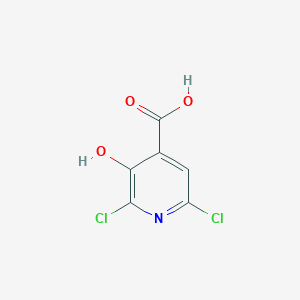
2,6-Dichloro-3-hydroxyisonicotinic acid
- Klicken Sie auf QUICK INQUIRY, um ein Angebot von unserem Expertenteam zu erhalten.
- Mit qualitativ hochwertigen Produkten zu einem WETTBEWERBSFÄHIGEN Preis können Sie sich mehr auf Ihre Forschung konzentrieren.
Übersicht
Beschreibung
2,6-Dichloro-3-hydroxyisonicotinic acid is a chemical compound with the CAS Number: 185422-96-2 and Linear Formula: C6H3Cl2NO3 . It has a molecular weight of 208 .
Molecular Structure Analysis
The IUPAC name for this compound is 2,6-dichloro-3-hydroxyisonicotinic acid . The InChI code is 1S/C6H3Cl2NO3/c7-3-1-2(6(11)12)4(10)5(8)9-3/h1,10H,(H,11,12) .Physical And Chemical Properties Analysis
This compound has a boiling point of 548.7°C at 760 mmHg . It is a solid at room temperature and should be stored in an inert atmosphere at 2-8°C .Wissenschaftliche Forschungsanwendungen
Plant Disease Resistance
2,6-Dichloro-3-hydroxyisonicotinic acid (INA) has been found to be effective in inducing plant resistance against bacteria and fungi . It has been used in field conditions to increase the resistance of pepper, pear, and rice to diseases caused by pathogens .
Systemic Acquired Resistance (SAR)
INA is known to induce Systemic Acquired Resistance (SAR) in plants . SAR is a natural plant defense mechanism that has been developed by plants through the evolutionary process. It has a broad spectrum action against pathogens .
Elicitor of Plant Immune System
INA is a synthetic elicitor that can stimulate the immune system of plants . The use of suitable substances (elicitors) stimulates the immune system of plants, which makes them resistant to infections even before the first symptoms appear .
Crop Protection
INA is very effective in protecting various crops against a wide range of pathogens . It provides an environmentally-friendly alternative to traditional pesticides .
Induction of Plant Defense Responses
INA is used to induce plant defense responses . It is used to activate plant defense responses and provide attractive alternatives to conventional biocidal agrochemicals .
Research in Plant Health Protection
INA plays a significant role in the field of plant health protection . Prophylaxis is much more effective and profitable than combating the consequences of pathogen infections .
Safety and Hazards
Wirkmechanismus
Target of Action
The primary target of 2,6-Dichloro-3-hydroxyisonicotinic acid (also known as INA) is the immune system of plants . It acts as an elicitor, a substance that stimulates the immune system of plants, making them resistant to infections even before the first symptoms appear .
Mode of Action
INA interacts with its targets by imitating the plant-pathogen interaction . This interaction triggers the plant’s natural defense mechanism, known as Systemic Acquired Resistance (SAR), which has a broad spectrum action against pathogens .
Biochemical Pathways
The biochemical pathway affected by INA is the SAR pathway . When a plant is attacked by a pathogen or exposed to INA, the SAR pathway is initiated . This pathway leads to the production of pathogenesis-related proteins that help the plant resist future pathogen attacks .
Pharmacokinetics
It is known that ina has high gastrointestinal absorption and is bbb permeant . Its lipophilicity (Log Po/w) is 1.18, indicating that it can easily cross cell membranes . The water solubility of INA is 0.204 mg/ml, suggesting that it can be distributed in the body fluids .
Result of Action
The result of INA’s action is increased resistance of plants to diseases caused by pathogens . For example, the application of INA in field conditions increased the resistance of pepper, pear, and rice to diseases caused by pathogens .
Eigenschaften
IUPAC Name |
2,6-dichloro-3-hydroxypyridine-4-carboxylic acid |
Source
|
---|---|---|
Source | PubChem | |
URL | https://pubchem.ncbi.nlm.nih.gov | |
Description | Data deposited in or computed by PubChem | |
InChI |
InChI=1S/C6H3Cl2NO3/c7-3-1-2(6(11)12)4(10)5(8)9-3/h1,10H,(H,11,12) |
Source
|
Source | PubChem | |
URL | https://pubchem.ncbi.nlm.nih.gov | |
Description | Data deposited in or computed by PubChem | |
InChI Key |
LOQNBXJKGZGLCJ-UHFFFAOYSA-N |
Source
|
Source | PubChem | |
URL | https://pubchem.ncbi.nlm.nih.gov | |
Description | Data deposited in or computed by PubChem | |
Canonical SMILES |
C1=C(C(=C(N=C1Cl)Cl)O)C(=O)O |
Source
|
Source | PubChem | |
URL | https://pubchem.ncbi.nlm.nih.gov | |
Description | Data deposited in or computed by PubChem | |
Molecular Formula |
C6H3Cl2NO3 |
Source
|
Source | PubChem | |
URL | https://pubchem.ncbi.nlm.nih.gov | |
Description | Data deposited in or computed by PubChem | |
DSSTOX Substance ID |
DTXSID60376273 |
Source
|
Record name | 2,6-Dichloro-3-hydroxyisonicotinic acid | |
Source | EPA DSSTox | |
URL | https://comptox.epa.gov/dashboard/DTXSID60376273 | |
Description | DSSTox provides a high quality public chemistry resource for supporting improved predictive toxicology. | |
Molecular Weight |
208.00 g/mol |
Source
|
Source | PubChem | |
URL | https://pubchem.ncbi.nlm.nih.gov | |
Description | Data deposited in or computed by PubChem | |
Product Name |
2,6-Dichloro-3-hydroxyisonicotinic acid | |
CAS RN |
185422-96-2 |
Source
|
Record name | 2,6-Dichloro-3-hydroxyisonicotinic acid | |
Source | EPA DSSTox | |
URL | https://comptox.epa.gov/dashboard/DTXSID60376273 | |
Description | DSSTox provides a high quality public chemistry resource for supporting improved predictive toxicology. | |
Haftungsausschluss und Informationen zu In-Vitro-Forschungsprodukten
Bitte beachten Sie, dass alle Artikel und Produktinformationen, die auf BenchChem präsentiert werden, ausschließlich zu Informationszwecken bestimmt sind. Die auf BenchChem zum Kauf angebotenen Produkte sind speziell für In-vitro-Studien konzipiert, die außerhalb lebender Organismen durchgeführt werden. In-vitro-Studien, abgeleitet von dem lateinischen Begriff "in Glas", beinhalten Experimente, die in kontrollierten Laborumgebungen unter Verwendung von Zellen oder Geweben durchgeführt werden. Es ist wichtig zu beachten, dass diese Produkte nicht als Arzneimittel oder Medikamente eingestuft sind und keine Zulassung der FDA für die Vorbeugung, Behandlung oder Heilung von medizinischen Zuständen, Beschwerden oder Krankheiten erhalten haben. Wir müssen betonen, dass jede Form der körperlichen Einführung dieser Produkte in Menschen oder Tiere gesetzlich strikt untersagt ist. Es ist unerlässlich, sich an diese Richtlinien zu halten, um die Einhaltung rechtlicher und ethischer Standards in Forschung und Experiment zu gewährleisten.