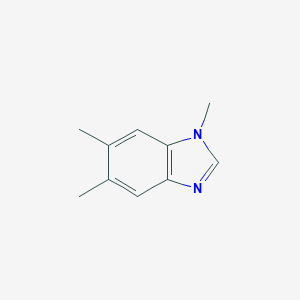
1,5,6-Trimethylbenzimidazole
Übersicht
Beschreibung
1,5,6-Trimethylbenzimidazole (TMB) is a heterocyclic organic compound that belongs to the benzimidazole family. TMB is widely used in scientific research as a ligand for metal ions, particularly cobalt and nickel. TMB is a versatile compound that has a wide range of applications in various fields of chemistry, including biochemistry, analytical chemistry, and materials science.
Safety and Hazards
In case of exposure, move the victim into fresh air and provide artificial respiration if necessary. If the chemical comes into contact with skin, remove contaminated clothing and wash off with soap and plenty of water. If it comes into contact with eyes, rinse with pure water for at least 15 minutes. If ingested, rinse mouth with water and do not induce vomiting .
Wirkmechanismus
Target of Action
1,5,6-Trimethylbenzimidazole (TMB) is a derivative of benzimidazole, a class of organic compounds that have been found to exhibit diverse pharmacological activities Benzimidazoles are known to interact with various biological targets, including enzymes, receptors, and proteins, depending on their chemical structure .
Mode of Action
Benzimidazoles, in general, are known to interact with their targets through various mechanisms, such as inhibition, activation, or modulation . The specific interaction of TMB with its target would depend on the chemical structure of TMB and the nature of the target.
Biochemical Pathways
Benzimidazoles are known to affect various biochemical pathways, depending on their targets . For instance, some benzimidazoles have been found to inhibit enzymes involved in critical biological processes, thereby affecting the corresponding biochemical pathways .
Pharmacokinetics
The pharmacokinetic properties of a compound are crucial in determining its bioavailability and therapeutic efficacy .
Result of Action
Based on the known activities of benzimidazoles, it can be inferred that tmb might exhibit antimicrobial, anticancer, antiviral, antiparasitic, antihypertensive, and anti-inflammatory activities .
Biochemische Analyse
Biochemical Properties
1,5,6-Trimethylbenzimidazole interacts with various biomolecules in biochemical reactions. It is part of the structure of certain coordination compounds, where it binds to the central metal atom . The nature of these interactions is largely determined by the electronic properties of the axial group in the compound .
Cellular Effects
Benzimidazole derivatives are known to have a broad spectrum of biological activities, including antimicrobial, antifungal, antiviral, antioxidant, anti-inflammatory, antidiabetic, antiparasitic, anthelmintic, anticoagulant, antiallergic, antiprotozoal, anticonvulsants, anticancer and cytotoxic activities .
Molecular Mechanism
Benzimidazole derivatives are known to interact with biopolymers of the living system due to their structural similarity to naturally occurring nucleotides . This interaction could potentially influence gene expression, enzyme activation or inhibition, and other molecular processes.
Metabolic Pathways
Benzimidazole derivatives are known to interact with various enzymes and cofactors , suggesting that this compound could potentially influence metabolic flux or metabolite levels.
Eigenschaften
IUPAC Name |
1,5,6-trimethylbenzimidazole | |
---|---|---|
Source | PubChem | |
URL | https://pubchem.ncbi.nlm.nih.gov | |
Description | Data deposited in or computed by PubChem | |
InChI |
InChI=1S/C10H12N2/c1-7-4-9-10(5-8(7)2)12(3)6-11-9/h4-6H,1-3H3 | |
Source | PubChem | |
URL | https://pubchem.ncbi.nlm.nih.gov | |
Description | Data deposited in or computed by PubChem | |
InChI Key |
LMAVJMARZPTUQH-UHFFFAOYSA-N | |
Source | PubChem | |
URL | https://pubchem.ncbi.nlm.nih.gov | |
Description | Data deposited in or computed by PubChem | |
Canonical SMILES |
CC1=CC2=C(C=C1C)N(C=N2)C | |
Source | PubChem | |
URL | https://pubchem.ncbi.nlm.nih.gov | |
Description | Data deposited in or computed by PubChem | |
Molecular Formula |
C10H12N2 | |
Source | PubChem | |
URL | https://pubchem.ncbi.nlm.nih.gov | |
Description | Data deposited in or computed by PubChem | |
Molecular Weight |
160.22 g/mol | |
Source | PubChem | |
URL | https://pubchem.ncbi.nlm.nih.gov | |
Description | Data deposited in or computed by PubChem | |
Synthesis routes and methods I
Procedure details
Synthesis routes and methods II
Procedure details
Haftungsausschluss und Informationen zu In-Vitro-Forschungsprodukten
Bitte beachten Sie, dass alle Artikel und Produktinformationen, die auf BenchChem präsentiert werden, ausschließlich zu Informationszwecken bestimmt sind. Die auf BenchChem zum Kauf angebotenen Produkte sind speziell für In-vitro-Studien konzipiert, die außerhalb lebender Organismen durchgeführt werden. In-vitro-Studien, abgeleitet von dem lateinischen Begriff "in Glas", beinhalten Experimente, die in kontrollierten Laborumgebungen unter Verwendung von Zellen oder Geweben durchgeführt werden. Es ist wichtig zu beachten, dass diese Produkte nicht als Arzneimittel oder Medikamente eingestuft sind und keine Zulassung der FDA für die Vorbeugung, Behandlung oder Heilung von medizinischen Zuständen, Beschwerden oder Krankheiten erhalten haben. Wir müssen betonen, dass jede Form der körperlichen Einführung dieser Produkte in Menschen oder Tiere gesetzlich strikt untersagt ist. Es ist unerlässlich, sich an diese Richtlinien zu halten, um die Einhaltung rechtlicher und ethischer Standards in Forschung und Experiment zu gewährleisten.