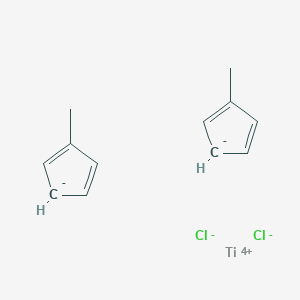
Dichlorobis(methyl-π-cyclopentadienyl)titan
Übersicht
Beschreibung
Bis(methylcyclopentadienyl)titanium dichloride is an organometallic compound that belongs to the class of metallocenes. It consists of a titanium atom bonded to two methyl-pi-cyclopentadienyl ligands and two chlorine atoms. This compound is of significant interest in the field of organometallic chemistry due to its unique structural and electronic properties, which make it a versatile catalyst and reagent in various chemical reactions.
Wissenschaftliche Forschungsanwendungen
Bis(methylcyclopentadienyl)titanium dichloride has a wide range of applications in scientific research:
Biology: The compound is studied for its potential biological activity and interactions with biomolecules.
Medicine: Research is ongoing to explore its potential use in medicinal chemistry, particularly in the development of new drugs and therapeutic agents.
Industry: It is used in the production of high-performance materials, such as advanced polymers and coatings.
Wirkmechanismus
Mode of Action
Dichlorobis(methyl-pi-cyclopentadienyl)titanium is known to react with bidentate Schiff bases in anhydrous tetrahydrofuran in the presence of triethylamine . This suggests that the compound can form complexes with other molecules, potentially altering their function or activity. The exact changes resulting from these interactions would depend on the specific targets involved.
Result of Action
Given its reactivity with bidentate Schiff bases , it’s plausible that the compound could influence the function of proteins or other biomolecules that contain similar functional groups.
Vorbereitungsmethoden
Synthetic Routes and Reaction Conditions
Bis(methylcyclopentadienyl)titanium dichloride can be synthesized through the reaction of titanium tetrachloride with methylcyclopentadienyl sodium in anhydrous tetrahydrofuran. The reaction typically proceeds as follows:
TiCl4+2NaC5H4CH3→(CH3C5H4)2TiCl2+2NaCl
The reaction mixture is stirred at room temperature for several hours, and the resulting product is purified by recrystallization from n-hexane .
Industrial Production Methods
Industrial production of dichlorobis(methyl-pi-cyclopentadienyl)titanium follows similar synthetic routes but on a larger scale. The reaction conditions are optimized to ensure high yield and purity of the product. The use of continuous flow reactors and automated systems helps in scaling up the production process while maintaining consistency and efficiency.
Analyse Chemischer Reaktionen
Types of Reactions
Bis(methylcyclopentadienyl)titanium dichloride undergoes various types of chemical reactions, including:
Oxidation: The compound can be oxidized to form titanium dioxide and other oxidation products.
Reduction: It can be reduced to lower oxidation states of titanium.
Substitution: The chlorine atoms can be substituted with other ligands, such as alkyl or aryl groups.
Common Reagents and Conditions
Oxidation: Common oxidizing agents include oxygen, hydrogen peroxide, and other peroxides.
Reduction: Reducing agents such as lithium aluminum hydride or sodium borohydride are used.
Substitution: Substitution reactions often involve the use of Grignard reagents or organolithium compounds.
Major Products Formed
Oxidation: Titanium dioxide and other titanium oxides.
Reduction: Lower oxidation state titanium complexes.
Substitution: Various substituted titanium complexes depending on the substituent used.
Vergleich Mit ähnlichen Verbindungen
Similar Compounds
Dichlorobis(cyclopentadienyl)titanium: Similar structure but without the methyl groups on the cyclopentadienyl ligands.
Dichlorobis(ethyl-pi-cyclopentadienyl)titanium: Similar structure with ethyl groups instead of methyl groups.
Dichlorobis(phenyl-pi-cyclopentadienyl)titanium: Similar structure with phenyl groups instead of methyl groups.
Uniqueness
Bis(methylcyclopentadienyl)titanium dichloride is unique due to the presence of methyl groups on the cyclopentadienyl ligands, which can influence its reactivity and selectivity in various chemical reactions. The methyl groups can provide steric hindrance and electronic effects that differentiate it from other similar compounds .
Biologische Aktivität
Bis(methylcyclopentadienyl)titanium dichloride (Cp2TiCl2) is a metallocene compound that has garnered attention in both organic synthesis and biological research due to its unique structural properties and potential therapeutic applications. This article explores its biological activity, focusing on its mechanisms of action, cytotoxicity, and implications for medicinal chemistry.
Chemical Structure and Properties
Bis(methylcyclopentadienyl)titanium dichloride consists of a titanium center coordinated by two methylcyclopentadienyl ligands and two chloride ions. Its molecular formula is C10H12Cl2Ti, and it exhibits a pseudo-tetrahedral geometry around the titanium atom. The compound's structure contributes to its reactivity and interaction with biological molecules.
The biological activity of bis(methylcyclopentadienyl)titanium dichloride can be attributed to several mechanisms:
- Reactivity with Biomolecules : The compound can form complexes with biomolecules such as proteins and nucleic acids, potentially leading to alterations in their structure and function.
- Catalytic Activity : As a catalyst, it facilitates various chemical reactions that may produce reactive intermediates capable of interacting with cellular components.
- Oxidative Stress Induction : Titanium complexes are known to generate reactive oxygen species (ROS), which can lead to oxidative stress in cells, contributing to cytotoxic effects.
Cytotoxicity Studies
Research has demonstrated the cytotoxic potential of bis(methylcyclopentadienyl)titanium dichloride against various cancer cell lines. A comparative analysis of cytotoxicity using the MTT assay revealed significant findings:
Compound | Cell Line | IC50 (μM) |
---|---|---|
Cp2TiCl2 | HT-29 (colon cancer) | 413 |
Titanocene Y | HT-29 | 2.1 |
Titanocene C | HT-29 | 8.9 |
These results indicate that while bis(methylcyclopentadienyl)titanium dichloride exhibits cytotoxicity, other titanocene derivatives show enhanced activity against cancer cells, suggesting that structural modifications can improve therapeutic efficacy .
Case Studies
- Antineoplastic Properties : A study highlighted the antineoplastic properties of titanocene compounds, including bis(methylcyclopentadienyl)titanium dichloride, which showed effectiveness against cancer cell lines resistant to traditional chemotherapy agents . The study emphasized the importance of understanding the structure-activity relationship in developing more potent anticancer drugs.
- Functionalization for Enhanced Activity : Research on amide-functionalized titanocenes demonstrated that modifying the ligand environment around titanium could significantly enhance cytotoxicity. For instance, titanocenes with specific substituents exhibited lower IC50 values compared to bis(methylcyclopentadienyl)titanium dichloride, indicating that ligand modification plays a crucial role in biological activity .
- Mechanistic Insights : Investigations into the mechanism of action revealed that bis(methylcyclopentadienyl)titanium dichloride induces apoptosis in cancer cells through ROS generation and subsequent activation of apoptotic pathways . This highlights the potential for using this compound as a lead structure in drug development aimed at cancer therapy.
Eigenschaften
IUPAC Name |
1-methylcyclopenta-1,3-diene;titanium(4+);dichloride | |
---|---|---|
Source | PubChem | |
URL | https://pubchem.ncbi.nlm.nih.gov | |
Description | Data deposited in or computed by PubChem | |
InChI |
InChI=1S/2C6H7.2ClH.Ti/c2*1-6-4-2-3-5-6;;;/h2*2-5H,1H3;2*1H;/q2*-1;;;+4/p-2 | |
Source | PubChem | |
URL | https://pubchem.ncbi.nlm.nih.gov | |
Description | Data deposited in or computed by PubChem | |
InChI Key |
NSBZPLSMZORBHY-UHFFFAOYSA-L | |
Source | PubChem | |
URL | https://pubchem.ncbi.nlm.nih.gov | |
Description | Data deposited in or computed by PubChem | |
Canonical SMILES |
CC1=CC=C[CH-]1.CC1=CC=C[CH-]1.[Cl-].[Cl-].[Ti+4] | |
Source | PubChem | |
URL | https://pubchem.ncbi.nlm.nih.gov | |
Description | Data deposited in or computed by PubChem | |
Molecular Formula |
C12H14Cl2Ti | |
Source | PubChem | |
URL | https://pubchem.ncbi.nlm.nih.gov | |
Description | Data deposited in or computed by PubChem | |
DSSTOX Substance ID |
DTXSID20926091 | |
Record name | Titanium(4+) chloride 2-methylcyclopenta-2,4-dien-1-ide (1/2/2) | |
Source | EPA DSSTox | |
URL | https://comptox.epa.gov/dashboard/DTXSID20926091 | |
Description | DSSTox provides a high quality public chemistry resource for supporting improved predictive toxicology. | |
Molecular Weight |
277.01 g/mol | |
Source | PubChem | |
URL | https://pubchem.ncbi.nlm.nih.gov | |
Description | Data deposited in or computed by PubChem | |
CAS No. |
1282-40-2 | |
Record name | Bis(methylcyclopentadienyl)titanium dichloride | |
Source | CAS Common Chemistry | |
URL | https://commonchemistry.cas.org/detail?cas_rn=1282-40-2 | |
Description | CAS Common Chemistry is an open community resource for accessing chemical information. Nearly 500,000 chemical substances from CAS REGISTRY cover areas of community interest, including common and frequently regulated chemicals, and those relevant to high school and undergraduate chemistry classes. This chemical information, curated by our expert scientists, is provided in alignment with our mission as a division of the American Chemical Society. | |
Explanation | The data from CAS Common Chemistry is provided under a CC-BY-NC 4.0 license, unless otherwise stated. | |
Record name | Titanium, dichlorobis(methyl-pi-cyclopentadienyl)- | |
Source | ChemIDplus | |
URL | https://pubchem.ncbi.nlm.nih.gov/substance/?source=chemidplus&sourceid=0001282402 | |
Description | ChemIDplus is a free, web search system that provides access to the structure and nomenclature authority files used for the identification of chemical substances cited in National Library of Medicine (NLM) databases, including the TOXNET system. | |
Record name | Titanium(4+) chloride 2-methylcyclopenta-2,4-dien-1-ide (1/2/2) | |
Source | EPA DSSTox | |
URL | https://comptox.epa.gov/dashboard/DTXSID20926091 | |
Description | DSSTox provides a high quality public chemistry resource for supporting improved predictive toxicology. | |
Haftungsausschluss und Informationen zu In-Vitro-Forschungsprodukten
Bitte beachten Sie, dass alle Artikel und Produktinformationen, die auf BenchChem präsentiert werden, ausschließlich zu Informationszwecken bestimmt sind. Die auf BenchChem zum Kauf angebotenen Produkte sind speziell für In-vitro-Studien konzipiert, die außerhalb lebender Organismen durchgeführt werden. In-vitro-Studien, abgeleitet von dem lateinischen Begriff "in Glas", beinhalten Experimente, die in kontrollierten Laborumgebungen unter Verwendung von Zellen oder Geweben durchgeführt werden. Es ist wichtig zu beachten, dass diese Produkte nicht als Arzneimittel oder Medikamente eingestuft sind und keine Zulassung der FDA für die Vorbeugung, Behandlung oder Heilung von medizinischen Zuständen, Beschwerden oder Krankheiten erhalten haben. Wir müssen betonen, dass jede Form der körperlichen Einführung dieser Produkte in Menschen oder Tiere gesetzlich strikt untersagt ist. Es ist unerlässlich, sich an diese Richtlinien zu halten, um die Einhaltung rechtlicher und ethischer Standards in Forschung und Experiment zu gewährleisten.