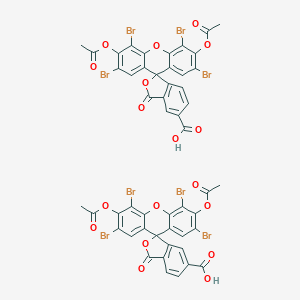
5(6)-Carboxyeosin Diacetat
Übersicht
Beschreibung
5(6)-Carboxyeosin-diacetate is a fluorescent dye commonly used in various scientific research applications. It is a derivative of eosin, a red dye that fluoresces under specific conditions. This compound is particularly valuable in biological and chemical research due to its ability to bind to certain molecules and emit fluorescence, making it useful for imaging and analytical techniques.
Wissenschaftliche Forschungsanwendungen
5(6)-Carboxyeosin-diacetate has a wide range of applications in scientific research:
Chemistry: It is used as a fluorescent probe to study chemical reactions and molecular interactions.
Biology: The compound is employed in cell imaging and fluorescence microscopy to visualize cellular structures and processes.
Medicine: It is used in diagnostic assays and as a marker in various medical tests.
Industry: The compound is used in quality control processes and as a tracer in environmental studies.
Wirkmechanismus
Target of Action
5(6)-Carboxyeosin diacetate, also known as 5(6)-Carboxyfluorescein diacetate (CFDA), is primarily used as a fluorescent tracer . It targets intracellular molecules by covalently binding to them . This compound is particularly useful in tracking lymphocyte migration and proliferation .
Mode of Action
The compound is a cell-permeant esterase substrate . It enters cells passively and is then hydrolyzed by intracellular esterases to produce the fluorescent product 5(6)-Carboxyfluorescein . This fluorescence can be used to track the enzymatic activity within cells and assess cell-membrane integrity .
Biochemical Pathways
It’s known that the compound’s fluorescence is activated by enzymatic activity, specifically by esterases . Therefore, it can be inferred that the compound may interact with pathways involving these enzymes.
Pharmacokinetics
A study on a similar compound, 5 (and 6)-carboxy-2′,7′-dichlorofluorescein, suggests that such compounds can passively diffuse into cells where they are hydrolyzed to their fluorescent forms . The fluorescent product is then effluxed via multidrug resistance-associated proteins into bile and sinusoidal blood .
Result of Action
The primary result of the action of 5(6)-Carboxyeosin diacetate is the production of fluorescence within cells . This fluorescence can be used to track cell activity and assess cell viability, making the compound a valuable tool in various biological and medical research applications .
Action Environment
The action of 5(6)-Carboxyeosin diacetate can be influenced by various environmental factors. For instance, the compound’s fluorescence is activated by enzymatic activity, which can vary depending on the cellular environment . Additionally, the compound’s ability to passively diffuse into cells suggests that its action may be influenced by factors affecting cell membrane permeability.
Biochemische Analyse
Biochemical Properties
5(6)-Carboxyeosin diacetate is known to interact with various enzymes, proteins, and other biomolecules within cells. One of the key interactions is with esterase enzymes, which hydrolyze the compound to produce a fluorescent product . This interaction is often used to assess enzymatic activity within cells .
Cellular Effects
The effects of 5(6)-Carboxyeosin diacetate on cells are primarily related to its role as a fluorescent tracer. The compound can be used to monitor various cellular processes, including xylem transport in plants . It is also used as a viability probe that assesses enzymatic activity and cell-membrane integrity .
Molecular Mechanism
The molecular mechanism of action of 5(6)-Carboxyeosin diacetate involves its hydrolysis by esterase enzymes within cells. This process produces a fluorescent product that can be detected and measured, providing insights into cellular processes and enzymatic activity .
Temporal Effects in Laboratory Settings
The effects of 5(6)-Carboxyeosin diacetate can change over time in laboratory settings. For example, in studies of xylem transport in plants, the compound was found to have a running speed of 0.3 cm/h in the isolated stem, which was consistent with the running speed of the material in the field .
Metabolic Pathways
The metabolic pathways involving 5(6)-Carboxyeosin diacetate primarily involve its hydrolysis by esterase enzymes within cells. This process produces a fluorescent product, which can then be detected and measured .
Transport and Distribution
5(6)-Carboxyeosin diacetate is known to passively diffuse into cells . Once inside the cell, it can be hydrolyzed by esterase enzymes to produce a fluorescent product. This product can then be transported between the xylem and parenchyma cells, indicating that material transport in the xylem could be through the symplastic pathway .
Subcellular Localization
The subcellular localization of 5(6)-Carboxyeosin diacetate and its fluorescent product can vary depending on the cell type and the specific experimental conditions. In some studies, the compound and its product have been observed in the cytoplasm, nucleus, and plastids of cells .
Vorbereitungsmethoden
Synthetic Routes and Reaction Conditions: The synthesis of 5(6)-Carboxyeosin-diacetate typically involves the reaction of eosin with acetic anhydride in the presence of a catalyst. The reaction conditions often include a controlled temperature and pH to ensure the proper formation of the diacetate derivative. The process may also involve purification steps such as recrystallization to obtain a high-purity product.
Industrial Production Methods: In an industrial setting, the production of 5(6)-Carboxyeosin-diacetate may involve large-scale reactors and automated systems to control the reaction parameters precisely. The use of high-throughput purification techniques, such as chromatography, ensures the consistent quality and purity of the compound.
Analyse Chemischer Reaktionen
Types of Reactions: 5(6)-Carboxyeosin-diacetate can undergo various chemical reactions, including:
Oxidation: The compound can be oxidized under specific conditions, leading to the formation of different oxidation products.
Reduction: Reduction reactions can convert the compound into its reduced forms, altering its fluorescence properties.
Substitution: The compound can participate in substitution reactions where one or more of its functional groups are replaced by other groups.
Common Reagents and Conditions:
Oxidation: Common oxidizing agents include hydrogen peroxide and potassium permanganate.
Reduction: Reducing agents such as sodium borohydride or lithium aluminum hydride are often used.
Substitution: Various reagents, including halogens and nucleophiles, can be used under controlled conditions.
Major Products: The major products formed from these reactions depend on the specific reagents and conditions used. For example, oxidation may yield different carboxylated derivatives, while reduction can produce deacetylated forms of the compound.
Vergleich Mit ähnlichen Verbindungen
Eosin Y: Another derivative of eosin, commonly used in histology.
Fluorescein: A widely used fluorescent dye with similar applications.
Rhodamine: Another fluorescent dye with distinct spectral properties.
Uniqueness: 5(6)-Carboxyeosin-diacetate is unique due to its specific fluorescence properties and its ability to bind to a wide range of molecules. This makes it particularly valuable in applications requiring high sensitivity and specificity.
Biologische Aktivität
5(6)-Carboxyeosin diacetate (CEDA) is a fluorescent dye widely used in biological research due to its membrane-permeant properties and ability to be hydrolyzed by intracellular esterases. This compound has garnered attention for its applications in cell viability assays, tracking cellular processes, and studying various biological mechanisms. This article reviews the biological activity of CEDA, focusing on its mechanisms, applications, and relevant research findings.
- Chemical Name : 5(6)-Carboxyeosin diacetate
- Molecular Formula : C₁₈H₁₄O₈
- Molecular Weight : 374.29 g/mol
- Solubility : Soluble in DMSO and other organic solvents
Upon entering cells, CEDA is hydrolyzed by intracellular esterases to produce carboxyfluorescein, a highly fluorescent compound. This reaction allows for the visualization of live cells and their metabolic activity. The fluorescence emitted can be detected using flow cytometry or fluorescence microscopy, making it a valuable tool for assessing cell viability and proliferation.
Applications in Biological Research
- Cell Viability Assays : CEDA is commonly used to assess cell viability in various experimental setups. It provides a straightforward method for determining the health of cells after treatment with drugs or other agents.
- Tracking Cell Proliferation : The dye allows researchers to track cell division over time. By labeling cells at the start of an experiment, changes in fluorescence intensity can be monitored to assess proliferation rates.
- Chemotaxis Studies : Research has demonstrated that CEDA can inhibit chemotactic behavior in sperm cells by affecting calcium ion efflux through plasma membrane calcium ATPase (PMCA) mechanisms. Specifically, studies have shown that pre-treatment with CEDA significantly reduces sperm chemotaxis towards attractants .
Case Study 1: Viability Assessment in Lacticaseibacillus Casei Zhang
A study developed a rapid flow cytometry method using CEDA to detect the viability of Lacticaseibacillus casei Zhang. The results indicated that varying concentrations of CEDA and different staining times significantly affected the viable count of the bacteria:
Experiment | CFDA Amount (μL) | Dyeing Time t1 (s) | Viable Count (AFU/μL) |
---|---|---|---|
1 | 5 | 5 | 1661 |
2 | 5 | 10 | 1762 |
3 | 10 | 15 | 1897 |
... | ... | ... | ... |
This illustrates the versatility of CEDA in microbial studies, providing insights into bacterial viability under various conditions .
Case Study 2: Chemotaxis Inhibition in Sperm Cells
In another significant study, CEDA was used to investigate its effects on sperm chemotaxis. The findings revealed that treatment with CEDA inhibited PMCA activity, leading to disrupted calcium homeostasis and impaired chemotactic behavior:
- Control Group LECI : Baseline chemotaxis index.
- CEDA Treatment Group LECI : Reduced to one-third at lower concentrations and nearly zero at higher concentrations.
This highlights the role of calcium signaling in sperm motility and how CEDA can serve as a tool for studying these processes .
Eigenschaften
IUPAC Name |
3',6'-diacetyloxy-2',4',5',7'-tetrabromo-1-oxospiro[2-benzofuran-3,9'-xanthene]-5-carboxylic acid;3',6'-diacetyloxy-2',4',5',7'-tetrabromo-3-oxospiro[2-benzofuran-1,9'-xanthene]-5-carboxylic acid | |
---|---|---|
Source | PubChem | |
URL | https://pubchem.ncbi.nlm.nih.gov | |
Description | Data deposited in or computed by PubChem | |
InChI |
InChI=1S/2C25H12Br4O9/c1-8(30)35-21-15(26)6-13-19(17(21)28)37-20-14(7-16(27)22(18(20)29)36-9(2)31)25(13)12-4-3-10(23(32)33)5-11(12)24(34)38-25;1-8(30)35-21-15(26)6-13-19(17(21)28)37-20-14(7-16(27)22(18(20)29)36-9(2)31)25(13)12-5-10(23(32)33)3-4-11(12)24(34)38-25/h2*3-7H,1-2H3,(H,32,33) | |
Source | PubChem | |
URL | https://pubchem.ncbi.nlm.nih.gov | |
Description | Data deposited in or computed by PubChem | |
InChI Key |
VUSZJWOKNYZNPK-UHFFFAOYSA-N | |
Source | PubChem | |
URL | https://pubchem.ncbi.nlm.nih.gov | |
Description | Data deposited in or computed by PubChem | |
Canonical SMILES |
CC(=O)OC1=C(C=C2C(=C1Br)OC3=C(C(=C(C=C3C24C5=C(C=C(C=C5)C(=O)O)C(=O)O4)Br)OC(=O)C)Br)Br.CC(=O)OC1=C(C=C2C(=C1Br)OC3=C(C(=C(C=C3C24C5=C(C=CC(=C5)C(=O)O)C(=O)O4)Br)OC(=O)C)Br)Br | |
Source | PubChem | |
URL | https://pubchem.ncbi.nlm.nih.gov | |
Description | Data deposited in or computed by PubChem | |
Molecular Formula |
C50H24Br8O18 | |
Source | PubChem | |
URL | https://pubchem.ncbi.nlm.nih.gov | |
Description | Data deposited in or computed by PubChem | |
DSSTOX Substance ID |
DTXSID70583451 | |
Record name | 3',6'-Bis(acetyloxy)-2',4',5',7'-tetrabromo-3-oxo-3H-spiro[2-benzofuran-1,9'-xanthene]-5-carboxylic acid--3',6'-bis(acetyloxy)-2',4',5',7'-tetrabromo-3-oxo-3H-spiro[2-benzofuran-1,9'-xanthene]-6-carboxylic acid (1/1) | |
Source | EPA DSSTox | |
URL | https://comptox.epa.gov/dashboard/DTXSID70583451 | |
Description | DSSTox provides a high quality public chemistry resource for supporting improved predictive toxicology. | |
Molecular Weight |
1551.9 g/mol | |
Source | PubChem | |
URL | https://pubchem.ncbi.nlm.nih.gov | |
Description | Data deposited in or computed by PubChem | |
CAS No. |
161338-87-0 | |
Record name | 3',6'-Bis(acetyloxy)-2',4',5',7'-tetrabromo-3-oxo-3H-spiro[2-benzofuran-1,9'-xanthene]-5-carboxylic acid--3',6'-bis(acetyloxy)-2',4',5',7'-tetrabromo-3-oxo-3H-spiro[2-benzofuran-1,9'-xanthene]-6-carboxylic acid (1/1) | |
Source | EPA DSSTox | |
URL | https://comptox.epa.gov/dashboard/DTXSID70583451 | |
Description | DSSTox provides a high quality public chemistry resource for supporting improved predictive toxicology. | |
Retrosynthesis Analysis
AI-Powered Synthesis Planning: Our tool employs the Template_relevance Pistachio, Template_relevance Bkms_metabolic, Template_relevance Pistachio_ringbreaker, Template_relevance Reaxys, Template_relevance Reaxys_biocatalysis model, leveraging a vast database of chemical reactions to predict feasible synthetic routes.
One-Step Synthesis Focus: Specifically designed for one-step synthesis, it provides concise and direct routes for your target compounds, streamlining the synthesis process.
Accurate Predictions: Utilizing the extensive PISTACHIO, BKMS_METABOLIC, PISTACHIO_RINGBREAKER, REAXYS, REAXYS_BIOCATALYSIS database, our tool offers high-accuracy predictions, reflecting the latest in chemical research and data.
Strategy Settings
Precursor scoring | Relevance Heuristic |
---|---|
Min. plausibility | 0.01 |
Model | Template_relevance |
Template Set | Pistachio/Bkms_metabolic/Pistachio_ringbreaker/Reaxys/Reaxys_biocatalysis |
Top-N result to add to graph | 6 |
Feasible Synthetic Routes
Q1: How does 5(6)-Carboxyeosin-diacetate enable the measurement of intracellular pH?
A: 5(6)-Carboxyeosin-diacetate is a pH-dependent fluorescent dye. This means its fluorescence intensity changes depending on the pH of its surrounding environment. When used in conjunction with techniques like fiber-optic nanoprobes, researchers can measure these fluorescence changes to determine intracellular pH []. Essentially, the dye acts as a reporter molecule, translating pH changes into detectable fluorescent signals.
Q2: Can you provide a specific example of how 5(6)-Carboxyeosin-diacetate was used in research to study intracellular pH dynamics?
A: In a study focusing on CA46 cells, researchers used 5(6)-Carboxyeosin-diacetate to monitor changes in intracellular pH caused by the addition and removal of ammonium ions (NH4+) []. By observing the fluctuations in fluorescence intensity, the researchers could track how the cells regulated their internal pH in response to this external stimulus. This provided valuable insights into the pH regulatory mechanisms of CA46 cells.
Q3: Beyond CA46 cells, are there other biological systems where 5(6)-Carboxyeosin-diacetate has been used to study pH changes?
A: Yes, 5(6)-Carboxyeosin-diacetate has proven valuable in studying neuronal activity. Research on Drosophila larvae neurons used this dye to investigate the impact of intracellular pH shifts on vesicle fusion, a critical process in synaptic transmission []. This study highlighted the role of pH as a potential signaling molecule in neuronal communication.
Haftungsausschluss und Informationen zu In-Vitro-Forschungsprodukten
Bitte beachten Sie, dass alle Artikel und Produktinformationen, die auf BenchChem präsentiert werden, ausschließlich zu Informationszwecken bestimmt sind. Die auf BenchChem zum Kauf angebotenen Produkte sind speziell für In-vitro-Studien konzipiert, die außerhalb lebender Organismen durchgeführt werden. In-vitro-Studien, abgeleitet von dem lateinischen Begriff "in Glas", beinhalten Experimente, die in kontrollierten Laborumgebungen unter Verwendung von Zellen oder Geweben durchgeführt werden. Es ist wichtig zu beachten, dass diese Produkte nicht als Arzneimittel oder Medikamente eingestuft sind und keine Zulassung der FDA für die Vorbeugung, Behandlung oder Heilung von medizinischen Zuständen, Beschwerden oder Krankheiten erhalten haben. Wir müssen betonen, dass jede Form der körperlichen Einführung dieser Produkte in Menschen oder Tiere gesetzlich strikt untersagt ist. Es ist unerlässlich, sich an diese Richtlinien zu halten, um die Einhaltung rechtlicher und ethischer Standards in Forschung und Experiment zu gewährleisten.