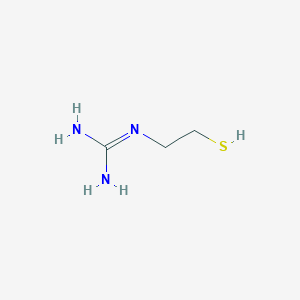
2-Mercaptoethylguanidine
Übersicht
Beschreibung
2-Mercaptoethylguanidine is an organic compound that belongs to the class of guanidines. It is characterized by the presence of a thiol group (-SH) and a guanidine group.
Vorbereitungsmethoden
Synthetic Routes and Reaction Conditions: The synthesis of 2-Mercaptoethylguanidine typically involves the reaction of thiourea with ethylenediamine under specific conditions. The reaction proceeds through the formation of an intermediate, which is then treated with a guanidylating agent to yield the final product .
Industrial Production Methods: Industrial production of this compound often employs similar synthetic routes but on a larger scale. The process involves careful control of reaction conditions, such as temperature and pH, to ensure high yield and purity of the product .
Analyse Chemischer Reaktionen
Types of Reactions: 2-Mercaptoethylguanidine undergoes various chemical reactions, including:
Oxidation: The thiol group can be oxidized to form disulfides.
Reduction: The guanidine group can be reduced under specific conditions.
Substitution: The thiol group can participate in nucleophilic substitution reactions
Common Reagents and Conditions:
Oxidation: Hydrogen peroxide or other oxidizing agents.
Reduction: Reducing agents such as sodium borohydride.
Substitution: Alkyl halides or other electrophiles
Major Products:
Oxidation: Disulfides.
Reduction: Reduced guanidine derivatives.
Substitution: Substituted thiol derivatives
Wissenschaftliche Forschungsanwendungen
Inhibition of Nitric Oxide Synthase
MEG has been identified as a potent inhibitor of inducible nitric oxide synthase (iNOS), which is crucial in the production of nitric oxide (NO) during inflammatory responses. Research indicates that MEG reduces inflammation by inhibiting iNOS activity, thereby decreasing NO levels in various models of inflammation.
- Mechanism of Action : MEG acts by preventing the interaction between iNOS and its substrate, leading to a reduction in NO synthesis. This inhibition is particularly relevant in conditions characterized by excessive NO production, which can contribute to tissue damage and inflammation .
- Case Studies : In a study involving bacterial meningitis in rabbits, MEG demonstrated significant anti-inflammatory effects by scavenging peroxynitrite and inhibiting iNOS, leading to improved clinical outcomes .
Scavenging of Peroxynitrite
Peroxynitrite is a reactive nitrogen species formed from the reaction of NO with superoxide. It plays a critical role in oxidative stress and cellular damage. MEG has been shown to effectively scavenge peroxynitrite, thereby mitigating its harmful effects.
- Kinetic Studies : MEG reacts with peroxynitrite with a second-order rate constant of approximately 1900 M⁻¹ s⁻¹ at 37°C, indicating its efficiency as a scavenger . This reaction helps protect cells from oxidative damage caused by peroxynitrite.
- Research Findings : In cultured macrophages, MEG was found to reduce mitochondrial dysfunction and DNA damage induced by peroxynitrite exposure. This protective effect underscores the potential of MEG as a therapeutic agent in conditions associated with oxidative stress .
Protective Effects Against Radiation
MEG has been investigated for its protective effects against radiation-induced damage. Studies have shown that MEG can enhance recovery from radiation exposure without reducing initial damage levels.
- Mechanism : The protective action of MEG appears to involve its ability to scavenge free radicals generated during radiation exposure, thereby reducing cellular injury and promoting recovery processes .
- Experimental Evidence : In experimental models, mice treated with MEG exhibited faster recovery rates post-radiation exposure compared to untreated controls, suggesting its potential use in radioprotection strategies .
Applications in Periodontal Disease
Recent studies have indicated that MEG may have beneficial effects in periodontal diseases characterized by inflammation and oxidative stress.
- Research Insights : Evidence suggests that MEG can inhibit iNOS expression in models of periodontitis, leading to reduced inflammatory responses and improved periodontal health outcomes .
Summary Table of Applications
Application | Mechanism | Research Findings |
---|---|---|
Inhibition of iNOS | Prevents NO production | Reduced inflammation in bacterial meningitis models |
Scavenging Peroxynitrite | Reacts with peroxynitrite | Decreased mitochondrial dysfunction in macrophages |
Radiation Protection | Scavenges free radicals | Enhanced recovery post-radiation exposure |
Periodontal Disease Treatment | Inhibits iNOS expression | Improved outcomes in experimental periodontitis models |
Wirkmechanismus
The mechanism of action of 2-Mercaptoethylguanidine involves its interaction with free radicals and reactive oxygen species. The thiol group can scavenge these reactive species, thereby protecting cells from oxidative damage. Additionally, the guanidine group can interact with various molecular targets, modulating their activity and providing protective effects .
Vergleich Mit ähnlichen Verbindungen
- 2-Aminoethylguanidine
- 2-Mercaptoethylamine
- 2-Guanidinoethanol
Comparison: 2-Mercaptoethylguanidine is unique due to the presence of both a thiol and a guanidine group, which confer distinct chemical reactivity and biological activity. Compared to 2-Aminoethylguanidine, it has enhanced radioprotective properties due to the thiol group. Compared to 2-Mercaptoethylamine, it has additional biological activity due to the guanidine group .
Biologische Aktivität
2-Mercaptoethylguanidine (MEG) is a thiol-containing compound known for its diverse biological activities, particularly in the context of inflammation and oxidative stress. This article explores the biological activity of MEG, focusing on its mechanisms of action, therapeutic potential, and relevant case studies.
MEG exhibits several mechanisms through which it exerts its biological effects:
- Nitric Oxide Synthase Inhibition : MEG acts as an inhibitor of inducible nitric oxide synthase (iNOS), which is crucial in inflammatory responses. By inhibiting iNOS, MEG can reduce the production of nitric oxide, a mediator involved in various pathophysiological conditions .
- Scavenging of Peroxynitrite : MEG is a potent scavenger of peroxynitrite, a reactive nitrogen species formed from nitric oxide and superoxide. It has a second-order rate constant of , indicating its effectiveness in neutralizing this harmful species .
- Anti-inflammatory Properties : Studies have demonstrated that MEG can decrease the inactivation of α1-antiproteinase by hypochlorous acid, suggesting a protective role against oxidative damage in inflammatory conditions . Additionally, it has shown efficacy in animal models for conditions like shock and arthritis .
Table 1: Summary of Biological Activities of this compound
Case Studies and Experimental Evidence
- Inflammation Models : In a study evaluating the anti-inflammatory potential of MEG, researchers used carrageenan-induced paw edema models. MEG treatment significantly reduced edema compared to control groups, indicating its efficacy in managing acute inflammation .
- Cell Proliferation Studies : The effects of MEG on HeLa S3 cells were investigated to assess its impact on cell viability and proliferation. Results indicated that MEG could influence cell growth dynamics, with implications for cancer research and therapeutic applications .
- Hemorrhagic Shock Studies : In experimental models simulating hemorrhagic shock, MEG administration improved tissue perfusion and reduced mortality rates. This suggests its potential utility in critical care settings where oxidative stress and inflammation are prevalent .
Eigenschaften
IUPAC Name |
2-(2-sulfanylethyl)guanidine | |
---|---|---|
Source | PubChem | |
URL | https://pubchem.ncbi.nlm.nih.gov | |
Description | Data deposited in or computed by PubChem | |
InChI |
InChI=1S/C3H9N3S/c4-3(5)6-1-2-7/h7H,1-2H2,(H4,4,5,6) | |
Source | PubChem | |
URL | https://pubchem.ncbi.nlm.nih.gov | |
Description | Data deposited in or computed by PubChem | |
InChI Key |
GAPFINWZKMCSBG-UHFFFAOYSA-N | |
Source | PubChem | |
URL | https://pubchem.ncbi.nlm.nih.gov | |
Description | Data deposited in or computed by PubChem | |
Canonical SMILES |
C(CS)N=C(N)N | |
Source | PubChem | |
URL | https://pubchem.ncbi.nlm.nih.gov | |
Description | Data deposited in or computed by PubChem | |
Molecular Formula |
C3H9N3S | |
Source | PubChem | |
URL | https://pubchem.ncbi.nlm.nih.gov | |
Description | Data deposited in or computed by PubChem | |
Related CAS |
19767-44-3 (mono-hydrochloride), 4337-69-3 (mono-hydrobromide) | |
Record name | 2-Mercaptoethylguanidine | |
Source | ChemIDplus | |
URL | https://pubchem.ncbi.nlm.nih.gov/substance/?source=chemidplus&sourceid=0001190745 | |
Description | ChemIDplus is a free, web search system that provides access to the structure and nomenclature authority files used for the identification of chemical substances cited in National Library of Medicine (NLM) databases, including the TOXNET system. | |
DSSTOX Substance ID |
DTXSID00152290 | |
Record name | 2-Mercaptoethylguanidine | |
Source | EPA DSSTox | |
URL | https://comptox.epa.gov/dashboard/DTXSID00152290 | |
Description | DSSTox provides a high quality public chemistry resource for supporting improved predictive toxicology. | |
Molecular Weight |
119.19 g/mol | |
Source | PubChem | |
URL | https://pubchem.ncbi.nlm.nih.gov | |
Description | Data deposited in or computed by PubChem | |
CAS No. |
1190-74-5 | |
Record name | (Mercaptoethyl)guanidine | |
Source | CAS Common Chemistry | |
URL | https://commonchemistry.cas.org/detail?cas_rn=1190-74-5 | |
Description | CAS Common Chemistry is an open community resource for accessing chemical information. Nearly 500,000 chemical substances from CAS REGISTRY cover areas of community interest, including common and frequently regulated chemicals, and those relevant to high school and undergraduate chemistry classes. This chemical information, curated by our expert scientists, is provided in alignment with our mission as a division of the American Chemical Society. | |
Explanation | The data from CAS Common Chemistry is provided under a CC-BY-NC 4.0 license, unless otherwise stated. | |
Record name | 2-Mercaptoethylguanidine | |
Source | ChemIDplus | |
URL | https://pubchem.ncbi.nlm.nih.gov/substance/?source=chemidplus&sourceid=0001190745 | |
Description | ChemIDplus is a free, web search system that provides access to the structure and nomenclature authority files used for the identification of chemical substances cited in National Library of Medicine (NLM) databases, including the TOXNET system. | |
Record name | 2-Mercaptoethylguanidine | |
Source | EPA DSSTox | |
URL | https://comptox.epa.gov/dashboard/DTXSID00152290 | |
Description | DSSTox provides a high quality public chemistry resource for supporting improved predictive toxicology. | |
Record name | 2-MERCAPTOETHYLGUANIDINE | |
Source | FDA Global Substance Registration System (GSRS) | |
URL | https://gsrs.ncats.nih.gov/ginas/app/beta/substances/S33ZNG2R5Y | |
Description | The FDA Global Substance Registration System (GSRS) enables the efficient and accurate exchange of information on what substances are in regulated products. Instead of relying on names, which vary across regulatory domains, countries, and regions, the GSRS knowledge base makes it possible for substances to be defined by standardized, scientific descriptions. | |
Explanation | Unless otherwise noted, the contents of the FDA website (www.fda.gov), both text and graphics, are not copyrighted. They are in the public domain and may be republished, reprinted and otherwise used freely by anyone without the need to obtain permission from FDA. Credit to the U.S. Food and Drug Administration as the source is appreciated but not required. | |
Retrosynthesis Analysis
AI-Powered Synthesis Planning: Our tool employs the Template_relevance Pistachio, Template_relevance Bkms_metabolic, Template_relevance Pistachio_ringbreaker, Template_relevance Reaxys, Template_relevance Reaxys_biocatalysis model, leveraging a vast database of chemical reactions to predict feasible synthetic routes.
One-Step Synthesis Focus: Specifically designed for one-step synthesis, it provides concise and direct routes for your target compounds, streamlining the synthesis process.
Accurate Predictions: Utilizing the extensive PISTACHIO, BKMS_METABOLIC, PISTACHIO_RINGBREAKER, REAXYS, REAXYS_BIOCATALYSIS database, our tool offers high-accuracy predictions, reflecting the latest in chemical research and data.
Strategy Settings
Precursor scoring | Relevance Heuristic |
---|---|
Min. plausibility | 0.01 |
Model | Template_relevance |
Template Set | Pistachio/Bkms_metabolic/Pistachio_ringbreaker/Reaxys/Reaxys_biocatalysis |
Top-N result to add to graph | 6 |
Feasible Synthetic Routes
Q1: What is 2-Mercaptoethylguanidine (MEG) primarily known for in these research papers?
A1: MEG is primarily studied for its radioprotective properties. [, , , , , , , , ] It has shown efficacy in protecting mice from the lethal effects of X-ray radiation. []
Q2: What are the potential mechanisms of action of MEG in radiation protection?
A2: Although the exact mechanism remains unclear, research suggests that MEG might exert its radioprotective effect through multiple pathways:
- Free Radical Scavenging: MEG and GED can react with oxidizing free radicals generated by ionizing radiation in aqueous solutions, suggesting a potential free radical scavenging mechanism. []
- Protein Binding: A significant portion of MEG in treated animals is found bound to proteins, primarily through mixed disulfide bonds with protein sulfhydryl groups. [, , , ] This protein binding may contribute to the protective effect.
- Dose Reduction: MEG may act as a "dose-reducing" agent, effectively lowering the radiation dose experienced by the organism. []
Q3: What are the limitations of MEG as a radioprotector?
A3: MEG does not offer universal protection against all radiation damage. High doses of radiation still prove lethal even with MEG pretreatment. [] Additionally, it may not be effective against fractionated or chronic radiation exposures. []
Q4: What is the chemical structure of this compound (MEG)?
A4: this compound contains a thiol (-SH) group, a guanidine group, and an ethyl bridge connecting them.
Q5: Does MEG interact with proteins?
A5: Yes, MEG readily binds to proteins in solution. This interaction is thought to occur through mixed disulfide bond formation with protein sulfhydryl groups and through hydrogen bonding between the guanidine group of MEG and carboxylate and hydroxyl groups on the protein. [, ]
Q6: How does radiation affect the binding of MEG to proteins?
A6: Irradiation with Cobalt-60 gamma rays increases the amount of MEG bound to proteins. This observation contradicts the theory that radiation protection arises from the splitting of the disulfide bond between MEG and proteins. []
Q7: Does MEG interact with enzymes?
A7: MEG has been shown to protect various enzymes, including yeast enolase, rabbit muscle enolase, and lactic dehydrogenase, from ionizing radiation. [] Additionally, it has been found to inhibit dopamine-beta-hydroxylase and mucopolysaccharide sulfation. [, ]
Q8: Does MEG affect the uptake of iodine by the thyroid gland?
A8: Research indicates that both MEG and its precursor, AET (S-(2-aminoethyl)thiuronium bromide hydrobromide), can affect the uptake of radioactive iodine (I-131) by the thyroid gland in rats. []
Q9: What are the main metabolites of MEG observed in mice?
A9: Following administration of MEG or GED, various metabolites have been identified in mice tissues, including:
- Protein-bound MEG (primarily in mixed disulfide linkage) [, ]
- GED [, ]
- Taurocyamine [, , ]
- Guanidoethanesulfinic acid [, , ]
- S-acetyl-2-mercaptoethylguanidine []
- Sulfate [, , ]
Q10: Does the presence of a tumor affect MEG metabolism?
A10: Yes, the presence of tumors seems to impact MEG metabolism and excretion. Tumor-bearing mice exhibit difficulty excreting MEG compared to healthy mice. [, ] Additionally, tumor-bearing mice show a higher concentration of MEG in the kidneys compared to their healthy counterparts. []
Q11: How does the tissue distribution of MEG compare to that of GED?
A11: Both MEG and GED exhibit different tissue distribution patterns. MEG is absorbed faster and achieves higher concentrations of protective forms in tissues compared to GED. [, ] The brain, subcutaneous mammary tumors, and testes show the lowest concentrations of AET sulfur, while the kidney, small intestines, and liver show the highest. []
Haftungsausschluss und Informationen zu In-Vitro-Forschungsprodukten
Bitte beachten Sie, dass alle Artikel und Produktinformationen, die auf BenchChem präsentiert werden, ausschließlich zu Informationszwecken bestimmt sind. Die auf BenchChem zum Kauf angebotenen Produkte sind speziell für In-vitro-Studien konzipiert, die außerhalb lebender Organismen durchgeführt werden. In-vitro-Studien, abgeleitet von dem lateinischen Begriff "in Glas", beinhalten Experimente, die in kontrollierten Laborumgebungen unter Verwendung von Zellen oder Geweben durchgeführt werden. Es ist wichtig zu beachten, dass diese Produkte nicht als Arzneimittel oder Medikamente eingestuft sind und keine Zulassung der FDA für die Vorbeugung, Behandlung oder Heilung von medizinischen Zuständen, Beschwerden oder Krankheiten erhalten haben. Wir müssen betonen, dass jede Form der körperlichen Einführung dieser Produkte in Menschen oder Tiere gesetzlich strikt untersagt ist. Es ist unerlässlich, sich an diese Richtlinien zu halten, um die Einhaltung rechtlicher und ethischer Standards in Forschung und Experiment zu gewährleisten.