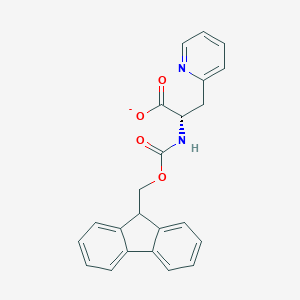
Fmoc-L-2-Pyridylalanin
Übersicht
Beschreibung
Fmoc-L-2-Pyridylalanine is a derivative of the amino acid alanine, modified with a fluorenylmethyloxycarbonyl (Fmoc) protecting group and a pyridyl group. It plays a crucial role in the development of new drugs and the study of protein structure and function .
Wissenschaftliche Forschungsanwendungen
Fmoc-L-2-Pyridylalanine has a wide range of applications in scientific research:
Wirkmechanismus
Target of Action
Fmoc-L-2-Pyridylalanine, also known as Fmoc-2-Pal-OH, is primarily used in peptide synthesis and proteomics research . It is an alanine derivative , which suggests that it may interact with proteins or enzymes that recognize or process alanine residues.
Mode of Action
As an alanine derivative , it may be incorporated into peptides during synthesis, potentially altering the properties of the resulting peptide. The fluorenylmethyloxycarbonyl (Fmoc) group is a common protecting group used in peptide synthesis, which can be removed under basic conditions to reveal the free amine group of the amino acid residue.
Vorbereitungsmethoden
Synthetic Routes and Reaction Conditions: The synthesis of Fmoc-L-2-Pyridylalanine typically involves a multi-step reaction process. One common method starts with the precursor Fmoc-serine methyl ester. The steps include:
Protection of the amino group: The amino group of serine is protected using the Fmoc group.
Formation of the pyridyl group: The protected serine is then reacted with 2-bromopyridine to introduce the pyridyl group.
Deprotection and purification: The final product is obtained by deprotecting the intermediate and purifying the compound.
Industrial Production Methods: Industrial production of Fmoc-L-2-Pyridylalanine follows similar synthetic routes but on a larger scale. The process involves optimizing reaction conditions to maximize yield and purity. Key factors include temperature control, reaction time, and the use of high-purity reagents .
Analyse Chemischer Reaktionen
Types of Reactions: Fmoc-L-2-Pyridylalanine undergoes various chemical reactions, including:
Oxidation: The pyridyl group can be oxidized under specific conditions.
Reduction: The compound can be reduced to modify the pyridyl group.
Substitution: The Fmoc group can be substituted with other protecting groups or functional groups.
Common Reagents and Conditions:
Oxidation: Common oxidizing agents include hydrogen peroxide and potassium permanganate.
Reduction: Reducing agents such as sodium borohydride and lithium aluminum hydride are used.
Substitution: Substitution reactions often involve bases like piperidine for Fmoc deprotection.
Major Products: The major products formed from these reactions depend on the specific conditions and reagents used. For example, oxidation of the pyridyl group can lead to the formation of pyridine N-oxide derivatives .
Vergleich Mit ähnlichen Verbindungen
Fmoc-L-3-Pyridylalanine: Similar to Fmoc-L-2-Pyridylalanine but with the pyridyl group at the 3-position.
Fmoc-L-4-Pyridylalanine: Has the pyridyl group at the 4-position.
Fmoc-D-2-Pyridylalanine: The D-enantiomer of Fmoc-L-2-Pyridylalanine.
Uniqueness: Fmoc-L-2-Pyridylalanine is unique due to the specific positioning of the pyridyl group, which can influence the reactivity and interactions of the compound. This positioning allows for distinct hydrogen bonding patterns and π-π interactions, making it a valuable tool in peptide synthesis and drug development .
Biologische Aktivität
Fmoc-L-2-Pyridylalanine (Fmoc-L-2-Pya) is a non-natural amino acid that has garnered attention in biochemical research due to its unique structural properties and potential biological applications. This article provides a comprehensive overview of its biological activity, including synthesis methods, structural characteristics, and its role in various biochemical contexts.
Structural Characteristics
Fmoc-L-2-Pya is characterized by the presence of a pyridyl group at the 2-position of the alanine side chain, which contributes to its distinct chemical properties. The Fmoc (9-fluorenylmethoxycarbonyl) protecting group enhances its stability during synthesis and facilitates incorporation into peptides.
Table 1: Structural Properties of Fmoc-L-2-Pyridylalanine
Property | Value |
---|---|
Molecular Formula | C₁₈H₁₉N₂O₄ |
Molecular Weight | 337.36 g/mol |
Melting Point | 160-162 °C |
Solubility | Soluble in DMSO, DMF |
Synthesis Methods
The synthesis of Fmoc-L-2-Pya typically involves solid-phase peptide synthesis (SPPS), where the amino acid is incorporated into peptide chains. The incorporation of 2-pyridylalanine has been shown to enhance the binding affinity of peptides to target proteins, such as antibodies.
Case Study: IgG-Binding Peptides
A notable study demonstrated that substituting histidine with 2-pyridylalanine in an IgG-binding peptide resulted in a significant increase in binding affinity. The modified peptide showed improved coupling efficiency and reduced by-product formation during synthesis, indicating that Fmoc-L-2-Pya can enhance the performance of peptide-based therapeutics .
Antimicrobial Properties
Research has indicated that derivatives of pyridylalanines, including Fmoc-L-2-Pya, exhibit antimicrobial activity. These compounds can act as inhibitors of elastase, an enzyme involved in various infections, including those related to cystic fibrosis . The incorporation of 2-pyridylalanine into peptide sequences may enhance their efficacy against bacterial pathogens.
Self-Assembly and Hydrogel Formation
Fmoc-L-2-Pya has been shown to participate in self-assembly processes, forming supramolecular structures that can lead to hydrogel formation. These hydrogels have potential applications in drug delivery systems and tissue engineering due to their biocompatibility and ability to mimic extracellular matrices .
Circular Dichroism Studies
Circular dichroism (CD) spectroscopy has been employed to study the conformational properties of Fmoc-L-2-Pya in solution. The results indicate that this compound exhibits distinct chiral properties, which are crucial for its biological function. The CD spectra confirmed the formation of helical structures, which are essential for the stability and activity of peptide assemblies .
Mechanical Properties
Studies on the mechanical properties of hydrogels formed from Fmoc-L-2-Pya have revealed a Young's modulus of approximately 14.1 GPa, indicating its potential for use in applications requiring specific mechanical characteristics . This property is particularly relevant for designing biomaterials that need to withstand physiological stresses.
Eigenschaften
IUPAC Name |
(2S)-2-(9H-fluoren-9-ylmethoxycarbonylamino)-3-pyridin-2-ylpropanoic acid | |
---|---|---|
Source | PubChem | |
URL | https://pubchem.ncbi.nlm.nih.gov | |
Description | Data deposited in or computed by PubChem | |
InChI |
InChI=1S/C23H20N2O4/c26-22(27)21(13-15-7-5-6-12-24-15)25-23(28)29-14-20-18-10-3-1-8-16(18)17-9-2-4-11-19(17)20/h1-12,20-21H,13-14H2,(H,25,28)(H,26,27)/t21-/m0/s1 | |
Source | PubChem | |
URL | https://pubchem.ncbi.nlm.nih.gov | |
Description | Data deposited in or computed by PubChem | |
InChI Key |
DXIVJCDZOMUITR-NRFANRHFSA-N | |
Source | PubChem | |
URL | https://pubchem.ncbi.nlm.nih.gov | |
Description | Data deposited in or computed by PubChem | |
Canonical SMILES |
C1=CC=C2C(=C1)C(C3=CC=CC=C32)COC(=O)NC(CC4=CC=CC=N4)C(=O)O | |
Source | PubChem | |
URL | https://pubchem.ncbi.nlm.nih.gov | |
Description | Data deposited in or computed by PubChem | |
Isomeric SMILES |
C1=CC=C2C(=C1)C(C3=CC=CC=C32)COC(=O)N[C@@H](CC4=CC=CC=N4)C(=O)O | |
Source | PubChem | |
URL | https://pubchem.ncbi.nlm.nih.gov | |
Description | Data deposited in or computed by PubChem | |
Molecular Formula |
C23H20N2O4 | |
Source | PubChem | |
URL | https://pubchem.ncbi.nlm.nih.gov | |
Description | Data deposited in or computed by PubChem | |
Molecular Weight |
388.4 g/mol | |
Source | PubChem | |
URL | https://pubchem.ncbi.nlm.nih.gov | |
Description | Data deposited in or computed by PubChem | |
CAS No. |
185379-40-2 | |
Record name | (αS)-α-[[(9H-Fluoren-9-ylmethoxy)carbonyl]amino]-2-pyridinepropanoic acid | |
Source | CAS Common Chemistry | |
URL | https://commonchemistry.cas.org/detail?cas_rn=185379-40-2 | |
Description | CAS Common Chemistry is an open community resource for accessing chemical information. Nearly 500,000 chemical substances from CAS REGISTRY cover areas of community interest, including common and frequently regulated chemicals, and those relevant to high school and undergraduate chemistry classes. This chemical information, curated by our expert scientists, is provided in alignment with our mission as a division of the American Chemical Society. | |
Explanation | The data from CAS Common Chemistry is provided under a CC-BY-NC 4.0 license, unless otherwise stated. | |
Haftungsausschluss und Informationen zu In-Vitro-Forschungsprodukten
Bitte beachten Sie, dass alle Artikel und Produktinformationen, die auf BenchChem präsentiert werden, ausschließlich zu Informationszwecken bestimmt sind. Die auf BenchChem zum Kauf angebotenen Produkte sind speziell für In-vitro-Studien konzipiert, die außerhalb lebender Organismen durchgeführt werden. In-vitro-Studien, abgeleitet von dem lateinischen Begriff "in Glas", beinhalten Experimente, die in kontrollierten Laborumgebungen unter Verwendung von Zellen oder Geweben durchgeführt werden. Es ist wichtig zu beachten, dass diese Produkte nicht als Arzneimittel oder Medikamente eingestuft sind und keine Zulassung der FDA für die Vorbeugung, Behandlung oder Heilung von medizinischen Zuständen, Beschwerden oder Krankheiten erhalten haben. Wir müssen betonen, dass jede Form der körperlichen Einführung dieser Produkte in Menschen oder Tiere gesetzlich strikt untersagt ist. Es ist unerlässlich, sich an diese Richtlinien zu halten, um die Einhaltung rechtlicher und ethischer Standards in Forschung und Experiment zu gewährleisten.