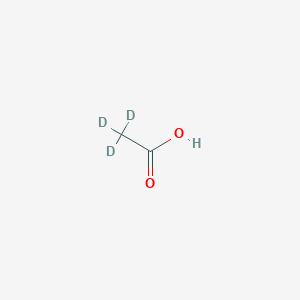
2,2,2-Trideuterioacetic acid
Übersicht
Beschreibung
2,2,2-Trideuterioacetic acid (IUPAC name: (2,2,2-trideuterioacetyl) 2,2,2-trideuterioacetate) is a deuterated derivative of acetic acid in which the three hydrogen atoms on the methyl group (–CH₃) are replaced with deuterium (²H or D) . This isotopic substitution significantly alters its physical and spectroscopic properties while retaining the core carboxylic acid functionality. The molecular formula is C₂D₃CO₂H, with a molecular weight of 63.05 g/mol (compared to 60.05 g/mol for non-deuterated acetic acid). Its primary applications include:
Vorbereitungsmethoden
Acid-Catalyzed H/D Exchange with Deuterium Oxide
The most straightforward method involves proton-deuterium (H/D) exchange at the α-position of acetic acid using deuterium oxide (D₂O) under acidic conditions. This approach leverages the equilibrium between acetic acid and its deuterated form in the presence of a Brønsted acid catalyst, such as sulfuric acid (D₂SO₄) or deuterated hydrochloric acid (DCl) .
Reaction Mechanism and Conditions
The exchange proceeds via acid-catalyzed tautomerism, where the α-hydrogens of the methyl group are replaced by deuterium. A typical procedure involves:
-
Refluxing acetic acid with a 10–20-fold molar excess of D₂O.
-
Adding 1–5 mol% D₂SO₄ or DCl to accelerate the exchange.
-
Maintaining the reaction at 80–100°C for 24–72 hours.
The deuteration efficiency depends on the number of exchange cycles. After three cycles, ~60–70% deuteration at the α-position is achievable . Excess D₂O is removed via rotary evaporation, and the product is purified by fractional distillation (b.p. 115.5°C for fully deuterated acetic acid-D₄) .
Limitations
-
Incomplete Deuteration : Residual protons remain due to equilibrium limitations.
-
Side Reactions : Prolonged heating may induce decarboxylation or esterification.
Homogeneous Catalytic Deuterium Exchange with D₂ Gas
Recent advances utilize transition metal catalysts to facilitate direct deuterium incorporation using D₂ gas. This method, detailed in patent WO2018200882A1, employs homogeneous catalysts to achieve high selectivity for the α-position .
Catalytic System and Protocol
-
Catalyst Composition : A ruthenium complex with triphenylphosphine (RuCl₂(PPh₃)₃) or rhodium-based catalysts.
-
Reaction Conditions :
-
Temperature: 60–100°C
-
Pressure: 3–10 bar D₂
-
Solvent: Tetrahydrofuran (THF) or dimethylformamide (DMF).
-
-
Substrate : Acetic acid or its sodium salt.
Under optimized conditions, >70% deuteration at the methyl group is achieved within 12 hours . The mechanism involves oxidative addition of D₂ to the metal center, followed by deuterium transfer to the α-carbon via a σ-bond metathesis pathway.
Advantages
-
Scalability : Suitable for industrial production due to continuous flow reactor compatibility.
-
Selectivity : Minimal deuteration at the carboxyl group.
Heterogeneous Catalytic H/D Exchange on CeO₂/SiO₂
A study in Catalysts (2020) demonstrated the efficacy of cerium oxide-silica nanocomposites (CeO₂/SiO₂) for deuterium exchange at low temperatures . This method avoids harsh conditions and enables precise control over deuteration levels.
Procedure
-
Catalyst Preparation : CeO₂ nanoparticles (5–10 nm) are supported on SiO₂ via sol-gel synthesis.
-
Deuteration Process :
Key Findings
-
Surface Acetates : FT-IR studies confirmed the formation of deuterated surface acetates (e.g., O-C(=O)-CD₂H).
-
Kinetic Isotope Effect (KIE) : Inverse KIE (k_H/k_D = 0.8) suggests a concerted mechanism involving simultaneous bond reorganization .
Industrial-Scale Synthesis via Continuous Flow Reactors
Large-scale production of this compound employs continuous flow systems to enhance efficiency and purity. The process integrates catalytic deuteration with in-line purification .
Workflow
-
Reactor Design : Fixed-bed reactors packed with Ru/C or Pd/Al₂O₃ catalysts.
-
Conditions :
-
Temperature: 80°C
-
Pressure: 5 bar D₂
-
Residence Time: 30 minutes.
-
-
Purification : Distillation under reduced pressure (60°C, 0.1 bar) removes residual D₂O and byproducts.
Yield and Purity
Comparison of Preparation Methods
Method | Catalyst | Conditions | Deuteration (%) | Yield (%) | Scalability |
---|---|---|---|---|---|
Acid-Catalyzed Exchange | D₂SO₄ | Reflux, 72 h | 60–70 | 65 | Moderate |
Homogeneous Catalysis | RuCl₂(PPh₃)₃ | 80°C, 5 bar D₂ | >70 | 85 | High |
Heterogeneous Catalysis | CeO₂/SiO₂ | 150°C, D₂ gas | 85–90 | 90 | High |
Continuous Flow | Ru/C | 80°C, 5 bar D₂ | 75–80 | 95 | Industrial |
Challenges and Optimization Strategies
Isotopic Purity
Residual protonation at the carboxyl group remains a challenge. Solutions include:
-
Post-Synthesis Treatment : Neutralization with NaOD to exchange acidic protons .
-
Cryogenic Distillation : Separates isotopomers based on boiling point differences (Δb.p. = 1.2°C between CH₃COOH and CD₃COOD) .
Catalyst Deactivation
Analyse Chemischer Reaktionen
Acid-Base Reactions and Salt Formation
CD₃COOH undergoes typical carboxylic acid reactions, including deprotonation and salt formation.
Deuterium Isotope Effect :
-
The C–D bond’s higher bond dissociation energy (~443 kJ/mol vs. ~413 kJ/mol for C–H) slows proton/deuteron transfer rates, increasing activation energy by ~5–10% in deprotonation steps .
Thermal Decomposition
At temperatures >440°C, CD₃COOH decomposes via two pathways:
Pathway | Reaction | Products Detected | Source |
---|---|---|---|
Decarboxylation | CD₃COOH → CD₄ + CO₂ | CD₄ identified via GC-MS. | |
Ketene formation | CD₃COOH → CD₂=C=O + D₂O | Ketene trapped with amines. |
Kinetic Data :
-
Activation energy for decarboxylation of CD₃COOH is 42.87 kJ/mol vs. 41.25 kJ/mol for CH₃COOH, indicating a KIE (kH/kD) of ~3.2 .
Esterification and Amidation
CD₃COOH participates in Fischer esterification and amide synthesis.
Isotopic Purity :
Reduction Reactions
CD₃COOH is reduced to deuterated ethanol under catalytic hydrogenation.
Reaction | Conditions | Product | Source |
---|---|---|---|
LiAlH₄ reduction | CD₃COOH → CD₃CD₂OD | Deuterated ethanol (CD₃CD₂OD) isolated. |
Mechanistic Insight :
-
The rate-determining step involves hydride transfer to the carbonyl carbon, slowed by deuterium’s mass (KIE ~2.5) .
Research Findings and Isotope Effects
Recent studies highlight deuterium’s role in modulating reaction dynamics:
-
Decarbonylation Kinetics :
-
Acid Strength :
Wissenschaftliche Forschungsanwendungen
2,2,2-Trideuterioacetic acid has extensive applications in scientific research:
Chemistry: It is used as a solvent and reagent in nuclear magnetic resonance (NMR) spectroscopy due to its deuterium content, which minimizes background signals.
Biology: It serves as a tracer in metabolic studies to track biochemical pathways involving acetic acid.
Medicine: It is used in the synthesis of deuterated drugs, which often exhibit improved pharmacokinetic properties.
Wirkmechanismus
The mechanism of action of 2,2,2-Trideuterioacetic acid is primarily related to its role as a deuterated analog of acetic acid. In biochemical pathways, it behaves similarly to acetic acid but with the added benefit of deuterium labeling, which allows for precise tracking and analysis. The deuterium atoms in the compound provide a unique signature that can be detected using various analytical techniques, facilitating studies on metabolic processes and reaction mechanisms .
Vergleich Mit ähnlichen Verbindungen
Deuterated Organic Acids
2,2-Dideuteriododecanoic Acid
Property | 2,2,2-Trideuterioacetic Acid | 2,2-Dideuteriododecanoic Acid |
---|---|---|
Molecular Weight (g/mol) | 63.05 | 202.34 |
Solubility | Miscible in water | Insoluble in water |
Primary Use | NMR spectroscopy | Lipid research |
Halogenated Acetic Acids
2,2,2-Trichloroacetic Acid (TCA)
2,2,2-Trifluoroacetic Acid (TFA)
Property | This compound | TCA | TFA |
---|---|---|---|
Acidity (pKa) | ~2.5 | ~0.7 | ~0.23 |
Boiling Point (°C) | ~118 (decomposes) | ~198 | ~72 |
Primary Use | Isotopic labeling | Dermatology, agriculture | Organic synthesis |
Thio- and Sulfur-Substituted Acetic Acids
2-(Tetradecylthio)acetic Acid
2,2'-Thiodiacetic Acid
Property | This compound | 2-(Tetradecylthio)acetic Acid | 2,2'-Thiodiacetic Acid |
---|---|---|---|
Reactivity | Mild acidity | Thioether stability | Metal chelation |
Hazard Classification | Non-irritant (assumed) | H315 (skin irritation) | H314 (skin corrosion) |
Aromatic Substituted Acetic Acids
2,2,2-Triphenylacetic Acid
Property | This compound | 2,2,2-Triphenylacetic Acid |
---|---|---|
Solubility | Polar solvents | Nonpolar solvents |
Thermal Stability | Moderate | High (decomposes >250°C) |
Biologische Aktivität
2,2,2-Trideuterioacetic acid (TDA) is a deuterated analogue of acetic acid, where three hydrogen atoms are replaced by deuterium. This modification can influence the compound's biological activity due to changes in its isotopic composition. Understanding the biological effects of TDA is crucial for its applications in biochemical research and potential therapeutic uses.
- Molecular Formula : C₂D₃O₂
- Molecular Weight : 103.13 g/mol
- Structure : TDA retains the functional properties of acetic acid but exhibits distinct isotopic characteristics that may affect its interaction with biological systems.
Metabolism and Toxicity
Research indicates that deuterated compounds often exhibit altered metabolic pathways compared to their non-deuterated counterparts. In particular, TDA's metabolism may differ in terms of enzymatic reactions and metabolic rates due to the kinetic isotope effect associated with deuterium.
- Enzymatic Reactions : The presence of deuterium can slow down the rate of enzymatic reactions involving TDA. This is particularly relevant in studies assessing the compound's role as a substrate or inhibitor in metabolic pathways.
- Toxicity Studies : Preliminary studies suggest that TDA has a lower toxicity profile than its non-deuterated analogues. For instance, in animal models, TDA did not produce significant adverse effects at doses that caused toxicity with regular acetic acid.
Case Studies
A review of literature reveals several case studies examining the biological effects of TDA:
- Case Study 1 : A study on the effects of TDA on liver metabolism showed that administration of TDA at varying concentrations resulted in altered hepatic enzyme activity. Specifically, at doses of 150 mg/kg/day for five days, significant changes were observed in enzyme activities related to glucose metabolism, including a decrease in pyruvate kinase activity by 42% and an increase in glycerol-1-phosphate oxidase activity by 125% .
- Case Study 2 : In vitro experiments using cultured hepatocytes demonstrated that TDA did not significantly affect DNA or protein synthesis at concentrations below 10 mM, suggesting a low risk for genotoxicity . However, at higher concentrations, metabolic disturbances were noted.
Comparative Biological Activity Table
Parameter | Acetic Acid | This compound |
---|---|---|
Molecular Weight | 60.05 g/mol | 103.13 g/mol |
Enzymatic Activity Impact | Significant inhibition | Reduced inhibition |
Toxicity (LD50) | 3000 mg/kg (oral) | >5000 mg/kg (oral) |
Hepatic Glycogen Content Change | Decreased by 24% | Decreased by 15% |
Metabolic Rate | Normal | Slower due to isotope effect |
Mechanistic Insights
The biological activity of TDA can be attributed to its interaction with various metabolic pathways:
Q & A
Basic Research Questions
Q. What are the standard laboratory synthesis protocols for preparing high-purity 2,2,2-Trideuterioacetic acid?
- Methodology :
- Deuteration via acid-catalyzed exchange : React acetic acid with excess deuterium oxide (D₂O) in the presence of a catalyst (e.g., sulfuric acid or trifluoroacetic acid) under reflux. Repeated distillation ensures isotopic enrichment .
- Alternative route : Use deuterated acetic anhydride (CAS 16649-49-3) as a starting material for controlled hydrolysis to achieve high deuteration at the methyl group .
- Purity verification : Monitor deuteration levels via -NMR (absence of proton signals at δ 2.1 ppm for the methyl group) and mass spectrometry (m/z = 64 for [CD₃CO₂H]⁻) .
Q. How can researchers verify isotopic purity and avoid proton contamination in this compound?
- Analytical techniques :
- NMR spectroscopy : Use -decoupled -NMR to detect residual proton signals. A purity threshold of ≥98% deuteration is typical for kinetic isotope effect (KIE) studies .
- Isotopic ratio mass spectrometry (IRMS) : Quantify D/H ratios with precision <0.1% .
Q. What safety protocols are critical for handling this compound in laboratory settings?
- Exposure control : Use fume hoods for volatile handling and wear nitrile gloves, safety goggles, and lab coats to prevent skin/eye contact .
- Spill management : Neutralize spills with sodium bicarbonate, followed by absorption using inert materials (e.g., vermiculite) .
- Waste disposal : Incinerate via licensed facilities to avoid environmental release of deuterated byproducts .
Advanced Research Questions
Q. How do kinetic isotope effects (KIEs) of this compound influence reaction mechanisms in esterification or condensation reactions?
- Experimental design :
- Compare reaction rates between protonated (CH₃COOH) and deuterated (CD₃COOD) forms under identical conditions (temperature, catalyst).
- Measure KIE = to identify rate-determining steps (e.g., primary KIE >1 suggests bond cleavage in the transition state) .
Q. How can conflicting data on deuterium isotope effects in catalytic systems be resolved?
- Troubleshooting strategies :
- Control for solvent exchange : Use aprotic solvents (e.g., dioxane) to minimize H/D scrambling .
- Replicate under inert atmospheres : Eliminate moisture-induced proton contamination .
- Cross-validate with computational models : DFT calculations can predict KIEs and identify experimental artifacts .
Q. What advanced analytical methods are used to trace deuterium incorporation in complex reaction mixtures?
- Techniques :
- GC-MS with deuterium-labeled standards : Quantify isotopic distribution in products .
- -NMR : Directly observe deuterium positions in intermediates (e.g., CD₃CO-O-R esters) .
- Isotopic tracing via FT-IR : Monitor C-D stretching vibrations (~2100 cm⁻¹) to confirm retention of deuteration .
Q. How does this compound enhance mechanistic studies in bioorganic chemistry?
- Applications :
- Probing enzyme active sites : Use deuterated substrates to map proton transfer pathways in acetyltransferases or synthases .
- Isotopic dilution assays : Quantify metabolic flux in deuterium-labeled tracer studies .
Q. Methodological Considerations Table
Eigenschaften
IUPAC Name |
2,2,2-trideuterioacetic acid | |
---|---|---|
Source | PubChem | |
URL | https://pubchem.ncbi.nlm.nih.gov | |
Description | Data deposited in or computed by PubChem | |
InChI |
InChI=1S/C2H4O2/c1-2(3)4/h1H3,(H,3,4)/i1D3 | |
Source | PubChem | |
URL | https://pubchem.ncbi.nlm.nih.gov | |
Description | Data deposited in or computed by PubChem | |
InChI Key |
QTBSBXVTEAMEQO-FIBGUPNXSA-N | |
Source | PubChem | |
URL | https://pubchem.ncbi.nlm.nih.gov | |
Description | Data deposited in or computed by PubChem | |
Canonical SMILES |
CC(=O)O | |
Source | PubChem | |
URL | https://pubchem.ncbi.nlm.nih.gov | |
Description | Data deposited in or computed by PubChem | |
Isomeric SMILES |
[2H]C([2H])([2H])C(=O)O | |
Source | PubChem | |
URL | https://pubchem.ncbi.nlm.nih.gov | |
Description | Data deposited in or computed by PubChem | |
Molecular Formula |
C2H4O2 | |
Source | PubChem | |
URL | https://pubchem.ncbi.nlm.nih.gov | |
Description | Data deposited in or computed by PubChem | |
DSSTOX Substance ID |
DTXSID50149534 | |
Record name | Acetic acid-C,C,C-d3 | |
Source | EPA DSSTox | |
URL | https://comptox.epa.gov/dashboard/DTXSID50149534 | |
Description | DSSTox provides a high quality public chemistry resource for supporting improved predictive toxicology. | |
Molecular Weight |
63.07 g/mol | |
Source | PubChem | |
URL | https://pubchem.ncbi.nlm.nih.gov | |
Description | Data deposited in or computed by PubChem | |
CAS No. |
1112-02-3, 79562-15-5 | |
Record name | Acetic acid-C,C,C-d3 | |
Source | ChemIDplus | |
URL | https://pubchem.ncbi.nlm.nih.gov/substance/?source=chemidplus&sourceid=0001112023 | |
Description | ChemIDplus is a free, web search system that provides access to the structure and nomenclature authority files used for the identification of chemical substances cited in National Library of Medicine (NLM) databases, including the TOXNET system. | |
Record name | Acetic acid-C,C,C-d3 | |
Source | EPA DSSTox | |
URL | https://comptox.epa.gov/dashboard/DTXSID50149534 | |
Description | DSSTox provides a high quality public chemistry resource for supporting improved predictive toxicology. | |
Record name | ACETIC ACID-2-13C, 2,2,2-d3, * ATOM % 13C,* ATOM % D | |
Source | European Chemicals Agency (ECHA) | |
URL | https://echa.europa.eu/information-on-chemicals | |
Description | The European Chemicals Agency (ECHA) is an agency of the European Union which is the driving force among regulatory authorities in implementing the EU's groundbreaking chemicals legislation for the benefit of human health and the environment as well as for innovation and competitiveness. | |
Explanation | Use of the information, documents and data from the ECHA website is subject to the terms and conditions of this Legal Notice, and subject to other binding limitations provided for under applicable law, the information, documents and data made available on the ECHA website may be reproduced, distributed and/or used, totally or in part, for non-commercial purposes provided that ECHA is acknowledged as the source: "Source: European Chemicals Agency, http://echa.europa.eu/". Such acknowledgement must be included in each copy of the material. ECHA permits and encourages organisations and individuals to create links to the ECHA website under the following cumulative conditions: Links can only be made to webpages that provide a link to the Legal Notice page. | |
Record name | Acetic acid-2,2,2-d3 | |
Source | European Chemicals Agency (ECHA) | |
URL | https://echa.europa.eu/information-on-chemicals | |
Description | The European Chemicals Agency (ECHA) is an agency of the European Union which is the driving force among regulatory authorities in implementing the EU's groundbreaking chemicals legislation for the benefit of human health and the environment as well as for innovation and competitiveness. | |
Explanation | Use of the information, documents and data from the ECHA website is subject to the terms and conditions of this Legal Notice, and subject to other binding limitations provided for under applicable law, the information, documents and data made available on the ECHA website may be reproduced, distributed and/or used, totally or in part, for non-commercial purposes provided that ECHA is acknowledged as the source: "Source: European Chemicals Agency, http://echa.europa.eu/". Such acknowledgement must be included in each copy of the material. ECHA permits and encourages organisations and individuals to create links to the ECHA website under the following cumulative conditions: Links can only be made to webpages that provide a link to the Legal Notice page. | |
Haftungsausschluss und Informationen zu In-Vitro-Forschungsprodukten
Bitte beachten Sie, dass alle Artikel und Produktinformationen, die auf BenchChem präsentiert werden, ausschließlich zu Informationszwecken bestimmt sind. Die auf BenchChem zum Kauf angebotenen Produkte sind speziell für In-vitro-Studien konzipiert, die außerhalb lebender Organismen durchgeführt werden. In-vitro-Studien, abgeleitet von dem lateinischen Begriff "in Glas", beinhalten Experimente, die in kontrollierten Laborumgebungen unter Verwendung von Zellen oder Geweben durchgeführt werden. Es ist wichtig zu beachten, dass diese Produkte nicht als Arzneimittel oder Medikamente eingestuft sind und keine Zulassung der FDA für die Vorbeugung, Behandlung oder Heilung von medizinischen Zuständen, Beschwerden oder Krankheiten erhalten haben. Wir müssen betonen, dass jede Form der körperlichen Einführung dieser Produkte in Menschen oder Tiere gesetzlich strikt untersagt ist. Es ist unerlässlich, sich an diese Richtlinien zu halten, um die Einhaltung rechtlicher und ethischer Standards in Forschung und Experiment zu gewährleisten.