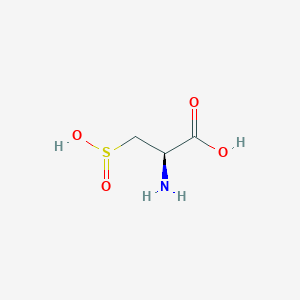
L-Cysteinsulfinsäure
Übersicht
Beschreibung
L-Cysteinesulfinic Acid is an organic compound with the nominal formula HO₂SCH₂CH(NH₂)CO₂H. It is a rare example of an amino acid bearing a sulfinic acid functional group. This compound is a white solid that is soluble in water and exists as the zwitterion at neutral pH. It is chiral, and only the L-enantiomer occurs in nature. L-Cysteinesulfinic Acid is an intermediate in cysteine metabolism and is not a coded amino acid but is produced post-translationally .
Wissenschaftliche Forschungsanwendungen
L-Cysteinsulfinsäure hat mehrere wissenschaftliche Forschungsanwendungen in verschiedenen Bereichen:
Chemie:
- Wird als Reagenz in der organischen Synthese verwendet, um die Sulfinsäure- funktionelle Gruppe in Moleküle einzubringen.
Biologie:
- Dient als Zwischenprodukt im Stoffwechselweg von Cystein und führt zur Produktion von Taurin, einer Verbindung, die für die Gallensäurebildung und die Neurotransmission wichtig ist .
Medizin:
- Wird wegen seiner potenziellen Rolle bei der Modulation der Neurotransmitteraktivität untersucht, da es als Agonist an metabotropen Glutamatrezeptoren wirken kann .
Industrie:
5. Wirkmechanismus
This compound übt seine Wirkungen hauptsächlich durch seine Rolle als Zwischenprodukt im Cystein-Stoffwechsel aus. Es wird durch das Enzym Sulfinoalanin-Decarboxylase zu Hypotaurin umgewandelt, und Hypotaurin wird anschließend zu Taurin oxidiert. Taurin ist an verschiedenen physiologischen Prozessen beteiligt, darunter die Gallensäurebildung, die Osmoregulation und die Neurotransmission .
Molekuläre Zielstrukturen und -wege:
Metabotrope Glutamatrezeptoren: this compound wirkt als Agonist an mehreren metabotropen Glutamatrezeptoren und beeinflusst die Neurotransmitterfreisetzung und die synaptische Plastizität.
Stoffwechselweg von Cystein: Es ist ein wichtiges Zwischenprodukt im Weg, der zur Produktion von Taurin führt.
Wirkmechanismus
Target of Action
L-Cysteinesulfinic acid (CSA) is a potent agonist at several rat metabotropic glutamate receptors (mGluRs), specifically mGluR1, mGluR5, mGluR2, mGluR4, mGluR6, and mGluR8 . These receptors play a crucial role in the central nervous system, mediating various physiological functions such as learning, memory, and motor control.
Mode of Action
CSA interacts with its targets, the mGluRs, by binding to these receptors and activating them . This interaction leads to the stimulation of phospholipase D (PLD) activity . The activation of PLD then triggers a series of downstream signaling events, leading to various cellular responses.
Biochemical Pathways
CSA is synthesized from L-cysteine by the enzyme cysteine dioxygenase (CDO) . This conversion involves the oxidation of sulfur to sulfinic acid. CSA is a putative excitatory amino acid (EAA) with neurotransmitter functionality . It is involved in the regulation of cardiovascular functions and is an intermediate in cysteine metabolism .
Pharmacokinetics
It is known that csa is synthesized endogenously from l-cysteine, suggesting that its bioavailability is likely regulated by the availability of l-cysteine and the activity of the enzyme cdo .
Result of Action
The activation of mGluRs by CSA leads to a variety of cellular responses. For example, CSA has been shown to decrease mean arterial blood pressure and heart rate in rats when microinjected into the nucleus tractus solitarius (NTS) . This suggests that CSA may play a role in the regulation of cardiovascular functions.
Action Environment
The action of CSA is likely influenced by various environmental factors. For instance, the activity of the enzyme CDO, which is responsible for the synthesis of CSA from L-cysteine, can be regulated by various factors, including the availability of L-cysteine and the presence of other molecules that can interact with CDO
Biochemische Analyse
Biochemical Properties
L-Cysteinesulfinic acid is a potent agonist at several rat metabotropic glutamate receptors (mGluRs) with pEC50s of 3.92, 4.6, 3.9, 2.7, 4.0, and 3.94 for mGluR1, mGluR5, mGluR2, mGluR4, mGluR6, and mGluR8, respectively . It interacts with these enzymes and proteins, influencing their function and playing a significant role in biochemical reactions .
Cellular Effects
L-Cysteinesulfinic acid influences cell function by impacting cell signaling pathways, gene expression, and cellular metabolism . It increases intracellular inositol phosphate levels in CHO cells expressing mGluR1, mGluR5, or mGluR8 and inhibits forskolin-induced cAMP production in CHO cells expressing mGluR2 or mGluR6 and hamster kidney cells expressing mGluR4 .
Molecular Mechanism
At the molecular level, L-Cysteinesulfinic acid exerts its effects through binding interactions with biomolecules, enzyme inhibition or activation, and changes in gene expression . It selectively binds to mGluR1α over adrenergic, dopamine, histamine, muscarinic, nicotinic, or serotonin receptors .
Temporal Effects in Laboratory Settings
The effects of L-Cysteinesulfinic acid change over time in laboratory settings . Information on the product’s stability, degradation, and any long-term effects on cellular function observed in in vitro or in vivo studies is currently limited.
Dosage Effects in Animal Models
The effects of L-Cysteinesulfinic acid vary with different dosages in animal models . Specific information on threshold effects observed in these studies, as well as any toxic or adverse effects at high doses, is currently limited.
Metabolic Pathways
L-Cysteinesulfinic acid is involved in metabolic pathways, interacting with enzymes or cofactors . Specific information on its effects on metabolic flux or metabolite levels is currently limited.
Transport and Distribution
L-Cysteinesulfinic acid is transported and distributed within cells and tissues . Specific information on any transporters or binding proteins that it interacts with, as well as any effects on its localization or accumulation, is currently limited.
Vorbereitungsmethoden
Synthetische Wege und Reaktionsbedingungen: L-Cysteinsulfinsäure kann durch Oxidation von L-Cystein synthetisiert werden. Das Enzym Cystein-Dioxygenase katalysiert die Umwandlung von L-Cystein zu this compound. Diese Reaktion beinhaltet die Einlagerung von molekularem Sauerstoff in das Schwefelatom von Cystein, wodurch die Sulfinsäuregruppe gebildet wird .
Industrielle Produktionsmethoden: Die industrielle Produktion von this compound erfolgt typischerweise durch chemische Oxidation von L-Cystein unter Verwendung von Oxidationsmitteln wie Wasserstoffperoxid oder Persäuren. Die Reaktion wird unter kontrollierten Bedingungen durchgeführt, um die selektive Bildung der Sulfinsäuregruppe zu gewährleisten, ohne dass es zu einer Überoxidation zur Sulfonsäure kommt .
Analyse Chemischer Reaktionen
Arten von Reaktionen: L-Cysteinsulfinsäure unterliegt verschiedenen chemischen Reaktionen, darunter Oxidations-, Reduktions- und Substitutionsreaktionen.
Häufige Reagenzien und Bedingungen:
Oxidation: this compound kann mit starken Oxidationsmitteln wie Wasserstoffperoxid weiter zu Cysteinsäure oxidiert werden.
Reduktion: Sie kann mit Reduktionsmitteln wie Natriumborhydrid wieder zu L-Cystein reduziert werden.
Substitution: Die Sulfinsäuregruppe kann an nukleophilen Substitutionsreaktionen teilnehmen und verschiedene Derivate bilden.
Hauptprodukte:
Oxidation: Cysteinsäure
Reduktion: L-Cystein
Substitution: Verschiedene Sulfinsäurederivate.
Vergleich Mit ähnlichen Verbindungen
L-Cysteinsulfinsäure kann mit anderen schwefelhaltigen Aminosäuren wie L-Cystein, Cysteinsäure und Taurin verglichen werden.
Ähnliche Verbindungen:
L-Cystein: Eine Vorstufe von this compound, die an der Proteinsynthese und Entgiftungsprozessen beteiligt ist.
Cysteinsäure: Ein Oxidationsprodukt von this compound, das an verschiedenen Stoffwechselwegen beteiligt ist.
Einzigartigkeit: this compound ist aufgrund ihrer Sulfinsäure- funktionellen Gruppe einzigartig, die es ihr ermöglicht, an spezifischen chemischen Reaktionen und Stoffwechselwegen teilzunehmen, die sich von denen anderer schwefelhaltiger Aminosäuren unterscheiden .
Biologische Aktivität
L-Cysteinesulfinic acid (L-CSA) is a naturally occurring amino acid derivative that plays a significant role in various biological processes, particularly in the central nervous system and metabolic pathways. This article explores the biological activity of L-CSA, highlighting its mechanisms of action, physiological roles, and potential therapeutic applications based on diverse research findings.
Overview of L-Cysteinesulfinic Acid
L-Cysteinesulfinic acid is formed from the oxidation of L-cysteine and serves as a precursor to taurine, an important amino acid involved in numerous physiological functions. The enzymatic conversion of L-CSA to taurine is facilitated by cysteine sulfinic acid decarboxylase (CSAD), which has been the subject of extensive biochemical studies.
Neurotransmitter Role
Research indicates that L-CSA acts as an endogenous neurotransmitter . It has been shown to activate specific receptors in the adult rat hippocampus that are insensitive to other excitatory amino acids like L-glutamate and L-aspartate. This unique receptor activation leads to an increase in phospholipase D (PLD) activity, suggesting that L-CSA may play a role in synaptic transmission and plasticity .
Decarboxylation to Taurine
The decarboxylation of L-CSA by CSAD produces taurine, which is crucial for various biological functions, including osmoregulation and neuromodulation. Studies have demonstrated that the activity of CSAD can be influenced by several factors, including substrate concentration and environmental conditions . The kinetics of this reaction have been characterized, revealing a maximum turnover rate () of approximately 5.6 s under optimal conditions .
Antioxidant Properties
L-Cysteinesulfinic acid exhibits antioxidant properties , which may contribute to its neuroprotective effects. It has been implicated in reducing oxidative stress in neural tissues, potentially offering therapeutic benefits for neurodegenerative diseases .
Antimicrobial Activity
Recent studies have explored the antimicrobial potential of L-CSA. In vitro experiments indicated that while L-CSA itself did not exhibit significant antimicrobial properties compared to other compounds like α-lipoic acid, it may still play a role in modulating microbial populations in specific environments .
Case Studies and Research Findings
- Neonatal Phenylketonuria (PKU) : A study observed elevated levels of L-CSA in the hair roots of neonates with PKU, which normalized after dietary control was implemented. This suggests a potential biomarker role for L-CSA in metabolic disorders .
- Cystein Metabolism : Research has shown that alterations in cysteine metabolism involving L-CSA can affect taurine biosynthesis pathways, impacting various physiological functions including neurotransmission and muscle contraction .
- In Vitro Studies : In vitro assays demonstrated that varying concentrations of L-CSA influenced the growth rates of pathogenic bacteria such as E. coli O157:H7 and Salmonella enterica, indicating its potential utility in food safety applications .
Table 1: Effects of Various Compounds on Bacterial Growth Rates
Compound | Concentration (mM) | E. coli Growth Rate (h) | Salmonella Growth Rate (h) |
---|---|---|---|
Ethyl Nitroacetate | 3 | 0.823 | 0.886 |
Taurine | 3 | 0.527 | 0.656 |
α-Lipoic Acid | 3 | 0.740 | 0.920 |
L-Cysteinesulfinic Acid | 3 | 0.224 | 0.195 |
Eigenschaften
IUPAC Name |
(2R)-2-amino-3-sulfinopropanoic acid | |
---|---|---|
Source | PubChem | |
URL | https://pubchem.ncbi.nlm.nih.gov | |
Description | Data deposited in or computed by PubChem | |
InChI |
InChI=1S/C3H7NO4S/c4-2(3(5)6)1-9(7)8/h2H,1,4H2,(H,5,6)(H,7,8)/t2-/m0/s1 | |
Source | PubChem | |
URL | https://pubchem.ncbi.nlm.nih.gov | |
Description | Data deposited in or computed by PubChem | |
InChI Key |
ADVPTQAUNPRNPO-REOHCLBHSA-N | |
Source | PubChem | |
URL | https://pubchem.ncbi.nlm.nih.gov | |
Description | Data deposited in or computed by PubChem | |
Canonical SMILES |
C(C(C(=O)O)N)S(=O)O | |
Source | PubChem | |
URL | https://pubchem.ncbi.nlm.nih.gov | |
Description | Data deposited in or computed by PubChem | |
Isomeric SMILES |
C([C@@H](C(=O)O)N)S(=O)O | |
Source | PubChem | |
URL | https://pubchem.ncbi.nlm.nih.gov | |
Description | Data deposited in or computed by PubChem | |
Molecular Formula |
C3H7NO4S | |
Source | PubChem | |
URL | https://pubchem.ncbi.nlm.nih.gov | |
Description | Data deposited in or computed by PubChem | |
DSSTOX Substance ID |
DTXSID20862546 | |
Record name | L-Cysteinesulfinic acid | |
Source | EPA DSSTox | |
URL | https://comptox.epa.gov/dashboard/DTXSID20862546 | |
Description | DSSTox provides a high quality public chemistry resource for supporting improved predictive toxicology. | |
Molecular Weight |
153.16 g/mol | |
Source | PubChem | |
URL | https://pubchem.ncbi.nlm.nih.gov | |
Description | Data deposited in or computed by PubChem | |
Physical Description |
Solid | |
Record name | 3-Sulfinoalanine | |
Source | Human Metabolome Database (HMDB) | |
URL | http://www.hmdb.ca/metabolites/HMDB0000996 | |
Description | The Human Metabolome Database (HMDB) is a freely available electronic database containing detailed information about small molecule metabolites found in the human body. | |
Explanation | HMDB is offered to the public as a freely available resource. Use and re-distribution of the data, in whole or in part, for commercial purposes requires explicit permission of the authors and explicit acknowledgment of the source material (HMDB) and the original publication (see the HMDB citing page). We ask that users who download significant portions of the database cite the HMDB paper in any resulting publications. | |
CAS No. |
1115-65-7 | |
Record name | L-Cysteinesulfinic acid | |
Source | CAS Common Chemistry | |
URL | https://commonchemistry.cas.org/detail?cas_rn=1115-65-7 | |
Description | CAS Common Chemistry is an open community resource for accessing chemical information. Nearly 500,000 chemical substances from CAS REGISTRY cover areas of community interest, including common and frequently regulated chemicals, and those relevant to high school and undergraduate chemistry classes. This chemical information, curated by our expert scientists, is provided in alignment with our mission as a division of the American Chemical Society. | |
Explanation | The data from CAS Common Chemistry is provided under a CC-BY-NC 4.0 license, unless otherwise stated. | |
Record name | Cysteinesulfinic acid, L- | |
Source | ChemIDplus | |
URL | https://pubchem.ncbi.nlm.nih.gov/substance/?source=chemidplus&sourceid=0001115657 | |
Description | ChemIDplus is a free, web search system that provides access to the structure and nomenclature authority files used for the identification of chemical substances cited in National Library of Medicine (NLM) databases, including the TOXNET system. | |
Record name | 3-sulfino-L-alanine | |
Source | DrugBank | |
URL | https://www.drugbank.ca/drugs/DB02153 | |
Description | The DrugBank database is a unique bioinformatics and cheminformatics resource that combines detailed drug (i.e. chemical, pharmacological and pharmaceutical) data with comprehensive drug target (i.e. sequence, structure, and pathway) information. | |
Explanation | Creative Common's Attribution-NonCommercial 4.0 International License (http://creativecommons.org/licenses/by-nc/4.0/legalcode) | |
Record name | L-Cysteinesulfinic acid | |
Source | EPA DSSTox | |
URL | https://comptox.epa.gov/dashboard/DTXSID20862546 | |
Description | DSSTox provides a high quality public chemistry resource for supporting improved predictive toxicology. | |
Record name | 3-sulphino-L-alanine | |
Source | European Chemicals Agency (ECHA) | |
URL | https://echa.europa.eu/substance-information/-/substanceinfo/100.012.935 | |
Description | The European Chemicals Agency (ECHA) is an agency of the European Union which is the driving force among regulatory authorities in implementing the EU's groundbreaking chemicals legislation for the benefit of human health and the environment as well as for innovation and competitiveness. | |
Explanation | Use of the information, documents and data from the ECHA website is subject to the terms and conditions of this Legal Notice, and subject to other binding limitations provided for under applicable law, the information, documents and data made available on the ECHA website may be reproduced, distributed and/or used, totally or in part, for non-commercial purposes provided that ECHA is acknowledged as the source: "Source: European Chemicals Agency, http://echa.europa.eu/". Such acknowledgement must be included in each copy of the material. ECHA permits and encourages organisations and individuals to create links to the ECHA website under the following cumulative conditions: Links can only be made to webpages that provide a link to the Legal Notice page. | |
Record name | CYSTEINESULFINIC ACID, L- | |
Source | FDA Global Substance Registration System (GSRS) | |
URL | https://gsrs.ncats.nih.gov/ginas/app/beta/substances/56X032NVQL | |
Description | The FDA Global Substance Registration System (GSRS) enables the efficient and accurate exchange of information on what substances are in regulated products. Instead of relying on names, which vary across regulatory domains, countries, and regions, the GSRS knowledge base makes it possible for substances to be defined by standardized, scientific descriptions. | |
Explanation | Unless otherwise noted, the contents of the FDA website (www.fda.gov), both text and graphics, are not copyrighted. They are in the public domain and may be republished, reprinted and otherwise used freely by anyone without the need to obtain permission from FDA. Credit to the U.S. Food and Drug Administration as the source is appreciated but not required. | |
Record name | 3-Sulfinoalanine | |
Source | Human Metabolome Database (HMDB) | |
URL | http://www.hmdb.ca/metabolites/HMDB0000996 | |
Description | The Human Metabolome Database (HMDB) is a freely available electronic database containing detailed information about small molecule metabolites found in the human body. | |
Explanation | HMDB is offered to the public as a freely available resource. Use and re-distribution of the data, in whole or in part, for commercial purposes requires explicit permission of the authors and explicit acknowledgment of the source material (HMDB) and the original publication (see the HMDB citing page). We ask that users who download significant portions of the database cite the HMDB paper in any resulting publications. | |
Retrosynthesis Analysis
AI-Powered Synthesis Planning: Our tool employs the Template_relevance Pistachio, Template_relevance Bkms_metabolic, Template_relevance Pistachio_ringbreaker, Template_relevance Reaxys, Template_relevance Reaxys_biocatalysis model, leveraging a vast database of chemical reactions to predict feasible synthetic routes.
One-Step Synthesis Focus: Specifically designed for one-step synthesis, it provides concise and direct routes for your target compounds, streamlining the synthesis process.
Accurate Predictions: Utilizing the extensive PISTACHIO, BKMS_METABOLIC, PISTACHIO_RINGBREAKER, REAXYS, REAXYS_BIOCATALYSIS database, our tool offers high-accuracy predictions, reflecting the latest in chemical research and data.
Strategy Settings
Precursor scoring | Relevance Heuristic |
---|---|
Min. plausibility | 0.01 |
Model | Template_relevance |
Template Set | Pistachio/Bkms_metabolic/Pistachio_ringbreaker/Reaxys/Reaxys_biocatalysis |
Top-N result to add to graph | 6 |
Feasible Synthetic Routes
Haftungsausschluss und Informationen zu In-Vitro-Forschungsprodukten
Bitte beachten Sie, dass alle Artikel und Produktinformationen, die auf BenchChem präsentiert werden, ausschließlich zu Informationszwecken bestimmt sind. Die auf BenchChem zum Kauf angebotenen Produkte sind speziell für In-vitro-Studien konzipiert, die außerhalb lebender Organismen durchgeführt werden. In-vitro-Studien, abgeleitet von dem lateinischen Begriff "in Glas", beinhalten Experimente, die in kontrollierten Laborumgebungen unter Verwendung von Zellen oder Geweben durchgeführt werden. Es ist wichtig zu beachten, dass diese Produkte nicht als Arzneimittel oder Medikamente eingestuft sind und keine Zulassung der FDA für die Vorbeugung, Behandlung oder Heilung von medizinischen Zuständen, Beschwerden oder Krankheiten erhalten haben. Wir müssen betonen, dass jede Form der körperlichen Einführung dieser Produkte in Menschen oder Tiere gesetzlich strikt untersagt ist. Es ist unerlässlich, sich an diese Richtlinien zu halten, um die Einhaltung rechtlicher und ethischer Standards in Forschung und Experiment zu gewährleisten.