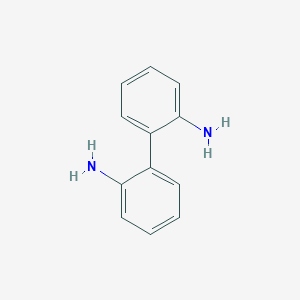
2,2'-Biphenyldiamine
Übersicht
Beschreibung
2,2'-Biphenyldiamine (CAS 1454-80-4) is an aromatic diamine characterized by two amine groups positioned at the 2 and 2' positions of a biphenyl backbone. This structural arrangement imparts rigidity and planarity, making it a critical building block in catalysis and polymer chemistry. Key applications include:
- Catalysis: Derivatives of this compound, such as the Grubbs third-generation catalyst modified with this ligand, enhance stability and percent conversion (up to 40% equilibrium yield) in ring-closing metathesis (RCM) reactions for DNA-encoded libraries .
- Polymers: It serves as a monomer in polyimide-based polymers of intrinsic microporosity (PIMs), contributing to high CO₂ sorption capacity and microporous structures .
- Electrocatalysis: In covalent organic frameworks (COFs), this compound-based materials exhibit superior conductivity (3.7 × 10⁻³ S m⁻¹) and CO₂-to-CO Faradaic efficiency (87–97%) in electrocatalytic reduction .
Vorbereitungsmethoden
Synthetic Routes and Reaction Conditions: The synthesis of 2,2’-Biphenyldiamine typically involves the following steps:
Starting Material: Aniline is used as the starting material.
Bromination: Aniline is brominated under weak acidic conditions, such as in acetic acid.
Coupling Reaction: The brominated product undergoes a coupling reaction to form the biphenyl structure.
Purification: The final product is purified through distillation to remove unreacted aniline and by-products.
Industrial Production Methods: In industrial settings, the production of 2,2’-Biphenyldiamine follows similar synthetic routes but on a larger scale. The process involves:
Large-scale bromination: of aniline.
Efficient coupling reactions: using catalysts to enhance yield.
Advanced purification techniques: such as recrystallization and chromatography to ensure high purity of the final product.
Analyse Chemischer Reaktionen
Types of Reactions: 2,2’-Biphenyldiamine undergoes various chemical reactions, including:
Oxidation: It can be oxidized to form quinonoid structures.
Reduction: The compound can be reduced to form corresponding amines.
Substitution: It participates in electrophilic substitution reactions due to the presence of amino groups.
Common Reagents and Conditions:
Oxidizing Agents: Potassium permanganate (KMnO4) or hydrogen peroxide (H2O2) for oxidation reactions.
Reducing Agents: Sodium borohydride (NaBH4) or lithium aluminum hydride (LiAlH4) for reduction reactions.
Catalysts: Palladium on carbon (Pd/C) for hydrogenation reactions.
Major Products:
Oxidation Products: Quinonoid derivatives.
Reduction Products: Corresponding amines.
Substitution Products: Various substituted biphenyl derivatives depending on the electrophile used.
Wissenschaftliche Forschungsanwendungen
2,2’-Biphenyldiamine has diverse applications in scientific research:
Chemistry: Used as an intermediate in the synthesis of dyes and pigments.
Biology: Investigated for its potential as a building block in the synthesis of biologically active molecules.
Medicine: Explored for its role in the development of pharmaceutical compounds with potential therapeutic effects.
Industry: Utilized in the production of rubber antioxidants to enhance the durability and longevity of rubber products
Wirkmechanismus
The mechanism of action of 2,2’-Biphenyldiamine involves its interaction with various molecular targets:
Enzyme Inhibition: It can inhibit specific enzymes by binding to their active sites.
Signal Transduction Pathways: The compound may interfere with cellular signaling pathways, affecting cell function and behavior.
Molecular Interactions: Its amino groups allow it to form hydrogen bonds and other interactions with biological molecules, influencing their activity.
Vergleich Mit ähnlichen Verbindungen
Comparison with Structural Analogs
Positional Isomers: 2,4'-Biphenyldiamine vs. 4,4'-Biphenyldiamine
Key Findings :
- 2,4'-Biphenyldiamine requires complex reduction steps for brominated derivatives (e.g., 5-bromo-2,4'-biphenyldiamine) due to competing azobenzene reduction .
- 4,4'-Biphenyldiamine -based COFs exhibit reduced conductivity compared to 2,2'-derivatives, highlighting the impact of amine positioning on electron transport .
Halogenated Derivatives: Brominated and Fluorinated Analogs
Key Findings :
- TFMB reduces melt viscosity in PMR-II polyimides by 30% while retaining thermal stability, enabling high-temperature aerospace applications .
- Octafluoro derivatives exhibit exceptional chemical resistance, critical for harsh-environment materials .
Methyl-Substituted Derivatives
Key Findings :
- 4-Methyl-2,2'-biphenyldiamine in Ru complexes lowers MLCT band energy by 0.2 eV compared to unsubstituted analogs, improving photosensitizing efficiency .
- 6,6'-Dimethyl derivative enables chiral separations with 90% enantiomeric excess in amino acid analysis .
Functional Comparison in Catalysis and Materials
Catalytic Performance
Catalyst System | Substrate Conversion | Stability | Reference |
---|---|---|---|
Grubbs A (unmodified) | 50–60% | Prone to isomerization | |
Grubbs B (this compound) | 85–90% | Resists decomposition at RT |
Polymer Properties
Polymer Type | CO₂ Sorption (mmol g⁻¹) | Conductivity (S m⁻¹) | Thermal Stability (°C) |
---|---|---|---|
PIM-AUC-5 (this compound) | 1.8 | 3.7 × 10⁻³ | 300 |
PIM-AUC-6 (benzidine) | 1.5 | 1.6 × 10⁻³ | 280 |
Key Insight : The rigid biphenyl spacer in this compound enhances microporosity and charge transport in polymers .
Biologische Aktivität
2,2'-Biphenyldiamine (BPDA), also known as benzidine or o-dianisidine, is an aromatic organic compound with the molecular formula . Its structure features two amino groups attached to a biphenyl backbone, which influences its chemical reactivity and biological activity. This article explores the biological activity of this compound, focusing on its biochemical properties, cellular effects, mechanisms of action, and potential applications in various fields.
This compound exhibits several significant biochemical properties:
- Solubility : It is soluble in organic solvents and demonstrates some solubility in water due to its amino groups.
- Reactivity : The presence of amine groups allows for hydrogen bonding and nucleophilic reactions with electrophiles.
- Stability : BPDA is thermally unstable and can decompose upon heating, leading to various products including aniline and ammonia.
Cellular Effects
Research indicates that this compound can influence cellular functions through:
- Cell Signaling : It may modulate cell signaling pathways, affecting processes such as proliferation and apoptosis.
- Gene Expression : BPDA has been shown to alter gene expression profiles in various cell types, potentially impacting metabolic pathways.
- Metabolic Interactions : It interacts with enzymes involved in metabolic processes, affecting the flux of metabolites within cells .
The mechanisms through which this compound exerts its biological effects include:
- Enzyme Inhibition : BPDA can inhibit specific enzymes by binding to their active sites. This inhibition can disrupt normal cellular functions and lead to cytotoxic effects .
- Signal Transduction Interference : The compound may interfere with signal transduction pathways by binding to key proteins involved in these pathways.
- Molecular Interactions : Its amino groups facilitate interactions with various biomolecules, influencing their activities through hydrogen bonding and other non-covalent interactions .
Biological Activity Data
The following table summarizes key biological activities associated with this compound:
Safety and Toxicity
It is crucial to note that this compound is classified as a human carcinogen. Prolonged exposure has been linked to bladder cancer and other health risks. Due to these hazards, its industrial use has significantly declined . Safety protocols must be strictly followed when handling this compound in laboratory settings.
Q & A
Q. What are the key synthetic routes for 2,2'-biphenyldiamine derivatives in advanced materials design?
Basic
Synthesis of this compound derivatives often involves coupling reactions, such as Ullmann or Suzuki-Miyaura cross-coupling, to introduce functional groups (e.g., hydroxyl, nitrile, or methyl substituents). For example, 6,6'-dihydroxy-2,2'-biphenyldiamine (DHBIPDA) is synthesized via oxidative coupling of phenol derivatives, enabling the creation of microporous polymer membranes for solvent-resistant nanofiltration . Enzymatic approaches using Laccase have also been reported for coupling p-chloroaniline to form 4,4’-biphenyldiamine, though yields and selectivity require optimization .
Advanced
Advanced routes leverage regioselective functionalization. For instance, 4-methyl-2,2'-biphenyldiamine derivatives are synthesized via ligand substitution in ruthenium polypyridyl complexes. This involves replacing bipyridine (bpy) ligands with methyl- and nitrile-substituted analogs (Mebpy-CN), which stabilize charge-transfer states in photodynamic applications . Computational modeling (DFT) aids in predicting substituent effects on electronic properties prior to synthesis .
Q. How do substituents on this compound influence charge-transfer properties in metal complexes?
Basic
Substituents like nitrile (–CN) or methyl (–CH₃) groups alter electron density distribution. Nitrile groups act as electron-withdrawing moieties, lowering MLCT (metal-to-ligand charge transfer) band energies in ruthenium complexes, as observed in redshifted absorption spectra (e.g., λₘₐₓ = 454–473 nm) . Methyl groups partially counteract this effect by donating electrons, balancing redox potentials .
Advanced
Substituent-induced electronic delocalization can be quantified via DFT and spectroelectrochemical studies. For example, nitrile substituents in [Ru(bpy)₂(Mebpy-CN)]²⁺ complexes enhance LUMO (ligand-centered) delocalization, reducing reorganization energy (λ = 0.60 eV) and stabilizing charge-separated states for prolonged photoluminescence (τ = 1.2–2.3 μs) . Electrochemical data (e.g., E₁/₂ redox potentials) correlate linearly with MLCT band positions, enabling predictive tuning of photosensitizer efficiency .
Q. What computational methods are used to predict the photophysical behavior of this compound-based complexes?
Basic
Density functional theory (DFT) and time-dependent DFT (TD-DFT) are standard for modeling ground- and excited-state properties. These methods calculate HOMO/LUMO energies, spin density distributions, and MLCT transitions. For instance, DFT predicts nitrile-induced stabilization of Ru(II) oxidation states in Mebpy-CN complexes, aligning with cyclic voltammetry data .
Advanced
Advanced workflows combine DFT with Marcus theory to analyze electron-transfer kinetics. Parameters like electronic coupling (HAB), reorganization energy (λ), and delocalization factor (α²) are derived from UV-Vis and emission spectra. For example, α² = 1.0×10⁻³ in mixed-valence Ru complexes indicates enhanced delocalization, reducing charge recombination rates and improving photosensitizing efficiency . Transient absorption spectroscopy further validates computational predictions of triplet-state lifetimes .
Q. How is this compound utilized in microporous polymer membranes for molecular separation?
Basic
this compound derivatives like DHBIPDA are polymerized via interfacial polymerization to form ultrathin films (<50 nm) with sub-nanometer pores. These membranes achieve >90% rejection of organic dyes (e.g., Rose Bengal) in organic solvents, attributed to size exclusion and π-π interactions .
Advanced
Pore-size distribution and solvent resistance are optimized by tuning monomer rigidity and crosslinking density. For example, β-ketoenamine covalent organic frameworks (COFs) incorporating this compound exhibit adjustable microporosity (BET surface area: 400–600 m²/g) for selective CO₂ adsorption . Gas permeation data correlate with simulated pore geometries, validated via grand canonical Monte Carlo (GCMC) simulations .
Q. What methodologies assess the antioxidant and bioactivity of this compound derivatives?
Basic
Antioxidant capacity is quantified via oxygen radical absorbance capacity (ORAC) assays. For example, 4,4’-biphenyldiamine synthesized via Laccase catalysis shows ORAC values 20% higher than p-chloroaniline, attributed to enhanced radical stabilization by conjugated biphenyl structures . Antifungal activity against Botrytis cinerea is evaluated using microdilution assays (MIC = 50–100 µg/mL) .
Advanced
Mechanistic studies combine electron paramagnetic resonance (EPR) and fluorescence quenching to identify reactive oxygen species (ROS) scavenging pathways. For Ru complexes, singlet oxygen (¹O₂) generation is monitored via time-resolved near-infrared phosphorescence (λₑₘ = 1270 nm), revealing ¹O₂ quantum yields (ΦΔ) inversely proportional to ligand substitution (e.g., ΦΔ = 0.45 for [Ru(bpy)₃]²⁺ vs. 0.25 for Mebpy-CN analogs) .
Q. How do structural variations in this compound impact enantioselective separation?
Advanced
Chiral 6,6'-dimethyl-2,2'-biphenyldiamine derivatives serve as axially chiral derivatizing agents for amino acid resolution. Their enantioselectivity arises from steric and electronic interactions in capillary electrophoresis (CE) or HPLC. For example, derivatives with bulky substituents achieve resolution factors (Rs) >2.0 for D/L-amino acids, validated via mass spectrometric detection . Molecular docking simulations predict chiral recognition mechanisms, guiding reagent design .
Q. What safety protocols are critical for handling halogenated this compound derivatives?
Basic
Halogenated derivatives (e.g., 4,4'-dibromo-2,2'-biphenyldiamine) require strict PPE (gloves, goggles) due to acute oral toxicity (LD₅₀ = 300 mg/kg) and environmental hazards. Emergency measures include decontamination with soap/water for skin contact and artificial respiration for inhalation exposure .
Advanced
Environmental persistence is assessed via OECD 301 biodegradability tests. Brominated derivatives exhibit high bioaccumulation potential (log Kow >4.5), necessitating waste treatment via incineration with alkaline scrubbers to prevent dioxin formation .
Eigenschaften
IUPAC Name |
2-(2-aminophenyl)aniline | |
---|---|---|
Source | PubChem | |
URL | https://pubchem.ncbi.nlm.nih.gov | |
Description | Data deposited in or computed by PubChem | |
InChI |
InChI=1S/C12H12N2/c13-11-7-3-1-5-9(11)10-6-2-4-8-12(10)14/h1-8H,13-14H2 | |
Source | PubChem | |
URL | https://pubchem.ncbi.nlm.nih.gov | |
Description | Data deposited in or computed by PubChem | |
InChI Key |
HOLGXWDGCVTMTB-UHFFFAOYSA-N | |
Source | PubChem | |
URL | https://pubchem.ncbi.nlm.nih.gov | |
Description | Data deposited in or computed by PubChem | |
Canonical SMILES |
C1=CC=C(C(=C1)C2=CC=CC=C2N)N | |
Source | PubChem | |
URL | https://pubchem.ncbi.nlm.nih.gov | |
Description | Data deposited in or computed by PubChem | |
Molecular Formula |
C12H12N2 | |
Source | PubChem | |
URL | https://pubchem.ncbi.nlm.nih.gov | |
Description | Data deposited in or computed by PubChem | |
DSSTOX Substance ID |
DTXSID00163003 | |
Record name | 2,2'-Biphenyldiamine | |
Source | EPA DSSTox | |
URL | https://comptox.epa.gov/dashboard/DTXSID00163003 | |
Description | DSSTox provides a high quality public chemistry resource for supporting improved predictive toxicology. | |
Molecular Weight |
184.24 g/mol | |
Source | PubChem | |
URL | https://pubchem.ncbi.nlm.nih.gov | |
Description | Data deposited in or computed by PubChem | |
CAS No. |
1454-80-4 | |
Record name | 2,2′-Diaminobiphenyl | |
Source | CAS Common Chemistry | |
URL | https://commonchemistry.cas.org/detail?cas_rn=1454-80-4 | |
Description | CAS Common Chemistry is an open community resource for accessing chemical information. Nearly 500,000 chemical substances from CAS REGISTRY cover areas of community interest, including common and frequently regulated chemicals, and those relevant to high school and undergraduate chemistry classes. This chemical information, curated by our expert scientists, is provided in alignment with our mission as a division of the American Chemical Society. | |
Explanation | The data from CAS Common Chemistry is provided under a CC-BY-NC 4.0 license, unless otherwise stated. | |
Record name | 2,2'-Biphenyldiamine | |
Source | ChemIDplus | |
URL | https://pubchem.ncbi.nlm.nih.gov/substance/?source=chemidplus&sourceid=0001454804 | |
Description | ChemIDplus is a free, web search system that provides access to the structure and nomenclature authority files used for the identification of chemical substances cited in National Library of Medicine (NLM) databases, including the TOXNET system. | |
Record name | 2,2'-Diaminobiphenyl | |
Source | DTP/NCI | |
URL | https://dtp.cancer.gov/dtpstandard/servlet/dwindex?searchtype=NSC&outputformat=html&searchlist=99367 | |
Description | The NCI Development Therapeutics Program (DTP) provides services and resources to the academic and private-sector research communities worldwide to facilitate the discovery and development of new cancer therapeutic agents. | |
Explanation | Unless otherwise indicated, all text within NCI products is free of copyright and may be reused without our permission. Credit the National Cancer Institute as the source. | |
Record name | 2,2'-Biphenyldiamine | |
Source | EPA DSSTox | |
URL | https://comptox.epa.gov/dashboard/DTXSID00163003 | |
Description | DSSTox provides a high quality public chemistry resource for supporting improved predictive toxicology. | |
Record name | 2,2'-Biphenyldiamine | |
Source | European Chemicals Agency (ECHA) | |
URL | https://echa.europa.eu/information-on-chemicals | |
Description | The European Chemicals Agency (ECHA) is an agency of the European Union which is the driving force among regulatory authorities in implementing the EU's groundbreaking chemicals legislation for the benefit of human health and the environment as well as for innovation and competitiveness. | |
Explanation | Use of the information, documents and data from the ECHA website is subject to the terms and conditions of this Legal Notice, and subject to other binding limitations provided for under applicable law, the information, documents and data made available on the ECHA website may be reproduced, distributed and/or used, totally or in part, for non-commercial purposes provided that ECHA is acknowledged as the source: "Source: European Chemicals Agency, http://echa.europa.eu/". Such acknowledgement must be included in each copy of the material. ECHA permits and encourages organisations and individuals to create links to the ECHA website under the following cumulative conditions: Links can only be made to webpages that provide a link to the Legal Notice page. | |
Record name | 2,2'-BIPHENYLDIAMINE | |
Source | FDA Global Substance Registration System (GSRS) | |
URL | https://gsrs.ncats.nih.gov/ginas/app/beta/substances/0DJ0J26MNW | |
Description | The FDA Global Substance Registration System (GSRS) enables the efficient and accurate exchange of information on what substances are in regulated products. Instead of relying on names, which vary across regulatory domains, countries, and regions, the GSRS knowledge base makes it possible for substances to be defined by standardized, scientific descriptions. | |
Explanation | Unless otherwise noted, the contents of the FDA website (www.fda.gov), both text and graphics, are not copyrighted. They are in the public domain and may be republished, reprinted and otherwise used freely by anyone without the need to obtain permission from FDA. Credit to the U.S. Food and Drug Administration as the source is appreciated but not required. | |
Synthesis routes and methods
Procedure details
Haftungsausschluss und Informationen zu In-Vitro-Forschungsprodukten
Bitte beachten Sie, dass alle Artikel und Produktinformationen, die auf BenchChem präsentiert werden, ausschließlich zu Informationszwecken bestimmt sind. Die auf BenchChem zum Kauf angebotenen Produkte sind speziell für In-vitro-Studien konzipiert, die außerhalb lebender Organismen durchgeführt werden. In-vitro-Studien, abgeleitet von dem lateinischen Begriff "in Glas", beinhalten Experimente, die in kontrollierten Laborumgebungen unter Verwendung von Zellen oder Geweben durchgeführt werden. Es ist wichtig zu beachten, dass diese Produkte nicht als Arzneimittel oder Medikamente eingestuft sind und keine Zulassung der FDA für die Vorbeugung, Behandlung oder Heilung von medizinischen Zuständen, Beschwerden oder Krankheiten erhalten haben. Wir müssen betonen, dass jede Form der körperlichen Einführung dieser Produkte in Menschen oder Tiere gesetzlich strikt untersagt ist. Es ist unerlässlich, sich an diese Richtlinien zu halten, um die Einhaltung rechtlicher und ethischer Standards in Forschung und Experiment zu gewährleisten.