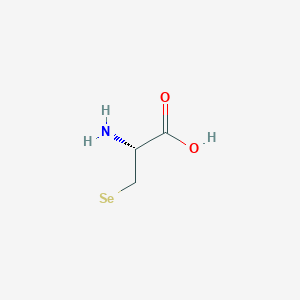
Selenocysteine
Übersicht
Beschreibung
Selenocysteine, recognized as the 21st genetically encoded amino acid, is found in enzymes known as selenoproteins, typically located in their active site[“][“]. The incorporation of Selenocysteine into proteins requires specialized biosynthesis and recoding machinery that evolved distinctly in bacteria compared to archaea and eukaryotes[“]. The UGA codon, normally a stop, is recoded for Selenocysteine insertion, through a highly regulated process involving various cis-signals and trans-factors[“].
Selenocysteine plays a role in redox enzymes that are essential to human health and important targets in disease[“]. It is an essential amino acid for certain species, such as humans and other vertebrates, although it has disappeared from others, such as insects[“]. Researchers have discovered that it is also preserved in fungi, contrary to existing belief[“].
The study of Selenocysteine covers its biosynthesis and peculiar insertion, the function and regulation of specific selenoproteins, their role in human health and disease, the evolution and phylogenetic distribution of Selenocysteine, and the biochemical nature of its catalytic benefit[“].
Synthetic Analysis
Reaction Equation The terminal reaction of selenocysteine synthesis is catalyzed by selenocysteine synthases, SelA in bacteria and SepSecS in archaea and eukaryotes. During this process, either serine or phosphoserine is converted into selenocysteine while being attached to tRNA Sec[“].
Reaction Conditions The synthesis of selenocysteine takes advantage of the electrophilic character of an oxidized selenocysteine (Se−S bond) to react with a nucleophilic arylboronic acid to provide the arylated selenocysteine within hours[“]. This reaction is amenable to a wide range of boronic acids with different biorelevant functional groups[“].
Reaction Steps The synthesis of selenocysteine involves several steps. It begins with the conversion of serine or phosphoserine into selenocysteine while being attached to tRNA Sec. This process is catalyzed by the enzyme serine palmitoyl transferase[“].
Reaction Mechanism The reaction mechanism of selenocysteine synthesis is based on the PLP co-factor and requires selenophosphate as the selenium donor[“]. Although adopting different structures, SelA and SepSecS employ similar general principles for substrate recognition and chemical catalysis[“].
Molecular Structure
Selenocysteine (Sec) is present in several enzymes, including glutathione peroxidases, tetraiodothyronine 5′ deiodinases, thioredoxin reductases, formate dehydrogenases, glycine reductases, selenophosphate synthetase, methionine-R-sulfoxide reductase B1 (SEPX1), and some hydrogenases[“][“][“]. It plays an indispensable role in human nutrition and has been implicated to have important health benefits[“].
Atomic Arrangement Selenocysteine has the same structure as cysteine, but with an atom of selenium taking the place of the usual sulfur[“].
Bonding Type Selenocysteine is the Se-analogue of cysteine. It is rarely encountered outside of living tissue because it is very susceptible to air-oxidation. More common is the oxidized derivative selenocystine, which has an Se-Se bond. The selenium valence electronic configuration is equivalent to that of sulfur, which explains their similar atomic and physicochemical properties[“].
Geometry Selenocysteine has the same structure as cysteine, but with an atom of selenium taking the place of the usual sulfur. It has a selenol group[“][“].
Electron Cloud Distribution The electron cloud distribution of selenocysteine is not explicitly mentioned in the search results. However, given its similarity to cysteine, it can be inferred that the electron cloud is likely distributed around the selenium atom and the surrounding atoms in a similar manner.
Stereochemistry Like other natural proteinogenic amino acids, cysteine and selenocysteine have L chirality in the older D/L notation based on homology to D- and L-glyceraldehyde[“].
Resonance Structure The resonance structures of selenocysteine are not explicitly mentioned in the search results. However, given its structural similarity to cysteine, it can be inferred that selenocysteine may have similar resonance structures, with the selenium atom playing a similar role to the sulfur atom in cysteine.
Wirkmechanismus
Target of Action Selenocysteine is found in tRNAs and in the catalytic site of some enzymes. The genes for glutathione peroxidase and formate dehydrogenase contain the TGA codon, which codes for this amino acid[“]. It is also found in selenoproteins, which contain selenocysteine residues[“].
Mode of Action Selenocysteine is unique in that it acts exclusively on L-selenocysteine but not on its sulfur counterpart, L-cysteine. The enzyme is proposed to function not only in the recycling of selenium via degradation of L-selenocysteine derived from selenoproteins, but also in energy metabolism linked to obesity and metabolic syndrome[“].
Result of Action The action of selenocysteine leads to the formation of various selenoproteins, including glutathione peroxidases, tetraiodothyronine 5′ deiodinases, thioredoxin reductases, formate dehydrogenases, glycine reductases, selenophosphate synthetase 2, methionine-R-sulfoxide reductase B1 (SEPX1), and some hydrogenases. These selenoproteins play crucial roles in various biological processe[“].
Physical Properties
Chemical Properties
Chemical Reaction Type Selenocysteine is the selenium analogue of cysteine. It is rarely encountered outside of living tissue because it is very susceptible to air-oxidation. More common is the oxidized derivative selenocystine, which has an Se-Se bond[“].
Reactivity Selenocysteine is unique in that it acts exclusively on L-selenocysteine but not on its sulfur counterpart, L-cysteine. The enzyme is proposed to function not only in the recycling of selenium via degradation of L-selenocysteine derived from selenoproteins, but also in energy metabolism linked to obesity and metabolic syndrome[“].
Redox Property Selenocysteine is involved in various redox reactions. For example, proteins containing selenosulfide (Se-S) or diselenide (Se-Se) bonds could have high conformational stability in a reducing environment[“]. Selenoproteins containing the CXXU motif (SelH, M, T, V, and W) are putative oxidoreductases that participate in various cellular processes depending on redox regulation[“].
Acidity and Alkalinity The Se-H group in selenocysteine is more acidic (pKa = 5.431) than the thiol group; thus, it is deprotonated at physiological pH[“].
Stability Selenocysteine is stable under certain conditions. For example, Sec–SeI4 is stable in air and can be manipulated as a compound having “shelf-stability”[“]. However, it is very susceptible to air-oxidation[“].
Toxicity Selenium is a vital trace element present as selenocysteine in proteins that are, thus, known as selenoproteins. However, owing to the duality of selenium[“].
Biochemical Properties
Cellular Effects Selenocysteine has a wide range of cellular functions including regulation of selenium transport, thyroid hormones, immunity, and redox homeostasis. Selenium deficiency contributes to various diseases, such as cardiovascular disease, cancer, liver disease, and arthropathy. Selenium supplementation, for the most part, is immunostimulatory, which is measured by a wide range of parameters including T cell proliferation, NK cell activity, innate immune cell functions, and many others[“].
Molecular Mechanism Selenocysteine is unique in that it acts exclusively on L-selenocysteine but not on its sulfur counterpart, L-cysteine[“]. The enzyme is proposed to function not only in the recycling of selenium via degradation of L-selenocysteine derived from selenoproteins, but also in energy metabolism linked to obesity and metabolic syndrome. It is found in tRNAs and in the catalytic site of some enzymes[“].
Time Effect The effect of selenocysteine over time is not explicitly mentioned in the search results. However, it is known that the biological effects of selenium are largely mediated by selenium-containing proteins (selenoproteins) that are present in all three domains of life. Most of these proteins contain at least one selenocysteine (Sec), a selenium-containing amino acid, and most serve oxidoreductase functions[“].
Wissenschaftliche Forschungsanwendungen
Selenoproteins Biosynthesis
Sec is the 21st genetically encoded amino acid. It is incorporated into proteins, known as selenoproteins, through a specialized biosynthesis and recoding machinery. This process has evolved distinctly in bacteria compared to archaea and eukaryotes[“].
Genetic Code Expansion
The genetic code expansion efforts have been highlighted recently to engineer site-directed incorporation of Sec in bacteria and yeast[“]. This allows for the production of selenoproteins with 21 or more amino acids[“].
Redox Enzymes
Sec plays a crucial role in redox enzymes that are essential to human health and important targets in disease[“]. These enzymes are typically located in their active site.
Synthetic Biology
The delivery of recombinant selenoproteins to mammalian cells opens up new applications for selenoproteins in synthetic biology[“]. This involves the production and delivery of selenoproteins with 21 or more amino acids[“].
Cancer Research and Disease Treatment
Selenols, including Sec and hydrogen selenide (H2Se), play a key role in cancer research and disease treatment3. They are important reducing substances similar to thiols[“].
Soil Microbiology
The application of Sec significantly increases the number of soil microorganisms (bacteria and fungi) in certain forest ecosystems. This may be due to the direct utilization of Sec by microorganisms[“].
Zukünftige Richtungen
Bioinformatics and Selenium Research
Future research may focus on using omics technologies to study selenoproteins and the mechanisms of selenocysteine incorporation in various biological systems. This approach will enhance understanding of selenocysteine insertion and regulation of selenoprotein synthesis in mammalian cells (Santesmasses, Mariotti, & Gladyshev, 2020).Research on selenocysteine lyase
Future studies may examine the structure and mechanism of selenocysteine lyase in more depth, with a focus on elucidating its physiological role in mammals. This will help to understand how this enzyme differentiates between selenocysteine and cysteine (Mihara & Esaki, 2011).Selenium and brain diseases
Studying the role of selenoproteins, especially in the brain, is an important future direction. Given that selenium concentrations in the brain remain constant even in the presence of dietary deficiency, upcoming research may focus on the role of selenium and selenoproteins in neurological function and diseases such as Alzheimer's and Parkinson's disease (Chen & Berry, 2003).Selenium Phytoremediation
Genetic engineering to enhance selenium phytoremediation is a promising field. Future research may focus on developing transgenic plants to improve the ability to tolerate, accumulate and volatilize selenium, thus promoting environmental sustainability (Leduc et al., 2006).Proteomics and selenocysteine identification
The field of proteomics has great potential for the identification of selenocysteine-containing peptides. Future studies can utilize proteomic data to identify selenoproteins, which will enhance understanding of the biological role of selenium.Eigenschaften
InChI |
InChI=1S/C3H6NO2Se/c4-2(1-7)3(5)6/h2H,1,4H2,(H,5,6)/t2-/m0/s1 | |
---|---|---|
Source | PubChem | |
URL | https://pubchem.ncbi.nlm.nih.gov | |
Description | Data deposited in or computed by PubChem | |
InChI Key |
FDKWRPBBCBCIGA-REOHCLBHSA-N | |
Source | PubChem | |
URL | https://pubchem.ncbi.nlm.nih.gov | |
Description | Data deposited in or computed by PubChem | |
Canonical SMILES |
C(C(C(=O)O)N)[Se] | |
Source | PubChem | |
URL | https://pubchem.ncbi.nlm.nih.gov | |
Description | Data deposited in or computed by PubChem | |
Isomeric SMILES |
C([C@@H](C(=O)O)N)[Se] | |
Source | PubChem | |
URL | https://pubchem.ncbi.nlm.nih.gov | |
Description | Data deposited in or computed by PubChem | |
Molecular Formula |
C3H6NO2Se | |
Source | PubChem | |
URL | https://pubchem.ncbi.nlm.nih.gov | |
Description | Data deposited in or computed by PubChem | |
DSSTOX Substance ID |
DTXSID00881371 | |
Record name | L-Selenocysteine | |
Source | EPA DSSTox | |
URL | https://comptox.epa.gov/dashboard/DTXSID00881371 | |
Description | DSSTox provides a high quality public chemistry resource for supporting improved predictive toxicology. | |
Molecular Weight |
167.06 g/mol | |
Source | PubChem | |
URL | https://pubchem.ncbi.nlm.nih.gov | |
Description | Data deposited in or computed by PubChem | |
Product Name |
Selenocysteine | |
CAS RN |
10236-58-5 | |
Record name | Selenocysteine | |
Source | ChemIDplus | |
URL | https://pubchem.ncbi.nlm.nih.gov/substance/?source=chemidplus&sourceid=0010236585 | |
Description | ChemIDplus is a free, web search system that provides access to the structure and nomenclature authority files used for the identification of chemical substances cited in National Library of Medicine (NLM) databases, including the TOXNET system. | |
Record name | Selenocysteine | |
Source | DrugBank | |
URL | https://www.drugbank.ca/drugs/DB02345 | |
Description | The DrugBank database is a unique bioinformatics and cheminformatics resource that combines detailed drug (i.e. chemical, pharmacological and pharmaceutical) data with comprehensive drug target (i.e. sequence, structure, and pathway) information. | |
Explanation | Creative Common's Attribution-NonCommercial 4.0 International License (http://creativecommons.org/licenses/by-nc/4.0/legalcode) | |
Record name | L-Selenocysteine | |
Source | EPA DSSTox | |
URL | https://comptox.epa.gov/dashboard/DTXSID00881371 | |
Description | DSSTox provides a high quality public chemistry resource for supporting improved predictive toxicology. | |
Record name | 10236-58-5 | |
Source | European Chemicals Agency (ECHA) | |
URL | https://echa.europa.eu/information-on-chemicals | |
Description | The European Chemicals Agency (ECHA) is an agency of the European Union which is the driving force among regulatory authorities in implementing the EU's groundbreaking chemicals legislation for the benefit of human health and the environment as well as for innovation and competitiveness. | |
Explanation | Use of the information, documents and data from the ECHA website is subject to the terms and conditions of this Legal Notice, and subject to other binding limitations provided for under applicable law, the information, documents and data made available on the ECHA website may be reproduced, distributed and/or used, totally or in part, for non-commercial purposes provided that ECHA is acknowledged as the source: "Source: European Chemicals Agency, http://echa.europa.eu/". Such acknowledgement must be included in each copy of the material. ECHA permits and encourages organisations and individuals to create links to the ECHA website under the following cumulative conditions: Links can only be made to webpages that provide a link to the Legal Notice page. | |
Record name | SELENOCYSTEINE | |
Source | FDA Global Substance Registration System (GSRS) | |
URL | https://gsrs.ncats.nih.gov/ginas/app/beta/substances/0CH9049VIS | |
Description | The FDA Global Substance Registration System (GSRS) enables the efficient and accurate exchange of information on what substances are in regulated products. Instead of relying on names, which vary across regulatory domains, countries, and regions, the GSRS knowledge base makes it possible for substances to be defined by standardized, scientific descriptions. | |
Explanation | Unless otherwise noted, the contents of the FDA website (www.fda.gov), both text and graphics, are not copyrighted. They are in the public domain and may be republished, reprinted and otherwise used freely by anyone without the need to obtain permission from FDA. Credit to the U.S. Food and Drug Administration as the source is appreciated but not required. | |
Melting Point |
143-146 °C | |
Record name | Selenocysteine | |
Source | DrugBank | |
URL | https://www.drugbank.ca/drugs/DB02345 | |
Description | The DrugBank database is a unique bioinformatics and cheminformatics resource that combines detailed drug (i.e. chemical, pharmacological and pharmaceutical) data with comprehensive drug target (i.e. sequence, structure, and pathway) information. | |
Explanation | Creative Common's Attribution-NonCommercial 4.0 International License (http://creativecommons.org/licenses/by-nc/4.0/legalcode) | |
Retrosynthesis Analysis
AI-Powered Synthesis Planning: Our tool employs the Template_relevance Pistachio, Template_relevance Bkms_metabolic, Template_relevance Pistachio_ringbreaker, Template_relevance Reaxys, Template_relevance Reaxys_biocatalysis model, leveraging a vast database of chemical reactions to predict feasible synthetic routes.
One-Step Synthesis Focus: Specifically designed for one-step synthesis, it provides concise and direct routes for your target compounds, streamlining the synthesis process.
Accurate Predictions: Utilizing the extensive PISTACHIO, BKMS_METABOLIC, PISTACHIO_RINGBREAKER, REAXYS, REAXYS_BIOCATALYSIS database, our tool offers high-accuracy predictions, reflecting the latest in chemical research and data.
Strategy Settings
Precursor scoring | Relevance Heuristic |
---|---|
Min. plausibility | 0.01 |
Model | Template_relevance |
Template Set | Pistachio/Bkms_metabolic/Pistachio_ringbreaker/Reaxys/Reaxys_biocatalysis |
Top-N result to add to graph | 6 |
Feasible Synthetic Routes
Q & A
Q1: What makes selenocysteine unique among the 21 proteinogenic amino acids?
A: Unlike the other 20 amino acids, selenocysteine is incorporated into proteins co-translationally in response to a UGA codon, which typically signals translation termination. This process is dependent on a specific mRNA stem-loop structure called the selenocysteine insertion sequence (SECIS) element and a dedicated translational machinery. []
Q2: What is the role of the SECIS element in selenocysteine incorporation?
A: The SECIS element, located in the 3′-UTR of eukaryotic selenoprotein mRNAs, is crucial for recruiting the selenocysteine-specific elongation factor (SelB) and tRNA (tRNASec) needed to reassign the UGA codon from a stop signal to a selenocysteine codon. [, , ]
Q3: How does the efficiency of selenocysteine incorporation compare to that of canonical amino acids?
A: Studies using the phospholipid glutathione peroxidase gene as a model have shown that the efficiency of selenocysteine incorporation is generally much lower than 100%. [] This suggests that selenocysteine incorporation competes with translational termination at UGA codons.
Q4: Can multiple selenocysteine residues be incorporated into a protein in a processive manner?
A: Research suggests that multiple selenocysteine incorporations are unlikely to be processive. The presence of two or three consecutive UGA codons in a reading frame dramatically reduces the yield of full-length protein, indicating that each UGA-selenocysteine decoding event is likely independent. []
Q5: What adaptations have evolved in selenoprotein P to enhance the incorporation of multiple selenocysteines?
A: Selenoprotein P, which requires multiple selenocysteine residues, exhibits two key adaptations: (1) A conserved, inefficiently decoded UGA codon in the N-terminal region acts as a checkpoint for selenocysteine incorporation factors and slows down ribosome progression, potentially allowing more time for Sec-tRNASec recruitment. (2) Introns downstream of this inefficiently decoded UGA may trigger nonsense-mediated decay if selenocysteine incorporation factors are limiting. []
Q6: What is the molecular formula and weight of selenocysteine?
A: Selenocysteine (C3H7NO2Se) has a molecular weight of 168.05 g/mol. [] It is structurally analogous to cysteine, with a selenium atom replacing sulfur.
Q7: How does the reactivity of selenocysteine compare to cysteine, and what is the significance of this difference?
A: Selenocysteine exhibits higher chemical reactivity compared to cysteine due to the lower redox potential of selenium. [, , ] This enhanced reactivity is crucial for the catalytic activity of selenoenzymes, which often display significantly increased reaction rates compared to their cysteine-containing counterparts.
Q8: What is the primary biological function of most selenoproteins?
A: A majority of characterized selenoproteins function as redox-active enzymes, primarily involved in oxidative stress defense. These selenoenzymes utilize the unique properties of selenocysteine at their active sites to catalyze oxidation-reduction reactions. [, ]
Q9: Can you provide examples of specific selenoproteins and their functions?
A:
Glutathione peroxidases (GPx): These enzymes reduce hydrogen peroxide and lipid hydroperoxides, protecting cells from oxidative damage. Plasma glutathione peroxidase (GPx-3) is one example. [] * Thioredoxin reductases (TrxR): These enzymes are crucial for maintaining cellular redox balance and are involved in various processes, including hydroperoxide metabolism and deoxynucleotide biosynthesis. [] * Iodothyronine deiodinases:* These enzymes are involved in thyroid hormone metabolism, playing a vital role in regulating thyroid hormone levels. [, ]
Q10: What is the role of selenocysteine lyase (SCL) in selenium metabolism?
A: SCL specifically catalyzes the decomposition of selenocysteine into L-alanine and elemental selenium. [, ] While its exact role in vivo is still being investigated, evidence suggests SCL is involved in recycling selenium from degraded selenoproteins, particularly in tissues with high selenoprotein demand, such as the kidney and liver. []
Q11: How does the substrate specificity of selenocysteine lyase contribute to selenium homeostasis?
A: Selenocysteine lyase exhibits strict substrate specificity for L-selenocysteine and shows no activity towards L-cysteine. [] This specificity is crucial for preventing the depletion of essential cysteine pools while allowing the recycling of selenium from selenocysteine.
Q12: What structural features of selenocysteine lyase contribute to its substrate specificity?
A: Studies on rat selenocysteine lyase have revealed that a cysteine residue (Cys-375) located on a flexible loop plays a critical role in substrate recognition. This residue helps to correctly position and orient L-selenocysteine within the active site, while L-cysteine forms a non-productive adduct with the enzyme. []
Q13: What is the role of selenocysteine methyltransferase (SMT) in selenium metabolism in some plant species?
A: SMT catalyzes the methylation of selenocysteine to form methylselenocysteine, a less toxic and more readily accumulated form of selenium. This process is particularly important for selenium-tolerant plants like Astragalus bisulcatus, where SMT contributes to selenium detoxification and tolerance. []
Q14: What is the physiological methyl group donor for selenocysteine methyltransferase?
A: Unlike many other methyltransferases that utilize S-adenosylmethionine, selenocysteine methyltransferase primarily uses S-methylmethionine as its methyl group donor. []
Q15: How does the expression of selenocysteine methyltransferase affect selenium tolerance in biological systems?
A: Experiments using E. coli as a model system demonstrated that overexpressing either plant-derived or bacterial selenocysteine methyltransferase significantly increases selenium tolerance. This effect is dependent on the presence of S-methylmethionine in the growth medium. []
Q16: What is the significance of the zinc-binding motif and conserved cysteine residue in selenocysteine methyltransferase from Astragalus chrysochlorus?
A: While the exact function of these structural features is not fully understood, their conservation suggests a potential role in enzyme activity or regulation. The zinc-binding motif (GGCC) and the upstream cysteine residue might be involved in metal ion binding, which could influence enzyme conformation or catalytic activity. []
Q17: How does selenocysteine incorporation differ between prokaryotes and eukaryotes?
A: The most significant difference lies in the location of the SECIS element. In prokaryotes, it is typically located immediately downstream of the UGA codon within the coding sequence. In eukaryotes, it resides in the 3′-UTR, distant from the UGA codon. [, ]
Q18: How can the efficiency of UGA recoding as selenocysteine be manipulated experimentally?
A: Researchers have successfully used CRISPR-Cas9 technology to target and inactivate the Sec-tRNA[Ser]Sec gene in human cell lines. This approach leads to reduced levels of Sec-tRNA[Ser]Sec, effectively decreasing the efficiency of UGA-selenocysteine recoding by the ribosome. This strategy offers a valuable tool for studying the effects of selenoprotein deficiency in various cell types. []
Q19: What is the potential of selenocysteine incorporation for protein engineering?
A: The ability to site-specifically incorporate selenocysteine holds promise for engineering proteins with novel properties. For example, the introduction of selenocysteine can facilitate the formation of selenyl-sulfhydryl and diselenide bonds, expanding the repertoire of protein structures and functions achievable through genetic manipulation. [, ]
Q20: How can mass spectrometry be used to study selenocysteine-containing peptides and proteins?
A: Mass spectrometry techniques, particularly MALDI-TOF and ESI-MS, are valuable tools for analyzing selenoproteins. The unique isotopic signature of selenium allows for the identification and quantification of selenocysteine-containing peptides. These techniques can be used to confirm selenocysteine incorporation, determine selenium isotope ratios, and study the fragmentation patterns of selenoproteins. [, , ]
Q21: What are the potential applications of nuclear magnetic resonance (NMR) spectroscopy in studying selenocysteine-containing proteins?
A: The 77Se isotope is NMR-active, making it a valuable probe for studying the structure, dynamics, and interactions of selenocysteine-containing proteins. 77Se-NMR can provide insights into the local environment of selenocysteine residues, redox state changes, and protein-ligand interactions. []
Q22: What are the potential benefits of replacing cysteine with selenocysteine in peptides and proteins for structural studies?
A: Selenocysteine can serve as an isomorphous replacement for cysteine in X-ray crystallography. The larger electron density of selenium compared to sulfur enhances the anomalous scattering of X-rays, facilitating the determination of protein phases and improving the resolution of crystallographic models. [, ]
Q23: How does the redox potential of selenocysteine influence the oxidative folding of peptides and proteins?
A: The significantly more reducing redox potential of selenocysteine compared to cysteine promotes the preferential formation of diselenide bonds over disulfide bonds in peptides and proteins containing both selenocysteine and cysteine residues. This property can be exploited for directing the regioselective formation of disulfide bonds during oxidative protein folding. [, ]
Q24: Can selenocysteine be incorporated into proteins non-specifically?
A: Unlike selenomethionine, which can be non-specifically incorporated into proteins via the methionine biosynthesis pathway, selenocysteine incorporation appears to be highly specific and dependent on the dedicated selenocysteine insertion machinery. Studies using radiolabeled selenocysteine in rats have not shown evidence of its non-specific incorporation into proteins like albumin. []
Q25: What is the role of selenophosphate in selenocysteine biosynthesis?
A: Selenophosphate, synthesized by selenophosphate synthetase, serves as the selenium donor for the conversion of seryl-tRNASec to selenocysteyl-tRNASec by selenocysteine synthase. The high specificity of selenophosphate synthetase for selenide ensures the accurate and efficient delivery of selenium for selenocysteine biosynthesis. []
Q26: What are the key enzymes involved in the selenocysteine biosynthesis pathway?
A: The core enzymes in the pathway include:* Phosphoseryl-tRNASec kinase (PSTK): Phosphorylates seryl-tRNASec. []* O-phosphoseryl-tRNA:selenocysteine synthase (SepSecS): Uses selenophosphate to convert O-phosphoseryl-tRNASec to selenocysteyl-tRNASec. []* Selenophosphate synthetase (SPS or SelD): Synthesizes selenophosphate from selenide. [, ]
Q27: How does the selenocysteine biosynthesis pathway differ in trypanosomatid parasites compared to mammals?
A: While trypanosomatids possess the canonical selenocysteine biosynthesis machinery (PSTK, SepSecS, SelD, and EFSec/SelB), they lack certain factors found in mammals, such as SECIS binding protein 2 (SBP) and SecP43. [] The functional implications of these differences are still under investigation.
Q28: How does selenium supplementation affect the course of trypanosomatid infections?
A: Studies have shown that selenium supplementation can decrease parasitemia and ameliorate disease severity in various Trypanosoma infections. Selenium may exert its beneficial effects by boosting the host's immune response and enhancing antioxidant defenses to combat parasite-induced oxidative stress. []
Q29: How can computational chemistry and modeling contribute to selenocysteine research?
A29: Computational approaches can be employed to:* Model the structure and dynamics of selenoproteins.* Simulate the catalytic mechanisms of selenoenzymes.* Predict the impact of selenocysteine mutations on protein structure and function.* Design and optimize novel selenocysteine-containing peptides and proteins with desired properties.
Q30: What are the environmental implications of selenium methylation by freshwater bacteria?
A: The methylation of selenium by bacteria in freshwater environments plays a significant role in the biogeochemical cycling of this element. Volatile methylated selenium species, such as dimethyl selenide (DMSe) and dimethyl diselenide (DMDSe), can escape into the atmosphere, influencing global selenium distribution. []
Q31: What is the role of the bacterial thiopurine methyltransferase (bTPMT) in selenium methylation?
A: bTPMT, traditionally known for its role in xenobiotic metabolism, has been shown to contribute to selenium methylation in some freshwater bacteria. These bacteria can produce DMSe and DMDSe when grown in the presence of selenium, and this activity is dependent on a functional bTPMT gene (tpm). []
Q32: How does the abundance of bTPMT-containing bacteria impact selenium methylation in freshwater environments?
A: Studies have demonstrated a positive correlation between the population size of bTPMT-containing bacteria and the emission of methylated selenium species from freshwater samples supplemented with selenium. This finding highlights the significant contribution of these bacteria to selenium methylation in natural aquatic ecosystems. []
Haftungsausschluss und Informationen zu In-Vitro-Forschungsprodukten
Bitte beachten Sie, dass alle Artikel und Produktinformationen, die auf BenchChem präsentiert werden, ausschließlich zu Informationszwecken bestimmt sind. Die auf BenchChem zum Kauf angebotenen Produkte sind speziell für In-vitro-Studien konzipiert, die außerhalb lebender Organismen durchgeführt werden. In-vitro-Studien, abgeleitet von dem lateinischen Begriff "in Glas", beinhalten Experimente, die in kontrollierten Laborumgebungen unter Verwendung von Zellen oder Geweben durchgeführt werden. Es ist wichtig zu beachten, dass diese Produkte nicht als Arzneimittel oder Medikamente eingestuft sind und keine Zulassung der FDA für die Vorbeugung, Behandlung oder Heilung von medizinischen Zuständen, Beschwerden oder Krankheiten erhalten haben. Wir müssen betonen, dass jede Form der körperlichen Einführung dieser Produkte in Menschen oder Tiere gesetzlich strikt untersagt ist. Es ist unerlässlich, sich an diese Richtlinien zu halten, um die Einhaltung rechtlicher und ethischer Standards in Forschung und Experiment zu gewährleisten.