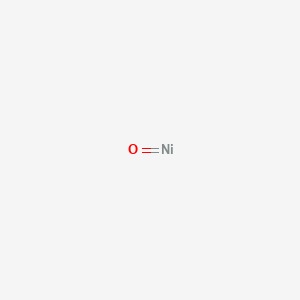
Nickel(II) oxide
- Klicken Sie auf QUICK INQUIRY, um ein Angebot von unserem Expertenteam zu erhalten.
- Mit qualitativ hochwertigen Produkten zu einem WETTBEWERBSFÄHIGEN Preis können Sie sich mehr auf Ihre Forschung konzentrieren.
Übersicht
Beschreibung
Nickel(II) oxide (NiO) is a chemical compound that is the principal oxide of nickel. It is classified as a basic metal oxide and is produced in several million kilograms annually, primarily as an intermediate in the production of nickel alloys .
Wirkmechanismus
Target of Action
Nickel oxide (NiO) is a p-type metal oxide that plays a significant role in various optoelectronic devices . The primary targets of NiO are the electrochromic materials in these devices . The widely accepted electrochromic mechanism of NiO involves the reversible conversion between colorless Ni 2+ and colored Ni 3+ and Ni 4+ .
Mode of Action
NiO interacts with its targets by undergoing a reversible conversion between its colorless (Ni 2+) and colored (Ni 3+ and Ni 4+) states . This interaction results in changes to the optical properties of the device, such as its color. The performance of NiO can be improved by optimizing the preparation parameters, which can adjust the morphology, crystal structure, and chemical state of the NiO film .
Biochemical Pathways
NiO toxicity can induce reactive oxygen species accumulation and oxidative stress responses in plants . It also reduces the phytohormone concentrations, including auxin, cytokinin, and gibberellic acid, thereby retarding plant growth . NiO toxicity alters the expression of genes involved in carbon/nitrogen metabolism pathways .
Pharmacokinetics
The pharmacokinetics of NiO nanoparticles (NPs) have been studied in the context of liver cancer treatment . NiO NPs are used as photosensitizers due to their significant bioavailability . HepG2 cells were incubated with different concentrations of NiO NPs ranging from 0-80μg/ml at 37 °C for 24 hours .
Result of Action
The result of NiO’s action depends on its application. In optoelectronic devices, NiO’s action results in dynamically adjustable optical properties, which can reduce building energy consumption . In a biological context, NiO toxicity can lead to organ damage by triggering downstream signaling through various pathways .
Action Environment
The action, efficacy, and stability of NiO can be influenced by environmental factors. For instance, NiO’s electrochromic performance can be enhanced by using appropriate modification strategies, such as surface modification, nanomaterialization, material compounding, doping, and electrolyte design . In a biological context, NiO’s toxicity can be influenced by factors such as the concentration of NiO NPs and the duration of exposure .
Biochemische Analyse
Biochemical Properties
Nickel oxide has been shown to interact with various biomolecules. For instance, a study on the ground beetle Blaps polychresta revealed that nickel oxide nanoparticles induced significant changes in aspartate aminotransferase (AST) and alanine aminotransferase (ALT) activities . These enzymes play crucial roles in amino acid metabolism, indicating that nickel oxide can influence biochemical reactions involving these enzymes .
Cellular Effects
Nickel oxide has been observed to have various effects on cells. In the aforementioned study, nickel oxide nanoparticles were found to cause histological alterations in the midgut tissues of the ground beetle . This suggests that nickel oxide can influence cell function and potentially impact cellular metabolism .
Molecular Mechanism
The molecular mechanism of nickel oxide’s action is complex and multifaceted. It appears to exert its effects at the molecular level through interactions with various biomolecules, leading to changes in enzyme activity and potentially influencing gene expression . The exact nature of these interactions and their consequences are still being explored.
Temporal Effects in Laboratory Settings
In laboratory settings, the effects of nickel oxide have been observed to change over time. For instance, the study on the ground beetle showed that the effects of nickel oxide nanoparticles on AST and ALT activities varied over a 30-day period . This suggests that nickel oxide may have long-term effects on cellular function .
Dosage Effects in Animal Models
The effects of nickel oxide also appear to vary with dosage. In the ground beetle study, different doses of nickel oxide nanoparticles were tested, and the sublethal dose of 0.02 mg/g was selected for further testing . This indicates that the effects of nickel oxide can be dose-dependent .
Metabolic Pathways
Its interaction with AST and ALT suggests that it may influence amino acid metabolism
Transport and Distribution
It is known that nickel oxide nanoparticles can be detected in midgut tissues, suggesting that they can be transported to and accumulate in specific tissues .
Subcellular Localization
The subcellular localization of nickel oxide is another area of active research. The presence of nickel oxide nanoparticles in midgut tissues suggests that they may be localized within specific cellular compartments .
Vorbereitungsmethoden
Nickel(II) oxide can be prepared through various methods:
Synthetic Routes and Reaction Conditions:
Industrial Production Methods:
Analyse Chemischer Reaktionen
Nickel(II) oxide undergoes various types of reactions:
Oxidation and Reduction:
Substitution: This compound reacts with the oxides of sodium and potassium at high temperatures to form corresponding nickelates.
Common Reagents and Conditions:
Major Products:
Wissenschaftliche Forschungsanwendungen
Biomedical Applications
Nickel(II) oxide nanoparticles (NiO NPs) have emerged as significant materials in biomedical applications due to their antimicrobial and anticancer properties. The biogenic synthesis of NiO NPs using plant extracts has been highlighted as a sustainable approach that avoids toxic chemicals. Key findings include:
- Antimicrobial Activity : NiO NPs exhibit strong antibacterial effects against pathogens like E. coli and S. aureus, making them promising candidates for medical coatings and drug delivery systems .
- Anticancer Properties : Studies indicate that NiO NPs can induce cytotoxic effects on cancer cells, providing potential therapeutic avenues for cancer treatment .
- Drug Delivery : The biocompatibility of NiO NPs allows their use in drug delivery systems, enhancing the efficacy of therapeutic agents .
Electrochemical Applications
NiO is extensively used in electrochemical applications, particularly in energy storage and conversion devices:
- Solid Oxide Fuel Cells (SOFCs) : NiO is utilized in the anode layer of SOFCs due to its excellent electrochemical properties and stability under operational conditions .
- Lithium-Ion Batteries : Doping NiO with lithium enhances its performance as a cathode material, improving energy density and cycle stability .
- Electrocatalysis : NiO serves as an effective electrocatalyst for various reactions, including oxygen evolution and hydrogen production, contributing to renewable energy technologies .
Ceramics and Glass Industry
In the ceramics sector, NiO plays a crucial role:
- Ceramic Glazes : It is used to produce colored glazes in porcelain and ceramic tiles, imparting vibrant colors while enhancing durability .
- Ferrites Production : NiO is a precursor for nickel ferrite (NiFe2O4), which is used in magnetic materials and electronic devices .
Environmental Applications
NiO's catalytic properties are leveraged for environmental protection:
- Pollutant Removal : NiO nanoparticles are effective in removing organic pollutants from wastewater through adsorption processes .
- Catalysts for Degradation Reactions : They are employed as catalysts in various chemical reactions aimed at degrading harmful substances in the environment .
Summary of Key Applications
Application Area | Specific Use Cases | Benefits |
---|---|---|
Biomedical | Antimicrobial coatings, drug delivery, anticancer agents | Biocompatible, effective against pathogens |
Electrochemistry | SOFCs, lithium-ion batteries, electrocatalysis | Enhanced energy storage and conversion |
Ceramics | Ceramic glazes, ferrites production | Aesthetic appeal, improved mechanical properties |
Environmental Protection | Pollutant removal, catalytic degradation | Sustainable remediation of contaminants |
Case Studies
- Antimicrobial Effects : Research demonstrated that NiO NPs synthesized from Moringa oleifera leaf extract showed significant antibacterial activity against various strains, suggesting their potential use in medical textiles and coatings .
- Electrochemical Performance : A study on lithium-doped nickel oxide indicated improvements in charge-discharge cycles when used as a cathode material in lithium-ion batteries, showcasing its viability for energy storage solutions .
- Ceramic Glaze Development : The incorporation of NiO into ceramic glazes not only enhanced color but also increased the thermal stability of the final products, making them suitable for high-temperature applications .
Vergleich Mit ähnlichen Verbindungen
Nickel(II) oxide can be compared with other similar compounds:
Nickel(III) Oxide (Ni2O3): A higher oxidation state of nickel oxide.
Nickel Hydroxide (Ni(OH)2): Used in nickel-cadmium and nickel-metal hydride batteries.
Nickel Carbonate (NiCO3): Another nickel compound that can be reduced to nickel oxide.
This compound’s diverse applications and unique properties make it a valuable compound in various scientific and industrial fields.
Biologische Aktivität
Nickel(II) oxide (NiO) is a compound that has garnered significant attention in recent years due to its diverse biological activities. This article will explore the biological properties of NiO, particularly focusing on its antibacterial, antioxidant, and anticancer activities. We will also present case studies and research findings to provide a comprehensive overview of its potential applications in biomedical fields.
Overview of this compound
This compound is an inorganic compound with the formula NiO. It appears as a green powder and is primarily used in ceramics, batteries, and as a catalyst. However, its nanoscale form, nickel oxide nanoparticles (NiO NPs), has shown promising biological activities, making it a subject of interest in nanomedicine.
Antibacterial Activity
Numerous studies have demonstrated the antibacterial properties of NiO NPs against various pathogens. The mechanism of action is primarily attributed to the generation of reactive oxygen species (ROS), which can damage bacterial cell membranes and DNA.
Research Findings
- Biosynthesized NiO NPs : A study highlighted that biosynthesized NiO NPs exhibited significant antibacterial activity against both Gram-positive and Gram-negative bacteria. The most susceptible strains included Bacillus licheniformis and Bacillus subtilis, while Klebsiella pneumoniae showed the least susceptibility .
- Mechanism of Action : The antibacterial action is believed to occur through the release of Ni²⁺ ions, which interfere with essential bacterial enzymes, leading to cell death. Additionally, ROS generated by NiO NPs disrupt vital cellular processes such as respiration and replication .
Table 1: Antibacterial Efficacy of NiO NPs
Bacterial Strain | Zone of Inhibition (mm) | Susceptibility Level |
---|---|---|
Bacillus licheniformis | 18 | High |
Bacillus subtilis | 16 | High |
Klebsiella pneumoniae | 10 | Low |
Pseudomonas aeruginosa | 14 | Moderate |
Methicillin-resistant Staphylococcus aureus (MRSA) | 12 | Moderate |
Antioxidant Activity
NiO NPs have been shown to exhibit significant antioxidant properties, which are crucial for combating oxidative stress in biological systems.
Case Study
In a recent study, the antioxidant activity of biosynthesized NiO NPs was evaluated using the DPPH assay. The results indicated that as the concentration of NiO NPs increased, their ability to scavenge free radicals also improved significantly. At a concentration of 100 μg/ml, the antioxidant activity reached 84%, which was comparable to standard antioxidants like L-ascorbic acid .
Table 2: Antioxidant Activity Comparison
Concentration (μg/ml) | Antioxidant Activity (%) |
---|---|
20 | 28 |
40 | 49 |
60 | 71 |
80 | 79 |
100 | 84 |
Anticancer Activity
Emerging research suggests that NiO NPs may have potential as anticancer agents due to their ability to induce apoptosis in cancer cells through ROS generation.
Research Insights
- Cell Line Studies : Studies have reported that NiO NPs exhibit cytotoxic effects against various cancer cell lines by promoting oxidative stress and apoptosis. The release of nickel ions can trigger apoptotic pathways in cancer cells .
- Mechanistic Understanding : The anticancer mechanism involves the interaction between Ni²⁺ ions and cellular components, leading to increased ROS levels that ultimately result in cell death .
Eigenschaften
CAS-Nummer |
1313-99-1 |
---|---|
Molekularformel |
NiO |
Molekulargewicht |
74.693 g/mol |
IUPAC-Name |
nickel(2+);oxygen(2-) |
InChI |
InChI=1S/Ni.O/q+2;-2 |
InChI-Schlüssel |
KVAWBJDCAFOHRG-UHFFFAOYSA-N |
Verunreinigungen |
Typical impurities, present at levels of 1% or less, include cobalt, copper, iron, and sulfur. |
SMILES |
O=[Ni] |
Kanonische SMILES |
[O-2].[Ni+2] |
Color/Form |
Green powder Greenish-black cubic crystals Yellow when hot |
Dichte |
6.67 (NTP, 1992) 6.72 6.7 g/cm³ |
melting_point |
3603 °F (NTP, 1992) 1955 °C |
Key on ui other cas no. |
1313-99-1 34492-97-2 11099-02-8 |
Physikalische Beschreibung |
Nickel oxide appears as odorless green-black cubic crystals (yellow when hot) or green powder. (NTP, 1992) DryPowder DryPowder; OtherSolid; PelletsLargeCrystals; WetSolid GREEN-TO-BLACK CRYSTALLINE POWDER. |
Piktogramme |
Irritant; Health Hazard; Environmental Hazard |
Löslichkeit |
less than 1 mg/mL at 68° F (NTP, 1992) 0.11 MG/100 ML @ 20 °C SOL IN ACIDS; AMMONIUM HYDROXIDE Insol in caustic solutions SOL IN POTASSIUM CYANIDE Insoluble in water; soluble in acids Solubility in water, mg/l at 20 °C: 1.1 (practically insoluble) |
Synonyme |
nickel monoxide nickel oxide nickel(II)oxide |
Dampfdruck |
0 mm Hg at 68 °F (NTP, 1992) |
Herkunft des Produkts |
United States |
Haftungsausschluss und Informationen zu In-Vitro-Forschungsprodukten
Bitte beachten Sie, dass alle Artikel und Produktinformationen, die auf BenchChem präsentiert werden, ausschließlich zu Informationszwecken bestimmt sind. Die auf BenchChem zum Kauf angebotenen Produkte sind speziell für In-vitro-Studien konzipiert, die außerhalb lebender Organismen durchgeführt werden. In-vitro-Studien, abgeleitet von dem lateinischen Begriff "in Glas", beinhalten Experimente, die in kontrollierten Laborumgebungen unter Verwendung von Zellen oder Geweben durchgeführt werden. Es ist wichtig zu beachten, dass diese Produkte nicht als Arzneimittel oder Medikamente eingestuft sind und keine Zulassung der FDA für die Vorbeugung, Behandlung oder Heilung von medizinischen Zuständen, Beschwerden oder Krankheiten erhalten haben. Wir müssen betonen, dass jede Form der körperlichen Einführung dieser Produkte in Menschen oder Tiere gesetzlich strikt untersagt ist. Es ist unerlässlich, sich an diese Richtlinien zu halten, um die Einhaltung rechtlicher und ethischer Standards in Forschung und Experiment zu gewährleisten.