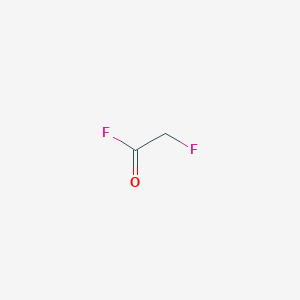
Fluoroacetyl fluoride
Ăbersicht
Beschreibung
Fluoroacetyl fluoride (CâHâFâO) is a fluorinated acyl halide characterized by the presence of two fluorine atoms: one in the acetyl group (CHâFâ) and another as the terminal fluoride (âCOF). Its molecular structure, determined via microwave spectroscopy and gas-phase electron diffraction, exhibits rotational isomerism due to the spatial arrangement of the fluorine atoms . The compound is synthesized through fluorination of acetyl derivatives or via acylation reactions using fluorinated reagents, as evidenced in studies involving fluoroacetyl chloride as a precursor .
Vorbereitungsmethoden
Synthetic Routes and Reaction Conditions: Fluoroacetyl fluoride can be synthesized through several methods. One common method involves the reaction of fluoroacetic acid with thionyl chloride, resulting in the formation of fluoroacetyl chloride, which is then treated with hydrogen fluoride to produce this compound . Another method involves the direct fluorination of acetyl fluoride using elemental fluorine under controlled conditions .
Industrial Production Methods: In industrial settings, this compound is typically produced through the fluorination of acetyl fluoride using specialized fluorinating agents such as sulfur tetrafluoride or cobalt trifluoride . These methods are preferred due to their efficiency and scalability, allowing for the large-scale production of this compound.
Analyse Chemischer Reaktionen
Hydrolysis Reactions
Fluoroacetyl fluoride undergoes hydrolysis, a key reaction influenced by enzymatic and non-enzymatic pathways:
Enzymatic Hydrolysis by FlK Thioesterase
-
Mechanism : The thioesterase FlK from Streptomyces cattleya selectively hydrolyzes fluoroacetyl-CoA to fluoroacetate and CoA, protecting the host from fluoroacetate toxicity .
-
Kinetic Specificity :
Non-Enzymatic Hydrolysis
-
In aqueous solutions, this compound hydrolyzes to fluoroacetic acid and HF :
-
The reaction rate is pH-dependent, with faster hydrolysis under alkaline conditions due to increased nucleophilicity of water .
Reactions in Superacidic Media
In HF/SbFâ superacid systems, this compound exhibits unique equilibria:
Protonation and Rearrangement
-
Observation : NMR studies reveal an equilibrium between this compound and a protonated α-fluoroalcohol species :
-
Key Factors :
Fluorination and Halogen Exchange
This compound participates in halogen-exchange reactions under catalytic conditions:
Comparative Reactivity with Acetyl Fluoride
This compoundâs reactivity diverges from acetyl fluoride (CHâCOF) due to fluorineâs electron-withdrawing effects:
Property | This compound | Acetyl Fluoride |
---|---|---|
Hydrolysis Rate | Faster (inductive effect) | Slower |
Thermal Stability | Lower (CâF bond lability) | Higher |
NMR Chemical Shift |
Wissenschaftliche Forschungsanwendungen
Key Applications
-
Organic Synthesis
- Fluoroacetyl fluoride is utilized in the synthesis of various fluorinated organic compounds. The introduction of fluorine can alter the reactivity of molecules, leading to new synthetic pathways.
- Example: It is used as a precursor for the synthesis of fluoroacetate derivatives, which are important in medicinal chemistry.
-
Pharmaceuticals
- Approximately 25% of small-molecule drugs contain fluorine atoms, enhancing their bioactivity and stability. This compound plays a role in synthesizing these fluorinated drugs.
- Case Study: Research indicates that fluorination can improve the efficacy of antitumor agents by modifying their pharmacokinetic profiles .
-
Materials Science
- The compound is used in developing advanced materials such as fluoropolymers, which exhibit exceptional chemical resistance and thermal stability.
- Applications: Fluoropolymers are widely used in coatings, electrical insulation, and automotive parts due to their durability and performance under extreme conditions .
Data Tables
Application Area | Specific Use | Benefits |
---|---|---|
Organic Synthesis | Synthesis of fluoroacetate derivatives | Enhanced reactivity and selectivity |
Pharmaceuticals | Development of new drugs | Increased bioactivity and metabolic stability |
Materials Science | Production of fluoropolymers | Superior chemical resistance and durability |
Case Studies
- Enzymatic Synthesis : Recent studies have focused on the enzymatic synthesis of fluorinated compounds, including fluoroacetyl derivatives. This method offers a greener alternative to traditional synthetic routes by utilizing enzymes to introduce fluorine selectively .
- Fluorinated Agrochemicals : this compound has been investigated for its role in developing more effective agrochemicals. The incorporation of fluorine into herbicides has been shown to enhance their potency and selectivity against target species while reducing environmental impact .
Wirkmechanismus
The mechanism of action of fluoroacetyl fluoride involves its ability to act as an acylating agent. It can react with nucleophiles, such as amino groups in proteins or enzymes, leading to the formation of stable acylated products . This property is particularly useful in biochemical studies where this compound is used to modify specific amino acid residues in enzymes, thereby altering their activity and providing insights into their catalytic mechanisms .
Vergleich Mit Àhnlichen Verbindungen
Key Properties:
- Boiling Point : 54°C
- Molecular Weight : 96.04 g/mol
- Reactivity : Hydrolyzes in water to form fluoroacetic acid (CHâFCOOH), a highly toxic metabolite .
- Applications : Primarily used as an acylating agent in organic synthesis, particularly for introducing fluorinated groups into pharmaceuticals and agrochemicals .
Physical Properties
The boiling points and molecular weights of fluoroacetyl fluoride and related acyl fluorides are compared below:
Key Observations :
- Fluorineâs electronegativity reduces boiling points compared to chlorine analogs (e.g., this compound vs. chloroacetyl fluoride) due to weaker intermolecular forces .
- Trithis compound, with three fluorine atoms, has a significantly lower boiling point (-28°C) owing to reduced molecular polarity and volatility .
Chemical Reactivity and Hydrolysis
Acyl fluorides are highly reactive, but their hydrolysis products determine their toxicity and environmental impact:
Key Observations :
- This compoundâs extreme toxicity arises from fluoroacetic acid, a potent inhibitor of the citric acid cycle .
- Chloroacetyl fluoride is less toxic because chloroacetic acid is metabolized more efficiently .
- Trifluoroacetic acid, while less acutely toxic, is environmentally persistent due to resistance to degradation .
Biologische AktivitÀt
Fluoroacetyl fluoride (FAF) is a compound of significant interest due to its biological activity and potential applications in various fields, including biochemistry and toxicology. This article provides a comprehensive overview of the biological activity of FAF, supported by case studies, research findings, and data tables.
Chemical Structure and Properties
This compound is an organofluorine compound characterized by the presence of both an acetyl group and a fluoride atom. Its chemical structure can be represented as:
This compound exhibits unique properties due to the electronegativity of fluorine, which influences its reactivity and interactions with biological molecules.
The biological activity of this compound primarily stems from its metabolic conversion to fluoroacetate, a potent inhibitor of key metabolic pathways. Once inside the organism, FAF is converted to fluoroacetyl-CoA, which subsequently participates in the tricarboxylic acid (TCA) cycle:
- Conversion to Fluoroacetate : FAF is hydrolyzed to yield fluoroacetate.
- Formation of Fluoroacetyl-CoA : Fluoroacetate is activated to fluoroacetyl-CoA through the action of CoA ligases.
- Inhibition of Metabolic Pathways : Fluoroacetyl-CoA competes with acetyl-CoA in various enzymatic reactions, particularly affecting the aconitase enzyme in the TCA cycle, leading to metabolic disruption.
Toxicity and Biological Impact
Fluoroacetate, derived from FAF, exhibits high toxicity comparable to some potent anticancer drugs. Its mechanism involves:
- Inhibition of Aconitase : The conversion of fluoroacetyl-CoA to fluorocitrate inhibits aconitase, blocking the TCA cycle and leading to energy depletion in cells.
- Calcium Homeostasis Disruption : The accumulation of citrate due to TCA cycle inhibition reduces ionized serum calcium levels, contributing to toxicity.
Table 1: Comparison of Biological Effects
Compound | Mechanism of Action | Toxicity Level |
---|---|---|
Fluoroacetate | Inhibits aconitase; disrupts TCA cycle | High |
Fluoroacetyl-CoA | Competes with acetyl-CoA; affects energy metabolism | Moderate to High |
Case Studies and Research Findings
- Enzymatic Studies : Research on fluoroacetyl-CoA-specific thioesterases like FlK from Streptomyces cattleya shows that these enzymes can discriminate between fluoroacetyl-CoA and acetyl-CoA with a significant kinetic advantage for the former. This specificity is crucial for understanding how organisms metabolize fluorinated compounds while avoiding self-poisoning .
- Microbial Resistance Mechanisms : Studies have identified bacterial mechanisms that allow for the detoxification of fluoroacetate through dehalogenation processes. These mechanisms involve specific enzymes that preferentially cleave C-F bonds, suggesting evolutionary adaptations to environmental organofluorine compounds .
- Toxicological Assessments : Longitudinal studies have demonstrated that exposure to fluorinated compounds can lead to neurological impairments and other health issues in sensitive populations. For instance, certain studies have linked high fluoride exposure with cognitive deficits in children .
Q & A
Q. How can Fluoroacetyl fluoride be synthesized in a laboratory setting, and what factors influence yield optimization?
Basic Research Question
this compound can be synthesized via two primary routes:
- Route 1 : Reaction of phosphorus oxychloride (POClâ) with fluoroacetamide, followed by hydrolysis.
- Route 2 : Direct reaction of POClâ with sodium or barium fluoroacetate salts.
Key considerations include:
- Purity of starting materials : Impurities in fluoroacetamide or salts reduce yields.
- Moisture control : Hydrolysis of intermediates must be minimized to avoid side reactions.
- Temperature : Exothermic reactions require careful temperature regulation (30â40°C optimal).
Yields exceeding 70% are achievable with anhydrous conditions and stoichiometric excess of POClâ .
Q. What analytical methods are recommended for detecting and quantifying this compound in experimental samples?
Basic Research Question
- Gas Chromatography-Mass Spectrometry (GC-MS) : Effective for volatile derivatives; use trifluoroacetic anhydride for derivatization to enhance detection limits (1â10 ppb) .
- Ion Chromatography (IC) : Suitable for aqueous samples; fluoride-specific electrodes can quantify hydrolyzed fluoride ions, though cross-validation with NMR is advised to confirm specificity .
- 19F NMR Spectroscopy : Provides structural confirmation and quantification in non-aqueous matrices (detection limit: ~0.1 mM) .
Critical Step : Sample preparation must exclude moisture to prevent hydrolysis to fluoroacetic acid .
Q. How do researchers reconcile discrepancies in reported acute toxicity data for this compound across studies?
Advanced Research Question
Discrepancies arise from:
- Exposure routes : Inhalation LCâ â values (e.g., 100 mg/mÂł in guinea pigs ) differ from oral or dermal toxicity due to absorption variability.
- Species-specific metabolism : Rodents exhibit higher fluoroacetate-CoA synthase activity, accelerating conversion to toxic fluorocitrate .
- Experimental design : Variability in exposure duration (e.g., 10-minute vs. 4-hour studies) impacts dose-response curves.
Resolution Strategy : Standardize protocols using OECD Test Guidelines 403 (inhalation) and 402 (dermal), with interspecies metabolic profiling .
Q. What experimental strategies are used to study the inhibition of the citric acid cycle by this compound derivatives?
Advanced Research Question
- In vitro assays : Isolated mitochondria are treated with fluoroacetyl-CoA to monitor fluorocitrate accumulation via LC-MS/MS. Activity of aconitase (target enzyme) is measured spectrophotometrically at 240 nm .
- Isotopic tracing : 13C-labeled this compound tracks metabolic flux disruption in cell cultures. Coupled with RNA-seq, this identifies compensatory pathways (e.g., glutaminolysis) .
- Computational modeling : Molecular docking simulations predict binding affinities of fluorocitrate to aconitase, validated by mutagenesis studies (e.g., Ser-567 in porcine aconitase) .
Q. What safety protocols are essential when handling this compound in laboratory settings?
Basic Research Question
- PPE : Acid-resistant gloves (e.g., neoprene), full-face respirators with fluoride-rated cartridges, and chemical aprons.
- Ventilation : Use fume hoods with â„100 fpm airflow; install HEPA filters to capture airborne particulates.
- Emergency response : Neutralize spills with calcium carbonate slurry; administer intravenous calcium gluconate for exposure treatment .
Documentation : Maintain SDS sheets referencing RTECS AP3150000 for toxicity profiles .
Q. How can isotopic labeling techniques elucidate the metabolic pathways of this compound in biological systems?
Advanced Research Question
- 18F radiolabeling : Synthesize 18F-fluoroacetyl fluoride via nucleophilic substitution (K18F + chloroacetyl fluoride). PET imaging tracks real-time distribution in murine models .
- 13C/2H labeling : Use 13C-CHâF-COF to trace incorporation into fluorocitrate via GC-IRMS. Kinetic isotope effects (KIEs) reveal rate-limiting steps in mitochondrial uptake .
Data Interpretation : Combine with metabolomics (e.g., HRMAS-NMR) to map metabolic perturbations .
Q. What are the key challenges in maintaining this compound stability during storage, and how are they mitigated?
Basic Research Question
- Hydrolysis sensitivity : Store under inert gas (Ar/Nâ) in sealed ampoules with molecular sieves (3Ă ) to scavenge moisture .
- Temperature : Long-term stability requires storage at â20°C; room temperature degradation occurs within 48 hours.
- Material compatibility : Use PTFE or glass containers; avoid metals (e.g., aluminum) due to fluoride corrosion .
Tables
Property | Value | Reference |
---|---|---|
Molecular Weight | 80.04 g/mol | |
LCâ â (Inhalation, Guinea Pig) | 100 mg/mÂł (10-minute exposure) | |
Optimal Synthesis Yield | 70â75% (Route 2) | |
Detection Limit (19F NMR) | 0.1 mM |
Eigenschaften
IUPAC Name |
2-fluoroacetyl fluoride | |
---|---|---|
Source | PubChem | |
URL | https://pubchem.ncbi.nlm.nih.gov | |
Description | Data deposited in or computed by PubChem | |
InChI |
InChI=1S/C2H2F2O/c3-1-2(4)5/h1H2 | |
Source | PubChem | |
URL | https://pubchem.ncbi.nlm.nih.gov | |
Description | Data deposited in or computed by PubChem | |
InChI Key |
LPGXUEOAYSYXNJ-UHFFFAOYSA-N | |
Source | PubChem | |
URL | https://pubchem.ncbi.nlm.nih.gov | |
Description | Data deposited in or computed by PubChem | |
Canonical SMILES |
C(C(=O)F)F | |
Source | PubChem | |
URL | https://pubchem.ncbi.nlm.nih.gov | |
Description | Data deposited in or computed by PubChem | |
Molecular Formula |
C2H2F2O | |
Source | PubChem | |
URL | https://pubchem.ncbi.nlm.nih.gov | |
Description | Data deposited in or computed by PubChem | |
DSSTOX Substance ID |
DTXSID70164784 | |
Record name | Acetyl fluoride, fluoro- | |
Source | EPA DSSTox | |
URL | https://comptox.epa.gov/dashboard/DTXSID70164784 | |
Description | DSSTox provides a high quality public chemistry resource for supporting improved predictive toxicology. | |
Molecular Weight |
80.03 g/mol | |
Source | PubChem | |
URL | https://pubchem.ncbi.nlm.nih.gov | |
Description | Data deposited in or computed by PubChem | |
CAS No. |
1514-42-7 | |
Record name | Acetyl fluoride, fluoro- | |
Source | CAS Common Chemistry | |
URL | https://commonchemistry.cas.org/detail?cas_rn=1514-42-7 | |
Description | CAS Common Chemistry is an open community resource for accessing chemical information. Nearly 500,000 chemical substances from CAS REGISTRY cover areas of community interest, including common and frequently regulated chemicals, and those relevant to high school and undergraduate chemistry classes. This chemical information, curated by our expert scientists, is provided in alignment with our mission as a division of the American Chemical Society. | |
Explanation | The data from CAS Common Chemistry is provided under a CC-BY-NC 4.0 license, unless otherwise stated. | |
Record name | Acetyl fluoride, fluoro- | |
Source | ChemIDplus | |
URL | https://pubchem.ncbi.nlm.nih.gov/substance/?source=chemidplus&sourceid=0001514427 | |
Description | ChemIDplus is a free, web search system that provides access to the structure and nomenclature authority files used for the identification of chemical substances cited in National Library of Medicine (NLM) databases, including the TOXNET system. | |
Record name | Acetyl fluoride, fluoro- | |
Source | EPA DSSTox | |
URL | https://comptox.epa.gov/dashboard/DTXSID70164784 | |
Description | DSSTox provides a high quality public chemistry resource for supporting improved predictive toxicology. | |
Retrosynthesis Analysis
AI-Powered Synthesis Planning: Our tool employs the Template_relevance Pistachio, Template_relevance Bkms_metabolic, Template_relevance Pistachio_ringbreaker, Template_relevance Reaxys, Template_relevance Reaxys_biocatalysis model, leveraging a vast database of chemical reactions to predict feasible synthetic routes.
One-Step Synthesis Focus: Specifically designed for one-step synthesis, it provides concise and direct routes for your target compounds, streamlining the synthesis process.
Accurate Predictions: Utilizing the extensive PISTACHIO, BKMS_METABOLIC, PISTACHIO_RINGBREAKER, REAXYS, REAXYS_BIOCATALYSIS database, our tool offers high-accuracy predictions, reflecting the latest in chemical research and data.
Strategy Settings
Precursor scoring | Relevance Heuristic |
---|---|
Min. plausibility | 0.01 |
Model | Template_relevance |
Template Set | Pistachio/Bkms_metabolic/Pistachio_ringbreaker/Reaxys/Reaxys_biocatalysis |
Top-N result to add to graph | 6 |
Feasible Synthetic Routes
Haftungsausschluss und Informationen zu In-Vitro-Forschungsprodukten
Bitte beachten Sie, dass alle Artikel und Produktinformationen, die auf BenchChem prĂ€sentiert werden, ausschlieĂlich zu Informationszwecken bestimmt sind. Die auf BenchChem zum Kauf angebotenen Produkte sind speziell fĂŒr In-vitro-Studien konzipiert, die auĂerhalb lebender Organismen durchgefĂŒhrt werden. In-vitro-Studien, abgeleitet von dem lateinischen Begriff "in Glas", beinhalten Experimente, die in kontrollierten Laborumgebungen unter Verwendung von Zellen oder Geweben durchgefĂŒhrt werden. Es ist wichtig zu beachten, dass diese Produkte nicht als Arzneimittel oder Medikamente eingestuft sind und keine Zulassung der FDA fĂŒr die Vorbeugung, Behandlung oder Heilung von medizinischen ZustĂ€nden, Beschwerden oder Krankheiten erhalten haben. Wir mĂŒssen betonen, dass jede Form der körperlichen EinfĂŒhrung dieser Produkte in Menschen oder Tiere gesetzlich strikt untersagt ist. Es ist unerlĂ€sslich, sich an diese Richtlinien zu halten, um die Einhaltung rechtlicher und ethischer Standards in Forschung und Experiment zu gewĂ€hrleisten.