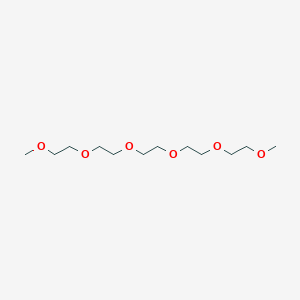
2,5,8,11,14,17-Hexaoxaoctadecane
Übersicht
Beschreibung
2,5,8,11,14,17-Hexaoxaoctadecane is a chemical compound with interesting properties and applications. It is part of a family of compounds known for their utility in various chemical reactions and processes.
Synthesis Analysis
The synthesis of compounds related to 2,5,8,11,14,17-Hexaoxaoctadecane, such as hexaazamacrocycles and hexaazatricyclooctadecane, involves complex chemical reactions. For instance, the synthesis of a dimeric hydroxobridged erbium(III) complex from hexacyclen, a related compound, demonstrates the intricate process of combining these molecules with metal ions under controlled conditions (Wang et al., 1998).
Molecular Structure Analysis
The molecular structure of these compounds is often complex and unique. The crystal structure determination of similar compounds, like chromium(III) complexes of hexaazamacrocycles, reveals distinct geometrical arrangements and coordination numbers (Chandrasekhar et al., 1993).
Chemical Reactions and Properties
Compounds like 2,5,8,11,14,17-Hexaoxaoctadecane exhibit interesting chemical reactions and properties, especially in their interaction with metal ions. Studies on related compounds show their ability to bind and extract metal ions, indicating their potential in applications like metal ion extraction and coordination chemistry (Lloris et al., 1998).
Physical Properties Analysis
The physical properties of these compounds, such as solubility, melting point, and crystalline structure, are influenced by their molecular makeup. For example, the crystal structure analysis of 1,4,7,10,13,16-hexaoxacyclooctadecane and its complexes provides insights into the physical characteristics of these compounds (Dunitz et al., 1974).
Chemical Properties Analysis
The chemical properties of 2,5,8,11,14,17-Hexaoxaoctadecane are characterized by its reactivity, stability, and interaction with other chemicals. Similar compounds have been shown to have significant reactivity and coordination abilities with various metal ions, highlighting their potential in chemical synthesis and applications (Aguilar et al., 1994).
Wissenschaftliche Forschungsanwendungen
Complexation with Metal Ions : 18-crown-6 forms stable complexes with various metal ions. It has been used for the extraction and separation of metal ions like silver(I), mercury(II), copper(II), platinum(II), and palladium(II) (Arpadjan, Mitewa, & Bontchev, 1987).
Solid-Liquid Equilibria Studies : The solubility of 18-crown-6 in various alcohols has been measured, providing insights into its physical properties and interactions in different solvents (Domańska & Venkatesu, 1998).
Macrocyclic Ligands Synthesis : The synthesis of macrocyclic ligands and their complexes, including those with 18-crown-6, has been explored for various applications in chemistry (Kılıç & Gündüz, 1986).
Biodegradation Studies : 18-crown-6 has been involved in studies related to the biodegradation of hydrocarbons like hexadecane, revealing insights into environmental remediation processes (Al-Hawash et al., 2018).
Environmental Chemistry : The compound has been used in studies related to environmental chemistry, such as the examination of the effects of biosurfactants on the biodegradation of hydrocarbons (Herman, Zhang, & Miller, 1997).
Molecular Structure Analysis : Studies on the molecular structure of complexes involving 18-crown-6 have provided insights into its coordination chemistry and potential applications (Motevalli, O’Brien, & Watson, 1996).
Thermodynamic Studies : Calorimetric studies have been conducted to understand the thermodynamics of complexes formed by 18-crown-6 with various ions, aiding in the comprehension of its binding properties and potential applications (Ozutsumi & Ishiguro, 1992).
Safety and Hazards
When handling 2,5,8,11,14,17-Hexaoxaoctadecane, avoid dust formation, breathing mist, gas or vapours, and contacting with skin and eye . Use personal protective equipment and wear chemical impermeable gloves . Ensure adequate ventilation, remove all sources of ignition, and evacuate personnel to safe areas . Keep people away from and upwind of spill/leak . Prevent further spillage or leakage if it is safe to do so . Do not let the chemical enter drains . Discharge into the environment must be avoided .
Wirkmechanismus
Target of Action
The primary targets of 2,5,8,11,14,17-Hexaoxaoctadecane are currently unknown . This compound, also known as Pentaethyleneglycol dimethyl ether , is a complex organic molecule with a molecular weight of 266.3312
Result of Action
The molecular and cellular effects of 2,5,8,11,14,17-Hexaoxaoctadecane’s action are currently unknown
Eigenschaften
IUPAC Name |
1-methoxy-2-[2-[2-[2-(2-methoxyethoxy)ethoxy]ethoxy]ethoxy]ethane | |
---|---|---|
Source | PubChem | |
URL | https://pubchem.ncbi.nlm.nih.gov | |
Description | Data deposited in or computed by PubChem | |
InChI |
InChI=1S/C12H26O6/c1-13-3-5-15-7-9-17-11-12-18-10-8-16-6-4-14-2/h3-12H2,1-2H3 | |
Source | PubChem | |
URL | https://pubchem.ncbi.nlm.nih.gov | |
Description | Data deposited in or computed by PubChem | |
InChI Key |
DMDPGPKXQDIQQG-UHFFFAOYSA-N | |
Source | PubChem | |
URL | https://pubchem.ncbi.nlm.nih.gov | |
Description | Data deposited in or computed by PubChem | |
Canonical SMILES |
COCCOCCOCCOCCOCCOC | |
Source | PubChem | |
URL | https://pubchem.ncbi.nlm.nih.gov | |
Description | Data deposited in or computed by PubChem | |
Molecular Formula |
C12H26O6 | |
Source | PubChem | |
URL | https://pubchem.ncbi.nlm.nih.gov | |
Description | Data deposited in or computed by PubChem | |
DSSTOX Substance ID |
DTXSID80152319 | |
Record name | 2,5,8,11,14,17-Hexaoxaoctadecane | |
Source | EPA DSSTox | |
URL | https://comptox.epa.gov/dashboard/DTXSID80152319 | |
Description | DSSTox provides a high quality public chemistry resource for supporting improved predictive toxicology. | |
Molecular Weight |
266.33 g/mol | |
Source | PubChem | |
URL | https://pubchem.ncbi.nlm.nih.gov | |
Description | Data deposited in or computed by PubChem | |
Product Name |
2,5,8,11,14,17-Hexaoxaoctadecane | |
CAS RN |
1191-87-3 | |
Record name | 2,5,8,11,14,17-Hexaoxaoctadecane | |
Source | CAS Common Chemistry | |
URL | https://commonchemistry.cas.org/detail?cas_rn=1191-87-3 | |
Description | CAS Common Chemistry is an open community resource for accessing chemical information. Nearly 500,000 chemical substances from CAS REGISTRY cover areas of community interest, including common and frequently regulated chemicals, and those relevant to high school and undergraduate chemistry classes. This chemical information, curated by our expert scientists, is provided in alignment with our mission as a division of the American Chemical Society. | |
Explanation | The data from CAS Common Chemistry is provided under a CC-BY-NC 4.0 license, unless otherwise stated. | |
Record name | 2,5,8,11,14,17-Hexaoxaoctadecane | |
Source | ChemIDplus | |
URL | https://pubchem.ncbi.nlm.nih.gov/substance/?source=chemidplus&sourceid=0001191873 | |
Description | ChemIDplus is a free, web search system that provides access to the structure and nomenclature authority files used for the identification of chemical substances cited in National Library of Medicine (NLM) databases, including the TOXNET system. | |
Record name | Pentaglyme | |
Source | DrugBank | |
URL | https://www.drugbank.ca/drugs/DB02580 | |
Description | The DrugBank database is a unique bioinformatics and cheminformatics resource that combines detailed drug (i.e. chemical, pharmacological and pharmaceutical) data with comprehensive drug target (i.e. sequence, structure, and pathway) information. | |
Explanation | Creative Common's Attribution-NonCommercial 4.0 International License (http://creativecommons.org/licenses/by-nc/4.0/legalcode) | |
Record name | 1191-87-3 | |
Source | DTP/NCI | |
URL | https://dtp.cancer.gov/dtpstandard/servlet/dwindex?searchtype=NSC&outputformat=html&searchlist=244990 | |
Description | The NCI Development Therapeutics Program (DTP) provides services and resources to the academic and private-sector research communities worldwide to facilitate the discovery and development of new cancer therapeutic agents. | |
Explanation | Unless otherwise indicated, all text within NCI products is free of copyright and may be reused without our permission. Credit the National Cancer Institute as the source. | |
Record name | 2,5,8,11,14,17-Hexaoxaoctadecane | |
Source | EPA DSSTox | |
URL | https://comptox.epa.gov/dashboard/DTXSID80152319 | |
Description | DSSTox provides a high quality public chemistry resource for supporting improved predictive toxicology. | |
Record name | 2,5,8,11,14,17-hexaoxaoctadecane | |
Source | European Chemicals Agency (ECHA) | |
URL | https://echa.europa.eu/substance-information/-/substanceinfo/100.013.403 | |
Description | The European Chemicals Agency (ECHA) is an agency of the European Union which is the driving force among regulatory authorities in implementing the EU's groundbreaking chemicals legislation for the benefit of human health and the environment as well as for innovation and competitiveness. | |
Explanation | Use of the information, documents and data from the ECHA website is subject to the terms and conditions of this Legal Notice, and subject to other binding limitations provided for under applicable law, the information, documents and data made available on the ECHA website may be reproduced, distributed and/or used, totally or in part, for non-commercial purposes provided that ECHA is acknowledged as the source: "Source: European Chemicals Agency, http://echa.europa.eu/". Such acknowledgement must be included in each copy of the material. ECHA permits and encourages organisations and individuals to create links to the ECHA website under the following cumulative conditions: Links can only be made to webpages that provide a link to the Legal Notice page. | |
Retrosynthesis Analysis
AI-Powered Synthesis Planning: Our tool employs the Template_relevance Pistachio, Template_relevance Bkms_metabolic, Template_relevance Pistachio_ringbreaker, Template_relevance Reaxys, Template_relevance Reaxys_biocatalysis model, leveraging a vast database of chemical reactions to predict feasible synthetic routes.
One-Step Synthesis Focus: Specifically designed for one-step synthesis, it provides concise and direct routes for your target compounds, streamlining the synthesis process.
Accurate Predictions: Utilizing the extensive PISTACHIO, BKMS_METABOLIC, PISTACHIO_RINGBREAKER, REAXYS, REAXYS_BIOCATALYSIS database, our tool offers high-accuracy predictions, reflecting the latest in chemical research and data.
Strategy Settings
Precursor scoring | Relevance Heuristic |
---|---|
Min. plausibility | 0.01 |
Model | Template_relevance |
Template Set | Pistachio/Bkms_metabolic/Pistachio_ringbreaker/Reaxys/Reaxys_biocatalysis |
Top-N result to add to graph | 6 |
Feasible Synthetic Routes
Q & A
Q1: How does 2,5,8,11,14,17-hexaoxaoctadecane interact with metal ions?
A1: 2,5,8,11,14,17-Hexaoxaoctadecane acts as a hexadentate ligand, meaning it can coordinate to metal ions through six oxygen atoms. This interaction is observed in the formation of complexes with alkaline earth metals like barium, strontium, and calcium. [, ] The oxygen atoms from the ether groups donate electron pairs to the metal ion, forming coordinate covalent bonds. This coordination can influence the reactivity and solubility of metal salts.
Q2: What is the typical coordination geometry observed in complexes of 2,5,8,11,14,17-hexaoxaoctadecane with metal ions like barium?
A2: In the complex bis(1,1,1,5,5,5-hexafluoro-2,4-pentanedionato)(2,5,8,11,14,17-hexaoxaoctadecane)barium, the 2,5,8,11,14,17-hexaoxaoctadecane coordinates to the barium ion in a meridional fashion. [] This means the ligand wraps around the metal ion, occupying one hemisphere of the coordination sphere. The two β-diketonate ligands then occupy opposite sides of the neutral ligand's mean plane, leading to a coordination number of 10 for the barium ion.
Q3: Can 2,5,8,11,14,17-hexaoxaoctadecane be incorporated into polymers, and if so, what properties does it impart?
A3: Yes, 2,5,8,11,14,17-hexaoxaoctadecane can be incorporated into polymers. [] Researchers have successfully synthesized hydrophilic polymers with both oligo(oxyethylene) moieties, similar to 2,5,8,11,14,17-hexaoxaoctadecane, and tertiary alcohol units in their main chain. These polymers exhibited good solubility in common solvents, likely attributed to the presence of the polar ether oxygen atoms from the incorporated 2,5,8,11,14,17-hexaoxaoctadecane units.
Haftungsausschluss und Informationen zu In-Vitro-Forschungsprodukten
Bitte beachten Sie, dass alle Artikel und Produktinformationen, die auf BenchChem präsentiert werden, ausschließlich zu Informationszwecken bestimmt sind. Die auf BenchChem zum Kauf angebotenen Produkte sind speziell für In-vitro-Studien konzipiert, die außerhalb lebender Organismen durchgeführt werden. In-vitro-Studien, abgeleitet von dem lateinischen Begriff "in Glas", beinhalten Experimente, die in kontrollierten Laborumgebungen unter Verwendung von Zellen oder Geweben durchgeführt werden. Es ist wichtig zu beachten, dass diese Produkte nicht als Arzneimittel oder Medikamente eingestuft sind und keine Zulassung der FDA für die Vorbeugung, Behandlung oder Heilung von medizinischen Zuständen, Beschwerden oder Krankheiten erhalten haben. Wir müssen betonen, dass jede Form der körperlichen Einführung dieser Produkte in Menschen oder Tiere gesetzlich strikt untersagt ist. Es ist unerlässlich, sich an diese Richtlinien zu halten, um die Einhaltung rechtlicher und ethischer Standards in Forschung und Experiment zu gewährleisten.