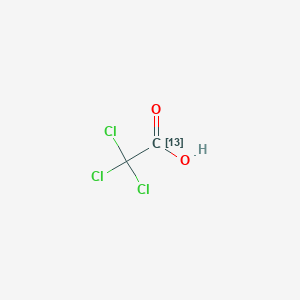
Trichloroessigsäure-1-13C
Übersicht
Beschreibung
Trichloroacetic acid-1-13C is a labeled compound where one of the carbon atoms in trichloroacetic acid is replaced with the carbon-13 isotope. This compound is used in various scientific research applications due to its unique properties. Trichloroacetic acid itself is a strong acid and a derivative of acetic acid, where all three hydrogen atoms of the methyl group are replaced by chlorine atoms .
Wissenschaftliche Forschungsanwendungen
Trichloroacetic acid-1-13C is widely used in scientific research due to its isotopic labeling, which allows for detailed studies in various fields:
Chemistry: Used as a catalyst in organic synthesis and for studying reaction mechanisms.
Biology: Employed in protein precipitation and DNA/RNA extraction.
Medicine: Utilized in clinical chemistry for protein precipitation and as a cauterant in cosmetic treatments.
Industry: Applied in the production of herbicides and other chemical products
Wirkmechanismus
Target of Action
Trichloroacetic acid-1-13C, an analogue of acetic acid, is a strong acid where the three hydrogen atoms of the methyl group have all been replaced by chlorine atoms . It primarily targets macromolecules including proteins, DNA, and RNA . It is used as a precipitant in clinical chemistry and biochemistry .
Mode of Action
It is known that it interacts with its targets (proteins, dna, and rna) and causes precipitation . This interaction and the resulting changes are crucial for its use in biochemistry and clinical chemistry.
Result of Action
The primary result of Trichloroacetic acid-1-13C’s action is the precipitation of macromolecules such as proteins, DNA, and RNA . This property is utilized in various biochemical and clinical chemistry applications.
Vorbereitungsmethoden
Synthetic Routes and Reaction Conditions
Trichloroacetic acid-1-13C can be synthesized by the chlorination of acetic acid-1-13C in the presence of a suitable catalyst such as red phosphorus. The reaction involves the substitution of hydrogen atoms with chlorine atoms: [ \text{CH}_3\text{COOH-1-13C} + 3\text{Cl}_2 \rightarrow \text{CCl}_3\text{COOH-1-13C} + 3\text{HCl} ] Another method involves the oxidation of trichloroacetaldehyde-1-13C .
Industrial Production Methods
Industrial production of trichloroacetic acid typically involves the chlorination of acetic acid or its derivatives at elevated temperatures (140–160 °C) with catalysts such as calcium hypochlorite. The crude product is then purified through melt crystallization and further enhanced via centrifugation or additional recrystallization steps .
Analyse Chemischer Reaktionen
Types of Reactions
Trichloroacetic acid-1-13C undergoes various chemical reactions, including:
Oxidation: It can be oxidized to produce carbon dioxide and chloroform.
Reduction: It can be reduced to form dichloroacetic acid.
Substitution: It can undergo nucleophilic substitution reactions where chlorine atoms are replaced by other nucleophiles.
Common Reagents and Conditions
Common reagents used in these reactions include chlorine gas for chlorination, oxygen for oxidation, and various nucleophiles for substitution reactions. The conditions typically involve elevated temperatures and the presence of catalysts .
Major Products Formed
The major products formed from these reactions include chloroform, dichloroacetic acid, and various substituted trichloroacetic acid derivatives .
Vergleich Mit ähnlichen Verbindungen
Similar Compounds
Chloroacetic acid: Contains one chlorine atom.
Dichloroacetic acid: Contains two chlorine atoms.
Trifluoroacetic acid: Contains three fluorine atoms instead of chlorine.
Uniqueness
Trichloroacetic acid-1-13C is unique due to its isotopic labeling, which allows for precise tracking and analysis in scientific studies. Its strong acidic nature and ability to precipitate macromolecules make it particularly valuable in biochemical and clinical applications .
Biologische Aktivität
2,2,2-Trichloroacetic acid (TCA) is a halogenated acetic acid that has garnered attention in various fields, particularly in biochemistry and dermatology. Its biological activity is primarily associated with its ability to precipitate proteins, its use in chemical peels, and its potential carcinogenic effects. This article delves into the mechanisms of TCA's biological activity, supported by case studies and research findings.
Mechanism of Protein Precipitation
TCA is widely used for protein precipitation in proteomics. It induces protein aggregation by disrupting hydrophobic interactions and salt bridges within proteins. The mechanism involves the formation of a "molten globule-like" state, where proteins partially unfold but do not completely denature. This state allows for effective precipitation at specific concentrations of TCA.
Key Findings on Protein Precipitation
- U-Shaped Precipitation Curves : Research indicates that TCA-induced protein precipitation curves are U-shaped, suggesting that both low and high concentrations of TCA are less effective than intermediate concentrations .
- Dependence on Protein Conformation : TCA is notably less effective at precipitating unfolded proteins. Studies using acidic fibroblast growth factor (aFGF) demonstrated that TCA preferentially precipitates proteins in their native or partially structured states .
- Concentration Effects : The optimal concentration for protein precipitation appears to be around 5% (w/v), where a stable intermediate accumulates .
Clinical Applications
TCA is commonly utilized in dermatological procedures for chemical peels due to its ability to induce controlled skin damage, promoting regeneration and improving skin texture.
Case Study: Inadvertent Use Leading to Adverse Effects
A significant clinical case involved a 31-year-old woman who experienced a severe reaction after TCA was mistakenly applied instead of acetic acid during a routine examination for a perineal lesion. The application led to immediate whitening of the area, indicating chemical burns. The patient was treated promptly with sodium bicarbonate to neutralize the acid, but she reported ongoing discomfort and discoloration one year later . This case underscores the importance of careful handling and application of TCA in clinical settings.
Toxicological Profile and Carcinogenicity
The safety profile of TCA has been evaluated through various studies, particularly focusing on its potential carcinogenic effects.
Research Findings on Carcinogenicity
- Animal Studies : Research conducted on B6C3F1 mice showed a statistically significant increase in hepatocellular adenomas and carcinomas following prolonged exposure to TCA in drinking water. In one study, male mice exposed to 5 g/L of TCA developed tumors at rates significantly higher than controls .
- Histopathological Evidence : Histological examinations revealed clear evidence of liver tumors in treated mice, reinforcing the notion that TCA possesses carcinogenic properties under certain conditions .
Summary Table of Biological Activities and Effects
Eigenschaften
IUPAC Name |
2,2,2-trichloroacetic acid | |
---|---|---|
Source | PubChem | |
URL | https://pubchem.ncbi.nlm.nih.gov | |
Description | Data deposited in or computed by PubChem | |
InChI |
InChI=1S/C2HCl3O2/c3-2(4,5)1(6)7/h(H,6,7)/i1+1 | |
Source | PubChem | |
URL | https://pubchem.ncbi.nlm.nih.gov | |
Description | Data deposited in or computed by PubChem | |
InChI Key |
YNJBWRMUSHSURL-OUBTZVSYSA-N | |
Source | PubChem | |
URL | https://pubchem.ncbi.nlm.nih.gov | |
Description | Data deposited in or computed by PubChem | |
Canonical SMILES |
C(=O)(C(Cl)(Cl)Cl)O | |
Source | PubChem | |
URL | https://pubchem.ncbi.nlm.nih.gov | |
Description | Data deposited in or computed by PubChem | |
Isomeric SMILES |
[13C](=O)(C(Cl)(Cl)Cl)O | |
Source | PubChem | |
URL | https://pubchem.ncbi.nlm.nih.gov | |
Description | Data deposited in or computed by PubChem | |
Molecular Formula |
C2HCl3O2 | |
Source | PubChem | |
URL | https://pubchem.ncbi.nlm.nih.gov | |
Description | Data deposited in or computed by PubChem | |
DSSTOX Substance ID |
DTXSID90565653 | |
Record name | Trichloro(1-~13~C)acetic acid | |
Source | EPA DSSTox | |
URL | https://comptox.epa.gov/dashboard/DTXSID90565653 | |
Description | DSSTox provides a high quality public chemistry resource for supporting improved predictive toxicology. | |
Molecular Weight |
164.38 g/mol | |
Source | PubChem | |
URL | https://pubchem.ncbi.nlm.nih.gov | |
Description | Data deposited in or computed by PubChem | |
CAS No. |
173470-69-4 | |
Record name | Trichloro(1-~13~C)acetic acid | |
Source | EPA DSSTox | |
URL | https://comptox.epa.gov/dashboard/DTXSID90565653 | |
Description | DSSTox provides a high quality public chemistry resource for supporting improved predictive toxicology. | |
Record name | 173470-69-4 | |
Source | European Chemicals Agency (ECHA) | |
URL | https://echa.europa.eu/information-on-chemicals | |
Description | The European Chemicals Agency (ECHA) is an agency of the European Union which is the driving force among regulatory authorities in implementing the EU's groundbreaking chemicals legislation for the benefit of human health and the environment as well as for innovation and competitiveness. | |
Explanation | Use of the information, documents and data from the ECHA website is subject to the terms and conditions of this Legal Notice, and subject to other binding limitations provided for under applicable law, the information, documents and data made available on the ECHA website may be reproduced, distributed and/or used, totally or in part, for non-commercial purposes provided that ECHA is acknowledged as the source: "Source: European Chemicals Agency, http://echa.europa.eu/". Such acknowledgement must be included in each copy of the material. ECHA permits and encourages organisations and individuals to create links to the ECHA website under the following cumulative conditions: Links can only be made to webpages that provide a link to the Legal Notice page. | |
Synthesis routes and methods I
Procedure details
Synthesis routes and methods II
Procedure details
Synthesis routes and methods III
Procedure details
Synthesis routes and methods IV
Procedure details
Retrosynthesis Analysis
AI-Powered Synthesis Planning: Our tool employs the Template_relevance Pistachio, Template_relevance Bkms_metabolic, Template_relevance Pistachio_ringbreaker, Template_relevance Reaxys, Template_relevance Reaxys_biocatalysis model, leveraging a vast database of chemical reactions to predict feasible synthetic routes.
One-Step Synthesis Focus: Specifically designed for one-step synthesis, it provides concise and direct routes for your target compounds, streamlining the synthesis process.
Accurate Predictions: Utilizing the extensive PISTACHIO, BKMS_METABOLIC, PISTACHIO_RINGBREAKER, REAXYS, REAXYS_BIOCATALYSIS database, our tool offers high-accuracy predictions, reflecting the latest in chemical research and data.
Strategy Settings
Precursor scoring | Relevance Heuristic |
---|---|
Min. plausibility | 0.01 |
Model | Template_relevance |
Template Set | Pistachio/Bkms_metabolic/Pistachio_ringbreaker/Reaxys/Reaxys_biocatalysis |
Top-N result to add to graph | 6 |
Feasible Synthetic Routes
Haftungsausschluss und Informationen zu In-Vitro-Forschungsprodukten
Bitte beachten Sie, dass alle Artikel und Produktinformationen, die auf BenchChem präsentiert werden, ausschließlich zu Informationszwecken bestimmt sind. Die auf BenchChem zum Kauf angebotenen Produkte sind speziell für In-vitro-Studien konzipiert, die außerhalb lebender Organismen durchgeführt werden. In-vitro-Studien, abgeleitet von dem lateinischen Begriff "in Glas", beinhalten Experimente, die in kontrollierten Laborumgebungen unter Verwendung von Zellen oder Geweben durchgeführt werden. Es ist wichtig zu beachten, dass diese Produkte nicht als Arzneimittel oder Medikamente eingestuft sind und keine Zulassung der FDA für die Vorbeugung, Behandlung oder Heilung von medizinischen Zuständen, Beschwerden oder Krankheiten erhalten haben. Wir müssen betonen, dass jede Form der körperlichen Einführung dieser Produkte in Menschen oder Tiere gesetzlich strikt untersagt ist. Es ist unerlässlich, sich an diese Richtlinien zu halten, um die Einhaltung rechtlicher und ethischer Standards in Forschung und Experiment zu gewährleisten.