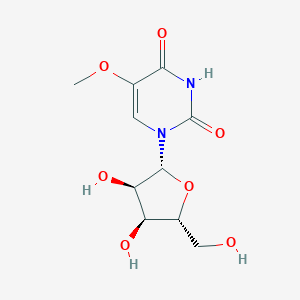
5-Methoxyuridine
Übersicht
Beschreibung
5-Methoxyuridine is a derivative of uridine, bearing an additional methoxy substituent at position 5 on the uracil ring . It is a purine nucleoside analog .
Synthesis Analysis
The synthesis of 5-Methoxyuridine has been mentioned in a few studies. One study revealed that uridine depletion and 5-methoxyuridine modification is critical for the stable expression of Adenine Base Editor (ABE) mRNA . Another study demonstrated that 5-methoxyuridine (5-moU) outperformed other modifications up to 4-fold increased transgene expression .
Molecular Structure Analysis
The three-dimensional structure of 5-methoxyuridine (mo5U) was determined with much higher precision than in a previous study . The introduction of m1Ψ in mRNA leads to errors during the translation of the IVT luciferase reporter, specifically causing a shift in the reading frame (+1 frameshift), which resulted in the production of shortened and altered proteins .
Chemical Reactions Analysis
5-Methoxyuridine is a purine nucleoside analog and has broad antitumor activity targeting indolent lymphoid malignancies . The anticancer mechanisms in this process rely on inhibition of DNA synthesis, induction of apoptosis, etc . Moreover, eGFP mRNAs with 5-methoxyuridine (5-moU) modifications ranked top three in cell lines tested .
Physical And Chemical Properties Analysis
5-Methoxyuridine is an analogue of the naturally occurring uridine triphosphate (UTP). Similar to N1-methylpseudouridine and pseudouridine modifications, 5-methoxyuridine is another common chemical modification used for in vitro mRNA synthesis in order to improve mRNA activity and reduce innate immune responses .
Wissenschaftliche Forschungsanwendungen
Therapeutic mRNA Modification
Chemically modified therapeutic mRNAs have gained momentum recently. In addition to commonly used modifications (e.g., pseudouridine), 5moU is considered a promising substitution of uridine in therapeutic mRNAs . Accurate identification of 5-Methoxyuridine (5moU) would be crucial for the study and quality control of relevant IVT mRNAs .
RNA Sequencing
By taking advantage of Oxford nanopore direct RNA sequencing, a machine-learning framework named NanoML-5moU has been designed specifically for the read-level detection and quantification of 5moU modification . This enables accurate read-level profiling of 5moU modification with nanopore direct RNA-sequencing .
Machine Learning Applications
The NanoML-5moU framework uses classical machine learning algorithms, including Support Vector Machine (SVM), Random Forest (RF), and XGBoost, for 5moU detection within NNUNN (N = A, C, T or G) 5-mers . This framework is publicly available on GitHub .
Gene Expression Regulation
RNA modification is a crucial post-transcriptional process that plays a pivotal role in fine-tuning gene expression, mRNA stability, splicing, translation, and ultimately, cellular function . 5moU is one of these chemical modifications that add an additional layer of complexity to the regulatory landscape .
tRNA Molecule Constituent
5-Methoxyuridine is a constituent of the first position of the anticodon of select bacterial tRNA molecules . It may be used to study the effects of substitutions on the codon reading efficiencies of tRNAs .
Biosynthesis Research
The uridine-5-0-derivatives, 5-methoxyuridine (mo5U) and uridine-5-oxy-acetic acid (cmo5U) occupy the first position of anticodons in certain tRNA species of B. subtilis and E. coli, respectively . Both modifications are derived from a common precursor, 5-hydroxyuridine .
Wirkmechanismus
Target of Action
5-Methoxyuridine (mo5U) is a modified nucleoside that primarily targets the anticodon wobble position in several tRNAs from Gram-negative bacteria . This position plays a critical role in the precise decoding of genetic codes .
Mode of Action
The interaction of 5-Methoxyuridine with its targets results in non-Watson–Crick base pairing with guanosine and pyrimidines at the third positions of codons . This expands the decoding capabilities of the tRNA, allowing for more flexibility in protein synthesis .
Biochemical Pathways
The presence of 5-Methoxyuridine in tRNA affects the biochemical pathway of protein synthesis by modulating codon recognition and ensuring accurate translation of the genetic code . It is a major modification in tRNA Ala1, tRNA Ser1, tRNA Pro3, and tRNA Thr4 .
Pharmacokinetics
It’s known that the terminal methylation frequency of mcmo5u in trna pro3 is low (≈30%) in the early log phase of cell growth, gradually increases as growth proceeds, and reaches nearly 100% in late log and stationary phases .
Result of Action
The result of 5-Methoxyuridine’s action is an enhanced translational fidelity by the ribosome . This is achieved through its ability to facilitate non-Watson–Crick base pairing, thereby expanding the decoding capabilities of the tRNA .
Action Environment
The action of 5-Methoxyuridine can be influenced by various environmental factors. For instance, the terminal methylation frequency of mcmo5U in tRNA Pro3 varies depending on the growth phase of the cell . .
Eigenschaften
IUPAC Name |
1-[(2R,3R,4S,5R)-3,4-dihydroxy-5-(hydroxymethyl)oxolan-2-yl]-5-methoxypyrimidine-2,4-dione | |
---|---|---|
Source | PubChem | |
URL | https://pubchem.ncbi.nlm.nih.gov | |
Description | Data deposited in or computed by PubChem | |
InChI |
InChI=1S/C10H14N2O7/c1-18-4-2-12(10(17)11-8(4)16)9-7(15)6(14)5(3-13)19-9/h2,5-7,9,13-15H,3H2,1H3,(H,11,16,17)/t5-,6-,7-,9-/m1/s1 | |
Source | PubChem | |
URL | https://pubchem.ncbi.nlm.nih.gov | |
Description | Data deposited in or computed by PubChem | |
InChI Key |
ZXIATBNUWJBBGT-JXOAFFINSA-N | |
Source | PubChem | |
URL | https://pubchem.ncbi.nlm.nih.gov | |
Description | Data deposited in or computed by PubChem | |
Canonical SMILES |
COC1=CN(C(=O)NC1=O)C2C(C(C(O2)CO)O)O | |
Source | PubChem | |
URL | https://pubchem.ncbi.nlm.nih.gov | |
Description | Data deposited in or computed by PubChem | |
Isomeric SMILES |
COC1=CN(C(=O)NC1=O)[C@H]2[C@@H]([C@@H]([C@H](O2)CO)O)O | |
Source | PubChem | |
URL | https://pubchem.ncbi.nlm.nih.gov | |
Description | Data deposited in or computed by PubChem | |
Molecular Formula |
C10H14N2O7 | |
Source | PubChem | |
URL | https://pubchem.ncbi.nlm.nih.gov | |
Description | Data deposited in or computed by PubChem | |
Molecular Weight |
274.23 g/mol | |
Source | PubChem | |
URL | https://pubchem.ncbi.nlm.nih.gov | |
Description | Data deposited in or computed by PubChem | |
Product Name |
5-Methoxyuridine | |
CAS RN |
35542-01-9 | |
Record name | 5-Methoxyuridine | |
Source | CAS Common Chemistry | |
URL | https://commonchemistry.cas.org/detail?cas_rn=35542-01-9 | |
Description | CAS Common Chemistry is an open community resource for accessing chemical information. Nearly 500,000 chemical substances from CAS REGISTRY cover areas of community interest, including common and frequently regulated chemicals, and those relevant to high school and undergraduate chemistry classes. This chemical information, curated by our expert scientists, is provided in alignment with our mission as a division of the American Chemical Society. | |
Explanation | The data from CAS Common Chemistry is provided under a CC-BY-NC 4.0 license, unless otherwise stated. | |
Record name | 5-Methoxyuridine | |
Source | ChemIDplus | |
URL | https://pubchem.ncbi.nlm.nih.gov/substance/?source=chemidplus&sourceid=0035542019 | |
Description | ChemIDplus is a free, web search system that provides access to the structure and nomenclature authority files used for the identification of chemical substances cited in National Library of Medicine (NLM) databases, including the TOXNET system. | |
Record name | 5-methoxyuridine | |
Source | European Chemicals Agency (ECHA) | |
URL | https://echa.europa.eu/substance-information/-/substanceinfo/100.047.812 | |
Description | The European Chemicals Agency (ECHA) is an agency of the European Union which is the driving force among regulatory authorities in implementing the EU's groundbreaking chemicals legislation for the benefit of human health and the environment as well as for innovation and competitiveness. | |
Explanation | Use of the information, documents and data from the ECHA website is subject to the terms and conditions of this Legal Notice, and subject to other binding limitations provided for under applicable law, the information, documents and data made available on the ECHA website may be reproduced, distributed and/or used, totally or in part, for non-commercial purposes provided that ECHA is acknowledged as the source: "Source: European Chemicals Agency, http://echa.europa.eu/". Such acknowledgement must be included in each copy of the material. ECHA permits and encourages organisations and individuals to create links to the ECHA website under the following cumulative conditions: Links can only be made to webpages that provide a link to the Legal Notice page. | |
Retrosynthesis Analysis
AI-Powered Synthesis Planning: Our tool employs the Template_relevance Pistachio, Template_relevance Bkms_metabolic, Template_relevance Pistachio_ringbreaker, Template_relevance Reaxys, Template_relevance Reaxys_biocatalysis model, leveraging a vast database of chemical reactions to predict feasible synthetic routes.
One-Step Synthesis Focus: Specifically designed for one-step synthesis, it provides concise and direct routes for your target compounds, streamlining the synthesis process.
Accurate Predictions: Utilizing the extensive PISTACHIO, BKMS_METABOLIC, PISTACHIO_RINGBREAKER, REAXYS, REAXYS_BIOCATALYSIS database, our tool offers high-accuracy predictions, reflecting the latest in chemical research and data.
Strategy Settings
Precursor scoring | Relevance Heuristic |
---|---|
Min. plausibility | 0.01 |
Model | Template_relevance |
Template Set | Pistachio/Bkms_metabolic/Pistachio_ringbreaker/Reaxys/Reaxys_biocatalysis |
Top-N result to add to graph | 6 |
Feasible Synthetic Routes
Q & A
A: 5-Methoxyuridine primarily functions as a modified nucleoside found in the anticodon loop of certain tRNA molecules, particularly in Bacillus subtilis. [, , ] Its primary target is the ribosome during translation. While the precise mechanism of interaction needs further investigation, research suggests that the presence of 5-Methoxyuridine at the wobble position of the anticodon influences codon recognition and enhances the reading efficiency of specific codons, ultimately impacting protein synthesis. [, ]
ANone: 5-Methoxyuridine is a pyrimidine nucleoside composed of a ribose sugar linked to a 5-methoxyuracil base.
ANone: While the provided research focuses heavily on the biological roles of 5-Methoxyuridine, its material compatibility and stability under various conditions haven't been extensively explored within these studies. Further research is needed to understand its behavior outside a biological context.
A: 5-Methoxyuridine itself doesn't function as a catalyst. Its primary role lies in modulating tRNA function during translation, not in catalyzing chemical reactions. [, , ]
A: While the provided research doesn't delve deeply into computational studies of 5-Methoxyuridine, the structural information gleaned from X-ray crystallography of related enzymes like CmoM provides valuable insights into its interactions with tRNA modifying enzymes. [] This structural data can potentially lay the foundation for future computational modeling and simulation studies.
ANone: Information regarding the stability and formulation of 5-Methoxyuridine as a standalone compound isn't explicitly discussed in the provided research.
ANone: The provided research primarily focuses on the fundamental biological role and biosynthesis of 5-Methoxyuridine as a tRNA modification in bacteria. Therefore, these specific aspects related to its pharmaceutical development, safety, and broader applications haven't been addressed within these studies.
A: The discovery of 5-Methoxyuridine as a novel tRNA modification in Bacillus subtilis marked a significant milestone in the field. [, ] Subsequently, research unveiled its role in codon recognition and highlighted its importance in translational fidelity. [, ] The identification of genes involved in its biosynthesis further advanced our understanding of this unique modification. [, ]
A: Research on 5-Methoxyuridine has fostered collaborations between biochemistry, molecular biology, and analytical chemistry. Understanding its biosynthesis has uncovered novel enzymatic reactions and metabolic pathways in bacteria. [, ] Furthermore, the development of analytical methods to detect and quantify 5-Methoxyuridine in RNA has implications for studying RNA modifications in diverse biological contexts. [] The use of 5-Methoxyuridine in mRNA engineering for therapeutic applications also highlights its potential in biomedicine. [, ]
Haftungsausschluss und Informationen zu In-Vitro-Forschungsprodukten
Bitte beachten Sie, dass alle Artikel und Produktinformationen, die auf BenchChem präsentiert werden, ausschließlich zu Informationszwecken bestimmt sind. Die auf BenchChem zum Kauf angebotenen Produkte sind speziell für In-vitro-Studien konzipiert, die außerhalb lebender Organismen durchgeführt werden. In-vitro-Studien, abgeleitet von dem lateinischen Begriff "in Glas", beinhalten Experimente, die in kontrollierten Laborumgebungen unter Verwendung von Zellen oder Geweben durchgeführt werden. Es ist wichtig zu beachten, dass diese Produkte nicht als Arzneimittel oder Medikamente eingestuft sind und keine Zulassung der FDA für die Vorbeugung, Behandlung oder Heilung von medizinischen Zuständen, Beschwerden oder Krankheiten erhalten haben. Wir müssen betonen, dass jede Form der körperlichen Einführung dieser Produkte in Menschen oder Tiere gesetzlich strikt untersagt ist. Es ist unerlässlich, sich an diese Richtlinien zu halten, um die Einhaltung rechtlicher und ethischer Standards in Forschung und Experiment zu gewährleisten.