaging Contrast Agents | Used in MRI | Enhanced imaging clarity |
Case Study: Antimicrobial Activity
Research demonstrated that this compound nanoparticles exhibited significant antibacterial activity against pathogens such as E. coli and S. aureus. The nanoparticles were shown to induce oxidative stress in bacterial cells, leading to cell death . Additionally, this compound has been investigated for its use in drug delivery systems, enhancing therapeutic efficacy by facilitating targeted delivery .
Electronics and Optoelectronics
This compound's semiconducting properties make it suitable for various electronic applications, including sensors and photovoltaic devices.
Table 4: Electronic Applications of this compound
Application | Type | Performance |
---|---|---|
Gas Sensors | This compound-Based Sensors | High sensitivity |
Photovoltaic Cells | Dye-Sensitized Solar Cells | Conversion efficiency up to 6.7% |
Transparent Conductors | Used in display technologies | High transparency and conductivity |
Case Study: Gas Sensors
this compound-based sensors have been developed for detecting harmful gases such as carbon monoxide and volatile organic compounds. These sensors demonstrate high sensitivity and selectivity due to the unique electronic properties of this compound nanoparticles .
Wirkmechanismus
The mechanism of action of cobalt tetraoxide involves its redox properties and the arrangement of cobalt ions in the spinel structure. The coexistence of tetrahedral Co(II) and octahedral Co(III) sites is linked to its catalytic activity. The shape and size of the nanoparticles can significantly alter the redox features and catalytic properties by exposing specific reactive crystal facets .
Vergleich Mit ähnlichen Verbindungen
Comparison with Other Cobalt Compounds
Cobalt oxide is often compared to soluble cobalt salts (e.g., cobalt chloride) and organometallic precursors (e.g., cobalt oxalate) in terms of applications and toxicity:
Key Findings :
- Toxicity: Soluble cobalt compounds (e.g., CoCl₂) exhibit higher genotoxicity than particulate this compound due to ion release .
- Applications : this compound’s stability makes it preferable for high-temperature processes (e.g., catalysis), while cobalt sulfate dominates battery manufacturing due to solubility .
Comparison with Transition Metal Oxides
Electrochemical Performance
This compound’s electrochemical activity is benchmarked against oxides like nickel cobaltite (NiCo₂O₄) and iron-doped variants:
Research Insights :
- MXene/N,S-rGO composites enhance Co₃O₄’s capacitance by 40% compared to bare Co₃O₄ .
- Fe-doped Co₃O₄ NPs achieve 99% conversion in sulfoxidation reactions, outperforming Ni-doped variants .
Catalytic Activity
This compound’s catalytic efficiency is contrasted with ferrites (e.g., CoFe₂O₄) and zincates (CoZnO):
Notable Trends:
- Co₃O₄/TiO₂ catalysts exhibit stronger metal-support interactions than SiO₂ or Al₂O₃ variants, enhancing stability .
- CoFe₂O₄’s exothermic activity is attributed to Fe³⁺ redox cycling, absent in pure Co₃O₄ .
Toxicity Comparison with Metal Oxide Nanoparticles
This compound NPs show moderate toxicity relative to other metal oxides:
Critical Notes:
Biologische Aktivität
Cobalt oxide (Co₃O₄) is a compound that has garnered significant attention in biomedical research due to its diverse biological activities, including anticancer, antibacterial, and antioxidant properties. This article provides a comprehensive overview of the biological activity of this compound, focusing on its synthesis, characterization, and various applications supported by recent research findings.
Synthesis and Characterization of this compound Nanoparticles
This compound nanoparticles (Co₃O₄-NPs) can be synthesized through various methods, including thermal decomposition, green synthesis using plant extracts, and microbial synthesis. These methods not only influence the size and morphology of the nanoparticles but also their biological properties.
- Thermal Decomposition : This method involves heating cobalt salts to produce nanoparticles with controlled size and phase purity. For instance, Co₃O₄-NPs synthesized via urea-based thermal decomposition exhibited a particle size of approximately 36 nm .
- Green Synthesis : Recent studies have highlighted eco-friendly approaches using plant extracts, such as Psidium guajava and Curcuma longa, which yield nanoparticles with enhanced biocompatibility and stability .
Anticancer Activity
This compound nanoparticles have demonstrated promising anticancer properties in various studies:
- Cytotoxicity : In vitro studies showed significant cytotoxic effects against human colorectal cancer cell lines (HT29 and SW620), with IC₅₀ values of 2.26 μg/mL for HT29 cells and 394.5 μg/mL for SW620 cells . The mechanism of action involves apoptosis induction, evidenced by increased expression of pro-apoptotic markers following exposure to Co₃O₄-NPs.
- Cell Viability : The viability of MCF-7 breast cancer cells decreased significantly when treated with Co₃O₄-NPs, showing a concentration-dependent response where cell viability dropped from 90% to 52% at increasing concentrations .
Antibacterial Activity
This compound nanoparticles exhibit notable antibacterial effects against both Gram-positive and Gram-negative bacteria:
- Inhibition Zones : Studies reported inhibition zones ranging from 7 mm to 18 mm against Staphylococcus aureus (Gram-positive) and Escherichia coli (Gram-negative) .
- Mechanism : The antibacterial mechanism is thought to involve the generation of reactive oxygen species (ROS) upon interaction with bacterial cells, leading to oxidative stress and cell death .
Antioxidant Activity
Research indicates that Co₃O₄-NPs possess antioxidant properties:
- Antioxidant Assays : The antioxidant activity increases with nanoparticle concentration, suggesting potential applications in reducing oxidative stress in biological systems .
Case Studies
- Cytotoxicity in Cancer Cells :
- Antibacterial Efficacy :
Table 1: Summary of Anticancer Effects of this compound Nanoparticles
Cell Line | IC₅₀ (μg/mL) | Mechanism |
---|---|---|
HT29 | 2.26 | Apoptosis induction |
SW620 | 394.5 | Apoptosis induction |
MCF-7 | Varies | Cytotoxicity |
HCT116 | Varies | Cytotoxicity |
Table 2: Antibacterial Activity of this compound Nanoparticles
Bacteria | Inhibition Zone (mm) | Type |
---|---|---|
Staphylococcus aureus | 7 - 18 | Gram-positive |
Escherichia coli | 7 - 18 | Gram-negative |
Q & A
Basic Research Questions
Q. What are the standard methods for characterizing the crystal structure and morphology of cobalt oxide nanoparticles?
To determine crystal structure, X-ray diffraction (XRD) is used to identify phases (e.g., CoO vs. Co₃O₄) by comparing peak positions to reference databases. Transmission electron microscopy (TEM) provides nanoscale morphology and particle size distribution. Hydrogen temperature-programmed reduction (H₂-TPR) profiles (e.g., distinct reduction peaks for Co₃O₄ → CoO → Co) reveal redox properties and surface reactivity . For phase differentiation, energy-dispersive X-ray spectroscopy (EDS) or Raman spectroscopy can supplement structural analysis .
Q. How can researchers ensure safe handling and storage of this compound in laboratory settings?
Follow safety data sheet (SDS) guidelines: store this compound in a locked, ventilated area to prevent moisture absorption or contamination. Use PPE (gloves, lab coats, respirators) to avoid inhalation of dust. In case of exposure, rinse affected areas with water and seek medical attention. Avoid incompatible materials (e.g., strong acids) to prevent hazardous reactions .
Q. What analytical techniques differentiate between cobalt(II) oxide (CoO) and cobalt(III,II) oxide (Co₃O₄)?
XRD distinguishes CoO (cubic structure, PDF #43-1003) from Co₃O₄ (spinel structure, PDF #42-1467) via distinct diffraction patterns. X-ray photoelectron spectroscopy (XPS) identifies oxidation states: Co²⁺ (780–782 eV binding energy) and Co³⁺ (779–780 eV). Thermogravimetric analysis (TGA) under air can also reveal phase transitions (e.g., Co₃O₄ decomposition at ~900°C) .
Advanced Research Questions
Q. How can experimental design resolve contradictions in reported catalytic activities of cobalt oxides?
Use factorial screening (e.g., varying aging temperature, surfactant concentration, reductant ratio) to systematically study synthesis-structure-activity relationships. For example, a 2⁶ factorial design (Table 1 in ) isolates variables impacting Co₃O₄ crystallinity and surface area. Pair this with in-situ characterization (e.g., operando XRD) to link synthesis conditions to catalytic performance .
Q. What methodologies benchmark the oxygen evolution reaction (OER) activity of this compound electrocatalysts?
Measure overpotential (η) at 10 mA cm⁻² geometric current density in 1 M KOH or 0.5 M H₂SO₄. Calculate turnover frequency (TOF) using electrochemically active surface area (ECSA) from double-layer capacitance. Stability tests (e.g., chronopotentiometry at 10 mA cm⁻² for 24 hours) and Faradaic efficiency (via gas chromatography) are critical. Co₃O₄ typically achieves η ≈ 350–430 mV in alkaline media but degrades in acidic conditions .
Q. How do synthesis parameters influence the redox properties of this compound catalysts?
Higher aging temperatures (e.g., 270°C vs. 240°C) increase crystallinity, reducing surface defects but lowering reducibility (evident in H₂-TPR peak shifts). Surfactant concentration (0.05–0.25 M) controls pore structure, affecting oxygen mobility. For example, 14 wt.% Co₃O₄/OMWNTs showed higher reducibility (H₂-TPR peak at 320°C) compared to 10 wt.% .
Q. What advanced techniques probe reaction mechanisms on this compound surfaces under operational conditions?
In-situ Fourier-transform infrared spectroscopy (FTIR) identifies intermediate species (e.g., *OOH during OER). X-ray absorption spectroscopy (XAS) at synchrotron facilities tracks Co oxidation state changes during catalysis. Electrochemical mass spectrometry (EC-MS) correlates potential with gas evolution rates .
Q. How can macroscopic quantum phenomena be observed in ultrapure this compound derivatives like PdCoO₂?
Measure magnetoresistance in ultrahigh-purity PdCoO₂ single crystals under strong magnetic fields (e.g., 10 Tesla). Coherent electron wave interference manifests as periodic oscillations in conductivity, observable over ~0.01 mm scales. Purity (>99.99%) minimizes defect scattering, enabling macroscopic quantum coherence .
Q. Data Analysis & Reproducibility
Q. How should researchers address discrepancies in reported OER overpotentials for this compound?
Normalize current density to ECSA instead of geometric area. Report electrolyte pH, temperature, and reference electrode calibration. Compare with standard catalysts (e.g., IrO₂) under identical conditions. Use statistical tools (e.g., error bars from triplicate experiments) to quantify uncertainty .
Q. What statistical approaches improve reproducibility in this compound synthesis?
Employ design of experiments (DoE) to quantify variable interactions (e.g., ANOVA for aging temperature vs. surfactant effects). Share raw characterization data (XRD, TEM) in supplementary files with metadata (instrument settings, calibration details) .
Eigenschaften
IUPAC Name |
cobalt(2+);cobalt(3+);oxygen(2-) | |
---|---|---|
Source | PubChem | |
URL | https://pubchem.ncbi.nlm.nih.gov | |
Description | Data deposited in or computed by PubChem | |
InChI |
InChI=1S/3Co.4O/q+2;2*+3;4*-2 | |
Source | PubChem | |
URL | https://pubchem.ncbi.nlm.nih.gov | |
Description | Data deposited in or computed by PubChem | |
InChI Key |
UBEWDCMIDFGDOO-UHFFFAOYSA-N | |
Source | PubChem | |
URL | https://pubchem.ncbi.nlm.nih.gov | |
Description | Data deposited in or computed by PubChem | |
Canonical SMILES |
[O-2].[O-2].[O-2].[O-2].[Co+2].[Co+3].[Co+3] | |
Source | PubChem | |
URL | https://pubchem.ncbi.nlm.nih.gov | |
Description | Data deposited in or computed by PubChem | |
Molecular Formula |
Co3O4 | |
Source | PubChem | |
URL | https://pubchem.ncbi.nlm.nih.gov | |
Description | Data deposited in or computed by PubChem | |
Molecular Weight |
240.797 g/mol | |
Source | PubChem | |
URL | https://pubchem.ncbi.nlm.nih.gov | |
Description | Data deposited in or computed by PubChem | |
Physical Description |
Dry Powder; Pellets or Large Crystals, Dry Powder; Dry Powder, Other Solid, Black or grey solid; [Merck Index] Black powder, insoluble in water; [MSDSonline] | |
Record name | Cobalt oxide | |
Source | EPA Chemicals under the TSCA | |
URL | https://www.epa.gov/chemicals-under-tsca | |
Description | EPA Chemicals under the Toxic Substances Control Act (TSCA) collection contains information on chemicals and their regulations under TSCA, including non-confidential content from the TSCA Chemical Substance Inventory and Chemical Data Reporting. | |
Record name | Cobalt oxide (Co3O4) | |
Source | EPA Chemicals under the TSCA | |
URL | https://www.epa.gov/chemicals-under-tsca | |
Description | EPA Chemicals under the Toxic Substances Control Act (TSCA) collection contains information on chemicals and their regulations under TSCA, including non-confidential content from the TSCA Chemical Substance Inventory and Chemical Data Reporting. | |
Record name | Cobaltic-cobaltous oxide | |
Source | Haz-Map, Information on Hazardous Chemicals and Occupational Diseases | |
URL | https://haz-map.com/Agents/8043 | |
Description | Haz-Map® is an occupational health database designed for health and safety professionals and for consumers seeking information about the adverse effects of workplace exposures to chemical and biological agents. | |
Explanation | Copyright (c) 2022 Haz-Map(R). All rights reserved. Unless otherwise indicated, all materials from Haz-Map are copyrighted by Haz-Map(R). No part of these materials, either text or image may be used for any purpose other than for personal use. Therefore, reproduction, modification, storage in a retrieval system or retransmission, in any form or by any means, electronic, mechanical or otherwise, for reasons other than personal use, is strictly prohibited without prior written permission. | |
CAS No. |
1308-06-1, 11104-61-3, 1307-96-6 | |
Record name | Cobaltic-cobaltous oxide | |
Source | ChemIDplus | |
URL | https://pubchem.ncbi.nlm.nih.gov/substance/?source=chemidplus&sourceid=0001308061 | |
Description | ChemIDplus is a free, web search system that provides access to the structure and nomenclature authority files used for the identification of chemical substances cited in National Library of Medicine (NLM) databases, including the TOXNET system. | |
Record name | Cobalt oxide | |
Source | EPA Chemicals under the TSCA | |
URL | https://www.epa.gov/chemicals-under-tsca | |
Description | EPA Chemicals under the Toxic Substances Control Act (TSCA) collection contains information on chemicals and their regulations under TSCA, including non-confidential content from the TSCA Chemical Substance Inventory and Chemical Data Reporting. | |
Record name | Cobalt oxide (Co3O4) | |
Source | EPA Chemicals under the TSCA | |
URL | https://www.epa.gov/chemicals-under-tsca | |
Description | EPA Chemicals under the Toxic Substances Control Act (TSCA) collection contains information on chemicals and their regulations under TSCA, including non-confidential content from the TSCA Chemical Substance Inventory and Chemical Data Reporting. | |
Record name | Cobalt oxide | |
Source | European Chemicals Agency (ECHA) | |
URL | https://echa.europa.eu/substance-information/-/substanceinfo/100.013.777 | |
Description | The European Chemicals Agency (ECHA) is an agency of the European Union which is the driving force among regulatory authorities in implementing the EU's groundbreaking chemicals legislation for the benefit of human health and the environment as well as for innovation and competitiveness. | |
Explanation | Use of the information, documents and data from the ECHA website is subject to the terms and conditions of this Legal Notice, and subject to other binding limitations provided for under applicable law, the information, documents and data made available on the ECHA website may be reproduced, distributed and/or used, totally or in part, for non-commercial purposes provided that ECHA is acknowledged as the source: "Source: European Chemicals Agency, http://echa.europa.eu/". Such acknowledgement must be included in each copy of the material. ECHA permits and encourages organisations and individuals to create links to the ECHA website under the following cumulative conditions: Links can only be made to webpages that provide a link to the Legal Notice page. | |
Record name | Tricobalt tetraoxide | |
Source | European Chemicals Agency (ECHA) | |
URL | https://echa.europa.eu/substance-information/-/substanceinfo/100.013.780 | |
Description | The European Chemicals Agency (ECHA) is an agency of the European Union which is the driving force among regulatory authorities in implementing the EU's groundbreaking chemicals legislation for the benefit of human health and the environment as well as for innovation and competitiveness. | |
Explanation | Use of the information, documents and data from the ECHA website is subject to the terms and conditions of this Legal Notice, and subject to other binding limitations provided for under applicable law, the information, documents and data made available on the ECHA website may be reproduced, distributed and/or used, totally or in part, for non-commercial purposes provided that ECHA is acknowledged as the source: "Source: European Chemicals Agency, http://echa.europa.eu/". Such acknowledgement must be included in each copy of the material. ECHA permits and encourages organisations and individuals to create links to the ECHA website under the following cumulative conditions: Links can only be made to webpages that provide a link to the Legal Notice page. | |
Record name | Cobalt oxide | |
Source | European Chemicals Agency (ECHA) | |
URL | https://echa.europa.eu/substance-information/-/substanceinfo/100.031.200 | |
Description | The European Chemicals Agency (ECHA) is an agency of the European Union which is the driving force among regulatory authorities in implementing the EU's groundbreaking chemicals legislation for the benefit of human health and the environment as well as for innovation and competitiveness. | |
Explanation | Use of the information, documents and data from the ECHA website is subject to the terms and conditions of this Legal Notice, and subject to other binding limitations provided for under applicable law, the information, documents and data made available on the ECHA website may be reproduced, distributed and/or used, totally or in part, for non-commercial purposes provided that ECHA is acknowledged as the source: "Source: European Chemicals Agency, http://echa.europa.eu/". Such acknowledgement must be included in each copy of the material. ECHA permits and encourages organisations and individuals to create links to the ECHA website under the following cumulative conditions: Links can only be made to webpages that provide a link to the Legal Notice page. | |
Record name | COBALT OXIDE | |
Source | FDA Global Substance Registration System (GSRS) | |
URL | https://gsrs.ncats.nih.gov/ginas/app/beta/substances/USK772NS56 | |
Description | The FDA Global Substance Registration System (GSRS) enables the efficient and accurate exchange of information on what substances are in regulated products. Instead of relying on names, which vary across regulatory domains, countries, and regions, the GSRS knowledge base makes it possible for substances to be defined by standardized, scientific descriptions. | |
Explanation | Unless otherwise noted, the contents of the FDA website (www.fda.gov), both text and graphics, are not copyrighted. They are in the public domain and may be republished, reprinted and otherwise used freely by anyone without the need to obtain permission from FDA. Credit to the U.S. Food and Drug Administration as the source is appreciated but not required. | |
Synthesis routes and methods I
Procedure details
Synthesis routes and methods II
Procedure details
Synthesis routes and methods III
Procedure details
Synthesis routes and methods IV
Procedure details
Haftungsausschluss und Informationen zu In-Vitro-Forschungsprodukten
Bitte beachten Sie, dass alle Artikel und Produktinformationen, die auf BenchChem präsentiert werden, ausschließlich zu Informationszwecken bestimmt sind. Die auf BenchChem zum Kauf angebotenen Produkte sind speziell für In-vitro-Studien konzipiert, die außerhalb lebender Organismen durchgeführt werden. In-vitro-Studien, abgeleitet von dem lateinischen Begriff "in Glas", beinhalten Experimente, die in kontrollierten Laborumgebungen unter Verwendung von Zellen oder Geweben durchgeführt werden. Es ist wichtig zu beachten, dass diese Produkte nicht als Arzneimittel oder Medikamente eingestuft sind und keine Zulassung der FDA für die Vorbeugung, Behandlung oder Heilung von medizinischen Zuständen, Beschwerden oder Krankheiten erhalten haben. Wir müssen betonen, dass jede Form der körperlichen Einführung dieser Produkte in Menschen oder Tiere gesetzlich strikt untersagt ist. Es ist unerlässlich, sich an diese Richtlinien zu halten, um die Einhaltung rechtlicher und ethischer Standards in Forschung und Experiment zu gewährleisten.