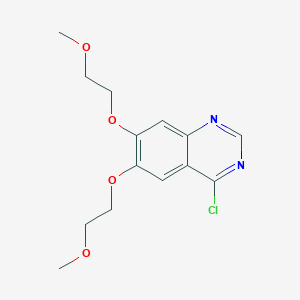
4-Chloro-6,7-bis(2-methoxyethoxy)quinazoline
Übersicht
Beschreibung
4-Chloro-6,7-bis(2-methoxyethoxy)quinazoline is a synthetic compound that has been shown to inhibit the growth of cancer cells . It is a building block and synthetic intermediate . It has been used as a precursor in the synthesis of receptor tyrosine kinase (RTK) inhibitors, dual RTK and histone deacetylase (HDAC) inhibitors, and anticancer compounds .
Synthesis Analysis
4-Chloro-6,7-bis(2-methoxyethoxy)quinazoline is a synthetic intermediate. It has been used as a precursor in the synthesis of receptor tyrosine kinase (RTK) inhibitors, dual RTK and histone deacetylase (HDAC) inhibitors, and anticancer compounds .Molecular Structure Analysis
The molecular formula of 4-Chloro-6,7-bis(2-methoxyethoxy)quinazoline is C14H17ClN2O4 . The molecular weight is 312.75 .Chemical Reactions Analysis
4-Chloro-6,7-bis(2-methoxyethoxy)quinazoline is a synthetic compound that reacts with hydrochloric acid in the stomach to form a chloride salt, which can be absorbed into the bloodstream .Wissenschaftliche Forschungsanwendungen
Synthesis of Receptor Tyrosine Kinase (RTK) Inhibitors
“4-Chloro-6,7-bis(2-methoxyethoxy)quinazoline” has been used as a precursor in the synthesis of receptor tyrosine kinase (RTK) inhibitors . RTK inhibitors are a type of targeted therapy that specifically target and inhibit tyrosine kinases, enzymes responsible for the activation of many proteins by signal transduction cascades. These inhibitors have shown promise in the treatment of cancer .
Synthesis of Dual RTK and Histone Deacetylase (HDAC) Inhibitors
This compound has also been used in the synthesis of dual RTK and HDAC inhibitors . HDAC inhibitors are a class of drugs that inhibit histone deacetylases, and are being studied as a treatment for cancer. By combining RTK and HDAC inhibition, researchers aim to develop more effective cancer treatments .
Synthesis of Anticancer Compounds
“4-Chloro-6,7-bis(2-methoxyethoxy)quinazoline” is a synthetic intermediate in the synthesis of various anticancer compounds . These compounds aim to inhibit the growth of cancer cells and have shown promise in preclinical and clinical trials .
Synthesis of EGFR Inhibitors
This compound is also a synthetic intermediate in the synthesis of EGFR inhibitors, including erlotinib . EGFR inhibitors are drugs that bind to the epidermal growth factor receptor (EGFR) and thereby block the activation of the receptor and the downstream signal transduction pathways, which leads to inhibiting the growth of cancer cells .
Aurora 2 Kinase Inhibition
“4-Chloro-6,7-bis(2-methoxyethoxy)quinazoline” is known to be an aurora 2 kinase inhibitor . Aurora kinases are essential for cell division, and their inhibition is being explored as a potential strategy for cancer treatment .
PDGF Inhibition
This compound is also a PDGF (Platelet-Derived Growth Factor) inhibitor . PDGFs are proteins involved in cell growth, division, and angiogenesis. Inhibiting PDGF can be useful in the treatment of cancer, as it can prevent the growth of new blood vessels that tumors need to grow .
Wirkmechanismus
Target of Action
The primary targets of 4-Chloro-6,7-bis(2-methoxyethoxy)quinazoline are Aurora Kinase 2 (ARK-2) and Platelet-Derived Growth Factor (PDGF) . These targets play crucial roles in cell division and growth, respectively. ARK-2 is involved in the regulation of the cell cycle, particularly during mitosis, while PDGF is a potent mitogen for cells of mesenchymal origin.
Mode of Action
4-Chloro-6,7-bis(2-methoxyethoxy)quinazoline acts as an inhibitor of its primary targets. It binds to the active sites of ARK-2 and PDGF, preventing these proteins from performing their normal functions . This interaction leads to changes in cellular processes, particularly those related to cell division and growth.
Biochemical Pathways
The inhibition of ARK-2 and PDGF affects several biochemical pathways. The most significant of these is the cell cycle pathway , where ARK-2 normally promotes progression from G2 to M phase. Its inhibition can lead to cell cycle arrest . PDGF inhibition, on the other hand, can lead to reduced cell proliferation and angiogenesis .
Result of Action
The molecular and cellular effects of 4-Chloro-6,7-bis(2-methoxyethoxy)quinazoline’s action include cell cycle arrest and reduced cell proliferation . These effects can lead to the death of rapidly dividing cells, such as cancer cells, making this compound potentially useful in cancer treatment.
Safety and Hazards
Eigenschaften
IUPAC Name |
4-chloro-6,7-bis(2-methoxyethoxy)quinazoline | |
---|---|---|
Source | PubChem | |
URL | https://pubchem.ncbi.nlm.nih.gov | |
Description | Data deposited in or computed by PubChem | |
InChI |
InChI=1S/C14H17ClN2O4/c1-18-3-5-20-12-7-10-11(16-9-17-14(10)15)8-13(12)21-6-4-19-2/h7-9H,3-6H2,1-2H3 | |
Source | PubChem | |
URL | https://pubchem.ncbi.nlm.nih.gov | |
Description | Data deposited in or computed by PubChem | |
InChI Key |
ZPJLDMNVDPGZIU-UHFFFAOYSA-N | |
Source | PubChem | |
URL | https://pubchem.ncbi.nlm.nih.gov | |
Description | Data deposited in or computed by PubChem | |
Canonical SMILES |
COCCOC1=C(C=C2C(=C1)C(=NC=N2)Cl)OCCOC | |
Source | PubChem | |
URL | https://pubchem.ncbi.nlm.nih.gov | |
Description | Data deposited in or computed by PubChem | |
Molecular Formula |
C14H17ClN2O4 | |
Source | PubChem | |
URL | https://pubchem.ncbi.nlm.nih.gov | |
Description | Data deposited in or computed by PubChem | |
DSSTOX Substance ID |
DTXSID70461407 | |
Record name | 4-Chloro-6,7-bis(2-methoxyethoxy)quinazoline | |
Source | EPA DSSTox | |
URL | https://comptox.epa.gov/dashboard/DTXSID70461407 | |
Description | DSSTox provides a high quality public chemistry resource for supporting improved predictive toxicology. | |
Molecular Weight |
312.75 g/mol | |
Source | PubChem | |
URL | https://pubchem.ncbi.nlm.nih.gov | |
Description | Data deposited in or computed by PubChem | |
Product Name |
4-Chloro-6,7-bis(2-methoxyethoxy)quinazoline | |
CAS RN |
183322-18-1 | |
Record name | 4-Chloro-6,7-bis(2-methoxyethoxy)quinazoline | |
Source | CAS Common Chemistry | |
URL | https://commonchemistry.cas.org/detail?cas_rn=183322-18-1 | |
Description | CAS Common Chemistry is an open community resource for accessing chemical information. Nearly 500,000 chemical substances from CAS REGISTRY cover areas of community interest, including common and frequently regulated chemicals, and those relevant to high school and undergraduate chemistry classes. This chemical information, curated by our expert scientists, is provided in alignment with our mission as a division of the American Chemical Society. | |
Explanation | The data from CAS Common Chemistry is provided under a CC-BY-NC 4.0 license, unless otherwise stated. | |
Record name | 4-Chloro-6,7-bis(2-methoxyethoxy)quinazoline | |
Source | ChemIDplus | |
URL | https://pubchem.ncbi.nlm.nih.gov/substance/?source=chemidplus&sourceid=0183322181 | |
Description | ChemIDplus is a free, web search system that provides access to the structure and nomenclature authority files used for the identification of chemical substances cited in National Library of Medicine (NLM) databases, including the TOXNET system. | |
Record name | 4-Chloro-6,7-bis(2-methoxyethoxy)quinazoline | |
Source | EPA DSSTox | |
URL | https://comptox.epa.gov/dashboard/DTXSID70461407 | |
Description | DSSTox provides a high quality public chemistry resource for supporting improved predictive toxicology. | |
Record name | 4-CHLORO-6,7-BIS(2-METHOXYETHOXY)QUINAZOLINE | |
Source | FDA Global Substance Registration System (GSRS) | |
URL | https://gsrs.ncats.nih.gov/ginas/app/beta/substances/K44UPE46KJ | |
Description | The FDA Global Substance Registration System (GSRS) enables the efficient and accurate exchange of information on what substances are in regulated products. Instead of relying on names, which vary across regulatory domains, countries, and regions, the GSRS knowledge base makes it possible for substances to be defined by standardized, scientific descriptions. | |
Explanation | Unless otherwise noted, the contents of the FDA website (www.fda.gov), both text and graphics, are not copyrighted. They are in the public domain and may be republished, reprinted and otherwise used freely by anyone without the need to obtain permission from FDA. Credit to the U.S. Food and Drug Administration as the source is appreciated but not required. | |
Synthesis routes and methods I
Procedure details
Synthesis routes and methods II
Procedure details
Synthesis routes and methods III
Procedure details
Retrosynthesis Analysis
AI-Powered Synthesis Planning: Our tool employs the Template_relevance Pistachio, Template_relevance Bkms_metabolic, Template_relevance Pistachio_ringbreaker, Template_relevance Reaxys, Template_relevance Reaxys_biocatalysis model, leveraging a vast database of chemical reactions to predict feasible synthetic routes.
One-Step Synthesis Focus: Specifically designed for one-step synthesis, it provides concise and direct routes for your target compounds, streamlining the synthesis process.
Accurate Predictions: Utilizing the extensive PISTACHIO, BKMS_METABOLIC, PISTACHIO_RINGBREAKER, REAXYS, REAXYS_BIOCATALYSIS database, our tool offers high-accuracy predictions, reflecting the latest in chemical research and data.
Strategy Settings
Precursor scoring | Relevance Heuristic |
---|---|
Min. plausibility | 0.01 |
Model | Template_relevance |
Template Set | Pistachio/Bkms_metabolic/Pistachio_ringbreaker/Reaxys/Reaxys_biocatalysis |
Top-N result to add to graph | 6 |
Feasible Synthetic Routes
Q & A
Q1: What is the role of 4-Chloro-6,7-bis(2-methoxyethoxy)quinazoline in the synthesis of Erlotinib Hydrochloride?
A1: 4-Chloro-6,7-bis(2-methoxyethoxy)quinazoline serves as a key intermediate in the synthesis of Erlotinib Hydrochloride. [, ] Both research papers describe a synthetic route where this compound reacts with m-ethynylaniline or m-aminostyrene, leading to the formation of the final drug molecule or a related impurity, respectively. You can find more details about the reaction conditions and yields in the papers:
Haftungsausschluss und Informationen zu In-Vitro-Forschungsprodukten
Bitte beachten Sie, dass alle Artikel und Produktinformationen, die auf BenchChem präsentiert werden, ausschließlich zu Informationszwecken bestimmt sind. Die auf BenchChem zum Kauf angebotenen Produkte sind speziell für In-vitro-Studien konzipiert, die außerhalb lebender Organismen durchgeführt werden. In-vitro-Studien, abgeleitet von dem lateinischen Begriff "in Glas", beinhalten Experimente, die in kontrollierten Laborumgebungen unter Verwendung von Zellen oder Geweben durchgeführt werden. Es ist wichtig zu beachten, dass diese Produkte nicht als Arzneimittel oder Medikamente eingestuft sind und keine Zulassung der FDA für die Vorbeugung, Behandlung oder Heilung von medizinischen Zuständen, Beschwerden oder Krankheiten erhalten haben. Wir müssen betonen, dass jede Form der körperlichen Einführung dieser Produkte in Menschen oder Tiere gesetzlich strikt untersagt ist. Es ist unerlässlich, sich an diese Richtlinien zu halten, um die Einhaltung rechtlicher und ethischer Standards in Forschung und Experiment zu gewährleisten.