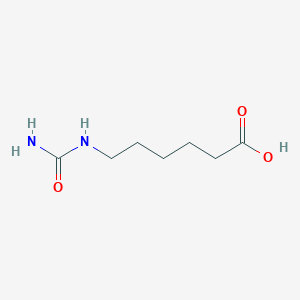
6-Ureidohexansäure
- Klicken Sie auf QUICK INQUIRY, um ein Angebot von unserem Expertenteam zu erhalten.
- Mit qualitativ hochwertigen Produkten zu einem WETTBEWERBSFÄHIGEN Preis können Sie sich mehr auf Ihre Forschung konzentrieren.
Übersicht
Beschreibung
6-Ureidohexanoic acid is a chemical compound with the molecular formula C7H14N2O3 and a molecular weight of 174.2 g/mol. It is a derivative of hexanoic acid, where the hexanoic acid chain is modified with a ureido group.
Wissenschaftliche Forschungsanwendungen
6-Ureidohexanoic acid has several applications in scientific research, including:
Chemistry: It is used as a building block for the synthesis of more complex molecules and as a reagent in various chemical reactions.
Biology: The compound is studied for its potential as an enzyme inhibitor, particularly for enzymes involved in metabolic pathways.
Medicine: Research has shown that 6-Ureidohexanoic acid can act as an inhibitor for tumor-relevant carbonic anhydrases IX and XII, making it a potential candidate for cancer therapy.
Industry: It is used in the synthesis of polymers and other materials with specific properties.
Wirkmechanismus
Target of Action
6-Ureidohexanoic acid is a potent and selective inhibitor for the tumor-relevant carbonic anhydrases IX and XII . Carbonic anhydrases are metalloenzymes that maintain pH homeostasis by catalyzing the reversible hydration of carbon dioxide to bicarbonate and a proton . They are involved in various physiological and pathological processes, including tumorigenesis .
Mode of Action
It is known to inhibit the activity of carbonic anhydrases ix and xii . These enzymes are overexpressed in many types of tumors and contribute to tumor growth and survival . By inhibiting these enzymes, 6-Ureidohexanoic acid may interfere with tumor growth and proliferation .
Biochemical Pathways
The biochemical pathways affected by 6-Ureidohexanoic acid are likely related to its inhibition of carbonic anhydrases IX and XII. These enzymes play a crucial role in maintaining pH homeostasis and are involved in various biochemical pathways . .
Result of Action
The molecular and cellular effects of 6-Ureidohexanoic acid’s action are likely related to its inhibition of carbonic anhydrases IX and XII. By inhibiting these enzymes, 6-Ureidohexanoic acid may interfere with tumor growth and proliferation . .
Vorbereitungsmethoden
Synthetic Routes and Reaction Conditions
6-Ureidohexanoic acid can be synthesized through the reaction of hexanoic acid with urea under specific conditions. The reaction typically involves heating hexanoic acid with urea in the presence of a catalyst to facilitate the formation of the ureido group. The reaction conditions, such as temperature and reaction time, are optimized to achieve high yields and purity of the product.
Industrial Production Methods
In industrial settings, the production of 6-Ureidohexanoic acid may involve large-scale synthesis using similar reaction conditions as in laboratory synthesis. The process is scaled up to accommodate higher volumes, and additional purification steps, such as recrystallization or chromatography, may be employed to ensure the desired purity and quality of the compound.
Analyse Chemischer Reaktionen
Types of Reactions
6-Ureidohexanoic acid undergoes various chemical reactions, including:
Oxidation: The compound can be oxidized to form corresponding oxo derivatives.
Reduction: Reduction reactions can convert the ureido group to an amine group.
Substitution: The ureido group can participate in nucleophilic substitution reactions, leading to the formation of various derivatives.
Common Reagents and Conditions
Oxidation: Common oxidizing agents such as potassium permanganate or chromium trioxide can be used under acidic or basic conditions.
Reduction: Reducing agents like lithium aluminum hydride or sodium borohydride are typically employed.
Substitution: Nucleophiles such as amines or alcohols can react with the ureido group under mild conditions.
Major Products Formed
Oxidation: Oxo derivatives of 6-Ureidohexanoic acid.
Reduction: Amino derivatives of 6-Ureidohexanoic acid.
Substitution: Various substituted ureidohexanoic acid derivatives.
Vergleich Mit ähnlichen Verbindungen
Similar Compounds
6-Aminohexanoic acid: A derivative of hexanoic acid with an amino group instead of a ureido group.
6-Dodecyloxy-6-oxohexylammonium 6-dodecyloxy-6-oxohexylcarbamate: A transdermal penetration enhancer derived from 6-aminohexanoic acid.
Uniqueness
6-Ureidohexanoic acid is unique due to its specific inhibition of carbonic anhydrases IX and XII, which are relevant in tumor growth and survival. This specificity makes it a valuable compound in cancer research and potential therapeutic applications.
Eigenschaften
IUPAC Name |
6-(carbamoylamino)hexanoic acid |
Source
|
---|---|---|
Source | PubChem | |
URL | https://pubchem.ncbi.nlm.nih.gov | |
Description | Data deposited in or computed by PubChem | |
InChI |
InChI=1S/C7H14N2O3/c8-7(12)9-5-3-1-2-4-6(10)11/h1-5H2,(H,10,11)(H3,8,9,12) |
Source
|
Source | PubChem | |
URL | https://pubchem.ncbi.nlm.nih.gov | |
Description | Data deposited in or computed by PubChem | |
InChI Key |
BQNWWHFSEXYHKU-UHFFFAOYSA-N |
Source
|
Source | PubChem | |
URL | https://pubchem.ncbi.nlm.nih.gov | |
Description | Data deposited in or computed by PubChem | |
Canonical SMILES |
C(CCC(=O)O)CCNC(=O)N |
Source
|
Source | PubChem | |
URL | https://pubchem.ncbi.nlm.nih.gov | |
Description | Data deposited in or computed by PubChem | |
Molecular Formula |
C7H14N2O3 |
Source
|
Source | PubChem | |
URL | https://pubchem.ncbi.nlm.nih.gov | |
Description | Data deposited in or computed by PubChem | |
DSSTOX Substance ID |
DTXSID10332723 |
Source
|
Record name | 6-ureidohexanoic acid | |
Source | EPA DSSTox | |
URL | https://comptox.epa.gov/dashboard/DTXSID10332723 | |
Description | DSSTox provides a high quality public chemistry resource for supporting improved predictive toxicology. | |
Molecular Weight |
174.20 g/mol |
Source
|
Source | PubChem | |
URL | https://pubchem.ncbi.nlm.nih.gov | |
Description | Data deposited in or computed by PubChem | |
CAS No. |
1468-42-4 |
Source
|
Record name | 6-ureidohexanoic acid | |
Source | EPA DSSTox | |
URL | https://comptox.epa.gov/dashboard/DTXSID10332723 | |
Description | DSSTox provides a high quality public chemistry resource for supporting improved predictive toxicology. | |
Haftungsausschluss und Informationen zu In-Vitro-Forschungsprodukten
Bitte beachten Sie, dass alle Artikel und Produktinformationen, die auf BenchChem präsentiert werden, ausschließlich zu Informationszwecken bestimmt sind. Die auf BenchChem zum Kauf angebotenen Produkte sind speziell für In-vitro-Studien konzipiert, die außerhalb lebender Organismen durchgeführt werden. In-vitro-Studien, abgeleitet von dem lateinischen Begriff "in Glas", beinhalten Experimente, die in kontrollierten Laborumgebungen unter Verwendung von Zellen oder Geweben durchgeführt werden. Es ist wichtig zu beachten, dass diese Produkte nicht als Arzneimittel oder Medikamente eingestuft sind und keine Zulassung der FDA für die Vorbeugung, Behandlung oder Heilung von medizinischen Zuständen, Beschwerden oder Krankheiten erhalten haben. Wir müssen betonen, dass jede Form der körperlichen Einführung dieser Produkte in Menschen oder Tiere gesetzlich strikt untersagt ist. Es ist unerlässlich, sich an diese Richtlinien zu halten, um die Einhaltung rechtlicher und ethischer Standards in Forschung und Experiment zu gewährleisten.