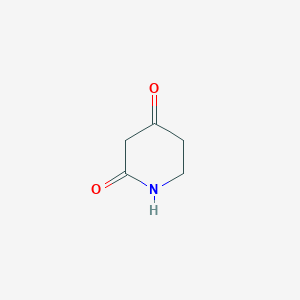
2,4-Piperidinedione
Übersicht
Beschreibung
2,4-Piperidinedione is a cyclic diketone derivative of piperidine, characterized by two ketone groups at the 2- and 4-positions of the six-membered ring. This structure confers unique reactivity due to the electron-withdrawing effects of the carbonyl groups and the presence of a lactam moiety (one nitrogen atom in the ring) . The compound is of significant interest in organic and medicinal chemistry due to its role as a precursor in synthesizing bioactive molecules and its participation in nucleophilic reactions with electrophilic species like azanone (HNO) . Derivatives of this compound, such as 3,3-diethyl-2,4-piperidinedione, are utilized in pharmaceutical applications, including sedatives and anticonvulsants .
Vorbereitungsmethoden
Synthetic Routes and Reaction Conditions: The synthesis of 2,4-Piperidinedione typically involves the reaction of methyl 2,4-dioxo-piperidine-3-carboxylate with a mixture of acetonitrile and water. The solution is heated to approximately 86°C and maintained for around 4 hours. After the reaction, the solvent is removed under vacuum, and the residue is purified using silica gel column chromatography with a mobile phase of dichloromethane and methanol in a ratio of 100:1. The final product is obtained as a white solid with a yield of 27% .
Industrial Production Methods: In industrial settings, this compound can be synthesized from tert-butyl 2,4-dioxopiperidine-1-carboxylate. This involves adding trifluoroacetic acid to a solution of the tert-butyl ester in dichloromethane at 0°C, followed by stirring at room temperature for 1 hour. The reaction is monitored by thin-layer chromatography .
Analyse Chemischer Reaktionen
Types of Reactions: 2,4-Piperidinedione undergoes various chemical reactions, including:
Oxidation: It can be oxidized to form more complex piperidine derivatives.
Reduction: Reduction reactions can convert the ketone groups to alcohols.
Substitution: The compound can participate in nucleophilic substitution reactions, where the ketone groups are replaced by other functional groups.
Common Reagents and Conditions:
Oxidation: Common oxidizing agents include potassium permanganate and chromium trioxide.
Reduction: Sodium borohydride and lithium aluminum hydride are frequently used reducing agents.
Substitution: Reagents like Grignard reagents and organolithium compounds are used for nucleophilic substitution.
Major Products: The major products formed from these reactions include various substituted piperidines and piperidine derivatives, which are valuable intermediates in pharmaceutical synthesis .
Wissenschaftliche Forschungsanwendungen
Medicinal Chemistry
Pharmaceutical Applications:
2,4-Piperidinedione derivatives are increasingly recognized for their potential in drug development. They serve as precursors for synthesizing a wide range of piperidine-based pharmacophores, which are integral to numerous therapeutic agents. These derivatives have been incorporated into various classes of pharmaceuticals, including antipsychotics and analgesics .
Biological Activity:
Research indicates that this compound exhibits notable biological activity. For instance, its derivatives have shown promise in treating conditions such as Alzheimer's disease due to their ability to inhibit acetylcholinesterase, an enzyme linked to cognitive decline . Additionally, compounds derived from this compound have been evaluated for their antimicrobial properties and potential use in cancer therapy .
Synthetic Methodologies
Synthesis of Derivatives:
The synthesis of this compound derivatives can be achieved through various methodologies. Recent advancements include:
- Organophotocatalysis: A novel one-step organophotocatalyzed method has been developed for synthesizing diverse substituted 2-piperidinones from readily available starting materials. This method offers high selectivity and efficiency, reducing the need for multi-step processes .
- Anionic Enolate Rearrangements: This approach enables the construction of structurally diverse piperidine-type systems from simple carbonyl compounds. Such transformations are crucial for creating complex molecules with potential medicinal properties .
Case Studies
Case Study 1: Drug Development
A study published in 2020 highlighted the synthesis of piperidine-2,4-dione-type azaheterocycles that demonstrated significant activity against various biological targets. The research emphasized the utility of these compounds in developing new therapeutic agents with enhanced efficacy and reduced side effects .
Case Study 2: Natural Product Synthesis
Another application involves the use of this compound in synthesizing natural products. The compound serves as a key intermediate in constructing complex natural products that have been shown to possess significant pharmacological activities .
Summary Table of Applications
Wirkmechanismus
The mechanism of action of 2,4-Piperidinedione involves its interaction with specific molecular targets and pathways. For instance, in medicinal chemistry, it acts as a precursor to drugs that modulate enzyme activity or receptor function. The compound’s structure allows it to bind to active sites of enzymes or receptors, thereby influencing their activity and leading to therapeutic effects .
Vergleich Mit ähnlichen Verbindungen
Structural and Reactivity Comparisons
1,3-Cyclohexanedione
- Reactivity: Exhibits lower nucleophilic reactivity toward HNO compared to 2,4-piperidinedione. The second-order rate constant ($k$) for 1,3-cyclohexanedione is $2.2 \times 10^3 \, \text{M}^{-1} \text{s}^{-1}$, whereas this compound shows a ninefold increase ($k = 2.0 \times 10^4 \, \text{M}^{-1} \text{s}^{-1}$) .
- Mechanistic Insight: The absence of nitrogen atoms reduces resonance stabilization in the enolate intermediate, leading to lower reactivity .
Barbituric Acid (2,4,6-Pyrimidinetrione)
- Structure : Contains three carbonyl groups and two nitrogen atoms in a six-membered ring.
- Reactivity: Despite structural similarities, barbituric acid exhibits significantly lower reactivity ($k = 2.2 \times 10^3 \, \text{M}^{-1} \text{s}^{-1}$) compared to this compound. This is attributed to greater resonance stabilization of the enolate intermediate, which diminishes nucleophilicity .
- Energy Barrier : The calculated energy barrier for barbituric acid (64.65 kJ/mol) is higher than that of this compound (45.65 kJ/mol), reflecting reduced kinetic favorability .
Meldrum’s Acid (2,2-Dimethyl-1,3-dioxane-4,6-dione)
- Reactivity: Demonstrates minimal reactivity toward HNO ($k = 8.7 \times 10^2 \, \text{M}^{-1} \text{s}^{-1}$), likely due to steric hindrance from the methyl groups and lack of nitrogen-mediated electronic effects .
1-(4-Methoxybenzyl)-2,4-Piperidinedione
- Structure : A substituted derivative of this compound with a 4-methoxybenzyl group.
- Reactivity : Shows a sixfold increase in reactivity ($k = 1.4 \times 10^4 \, \text{M}^{-1} \text{s}^{-1}$) compared to 1,3-cyclohexanedione. The electron-donating methoxy group enhances nucleophilicity, though steric effects slightly reduce reactivity compared to the parent compound .
Kinetic and Thermodynamic Data
Compound | Rate Constant ($k$, M⁻¹s⁻¹) | Energy Barrier (kJ/mol) | Key Structural Feature |
---|---|---|---|
This compound | $2.0 \times 10^4$ | 45.65 | Lactam ring with two ketones |
1,3-Cyclohexanedione | $2.2 \times 10^3$ | - | No nitrogen atoms |
Barbituric Acid | $2.2 \times 10^3$ | 64.65 | Three carbonyls, two nitrogens |
Meldrum’s Acid | $8.7 \times 10^2$ | 70.15 | Ester groups, no nitrogen |
1-(4-Methoxybenzyl)-2,4-Piperidinedione | $1.4 \times 10^4$ | 41.34 | Substituted benzyl group |
Source: Kinetic data and energy barriers derived from HNO reactivity studies .
Mechanistic Insights
- Resonance Effects: The lactam structure of this compound reduces resonance stabilization compared to fully conjugated systems like barbituric acid, resulting in a more reactive enolate intermediate .
- Electronic Effects : Substitution with electron-donating groups (e.g., methoxy) enhances nucleophilicity, while electron-withdrawing groups (e.g., acyl) decrease reactivity .
- Steric Factors : Bulky substituents, as seen in Meldrum’s acid, impede access to the reactive site, lowering reaction rates .
Pharmacological Relevance
Structural modifications (e.g., alkylation, aryl substitution) enable fine-tuning of bioactivity, as seen in sedatives like dihyprylon (3,3-diethyl-2,4-piperidinedione) .
Biologische Aktivität
2,4-Piperidinedione, also known as 2,4-dioxopiperidine, is a cyclic compound with significant biological activity, particularly in the fields of medicinal chemistry and pharmacology. This compound has garnered attention due to its potential as an antimicrobial agent and its role in various therapeutic applications. This article reviews the biological activities associated with this compound, supported by data tables and case studies.
Chemical Structure and Properties
The chemical structure of this compound consists of a piperidine ring with two carbonyl groups at the 2 and 4 positions. This unique structure contributes to its diverse biological activities.
Antimicrobial Activity
Recent studies have highlighted the antimicrobial properties of this compound derivatives. For instance, compounds derived from this scaffold have shown promising results against various pathogenic bacteria and fungi.
Case Study: Antibacterial Efficacy
A study published in 2023 examined the antibacterial activity of several piperidine derivatives, including those containing the this compound structure. The results indicated that certain compounds exhibited EC50 values ranging from 0.78 μg/mL to 3.48 μg/mL , demonstrating excellent inhibitory effects on bacterial growth. Notably, compound B14 was identified as particularly effective in disrupting bacterial cell membranes and interfering with fatty acid synthesis pathways .
Compound | EC50 (μg/mL) | Activity |
---|---|---|
B14 | 0.78 | Excellent |
C1 | 1.25 | Good |
B15 | 3.48 | Moderate |
The mechanism by which this compound exerts its biological effects involves several pathways:
- Disruption of Cell Membranes : Compounds derived from this compound can increase membrane permeability and disrupt the integrity of bacterial cell membranes .
- Inhibition of Fatty Acid Synthesis : Research indicates that these compounds can interfere with mRNA expression levels related to fatty acid synthesis genes such as ACC and Fab family genes .
Other Biological Activities
Beyond antimicrobial properties, derivatives of this compound have been investigated for their potential in treating other conditions:
Anticancer Activity
Recent findings suggest that certain piperidine derivatives may possess anticancer properties. For example, an analog of EF24 (a piperidinone derivative) has shown activity against various cancer types by inhibiting IKKb, a key regulator in NF-κB signaling pathways associated with inflammation and cancer progression .
Neuroprotective Effects
In silico studies have predicted that modified piperidine derivatives could affect neurotransmitter systems and voltage-gated ion channels, indicating potential applications in treating central nervous system disorders .
Q & A
Basic Questions
Q. What are the established synthetic routes for 2,4-Piperidinedione, and what key parameters influence reaction yields?
- Methodological Answer : The synthesis of this compound derivatives often involves amide coupling reactions or cyclization of precursor molecules. Key parameters include solvent choice (e.g., dichloromethane for controlled reactivity), temperature (room temperature for stability vs. reflux for faster kinetics), and catalysts (e.g., NaOH for deprotonation steps). Reaction progress should be monitored via TLC or NMR spectroscopy to optimize yields. For example, amide coupling protocols require rigorous exclusion of moisture to prevent hydrolysis .
Q. What safety protocols are recommended for handling this compound in laboratory settings?
- Methodological Answer : Refer to Safety Data Sheets (SDS) for specific guidelines: use PPE (gloves, lab coats, goggles), ensure adequate ventilation, and avoid direct contact. Storage should be in a cool, dry place away from oxidizing agents. In case of spills, neutralize with inert absorbents and dispose of according to hazardous waste regulations .
Q. How to conduct a systematic literature review on this compound’s pharmacological applications using frameworks like PICO?
- Methodological Answer : Apply the PICO framework to structure foreground questions:
- Population/Problem : Biological targets (e.g., enzymes, receptors).
- Intervention : this compound derivatives.
- Comparison : Existing inhibitors or analogs.
- Outcome : Efficacy metrics (e.g., IC50 values).
Use databases like PubMed and SciFinder with Boolean operators (e.g., "this compound AND kinase inhibition") to filter primary studies .
Q. What spectroscopic techniques are essential for characterizing this compound, and what spectral markers should be prioritized?
- Methodological Answer :
- NMR : Look for carbonyl signals (δ ~170–180 ppm in ) and α-proton splitting patterns (δ ~3.0–4.0 ppm in ).
- IR : Stretching vibrations for carbonyl groups (~1650–1750 cm).
- MS : Molecular ion peaks and fragmentation patterns to confirm molecular weight and structural motifs. Ensure purity via HPLC (>95%) .
Q. What are the common pitfalls in experimental design when synthesizing this compound derivatives, and how to mitigate them?
- Methodological Answer : Common issues include by-product formation due to incomplete cyclization and moisture sensitivity. Mitigation strategies:
- Use anhydrous solvents and inert atmospheres (N/Ar).
- Optimize stoichiometry via pilot studies.
- Include control reactions to identify side products .
Advanced Research Questions
Q. How can kinetic studies elucidate the reactivity of this compound with molecular oxygen under varying experimental conditions?
- Methodological Answer : Conduct time-resolved UV-Vis spectroscopy to monitor oxygen consumption rates. Vary concentrations (50–250 μM) and measure absorbance changes at specific wavelengths (e.g., 260 nm). Use pseudo-first-order kinetics to determine rate constants and assess pH dependence (e.g., buffered vs. unbuffered systems) .
Q. What methodologies are effective in resolving contradictions between theoretical predictions and experimental data regarding the compound’s stability?
- Methodological Answer : Perform sensitivity analyses using computational tools (e.g., DFT calculations) to identify discrepancies in reaction pathways. Validate via controlled experiments (e.g., stability under inert vs. oxidative atmospheres) and statistical error analysis (e.g., confidence intervals for degradation rates) .
Q. How to design comparative studies between this compound and its analogs to assess structure-activity relationships (SAR)?
- Methodological Answer :
- Structural Modifications : Introduce substituents at positions 2 and 4 (e.g., methyl, phenyl groups).
- Assays : Test derivatives against target enzymes (e.g., kinases) using fluorometric or calorimetric assays.
- Data Analysis : Correlate electronic (Hammett σ) or steric (Taft parameters) effects with activity trends. Use ANOVA to validate significance .
Q. What strategies optimize the purification of this compound derivatives when dealing with by-products?
- Methodological Answer : Combine column chromatography (silica gel, gradient elution) with recrystallization (solvent pair screening). For polar by-products, use reverse-phase HPLC. Monitor purity at each step via NMR integration and LC-MS .
Q. How to apply computational modeling to predict this compound’s interactions in biological systems, and validate these predictions experimentally?
- Methodological Answer :
- Docking Studies : Use AutoDock Vina to model binding poses in enzyme active sites (e.g., COX-2).
- MD Simulations : Assess stability of ligand-receptor complexes over 100-ns trajectories.
- Validation : Compare predicted binding affinities with experimental IC values from enzyme inhibition assays. Discrepancies may indicate solvation effects or conformational flexibility .
Eigenschaften
IUPAC Name |
piperidine-2,4-dione | |
---|---|---|
Source | PubChem | |
URL | https://pubchem.ncbi.nlm.nih.gov | |
Description | Data deposited in or computed by PubChem | |
InChI |
InChI=1S/C5H7NO2/c7-4-1-2-6-5(8)3-4/h1-3H2,(H,6,8) | |
Source | PubChem | |
URL | https://pubchem.ncbi.nlm.nih.gov | |
Description | Data deposited in or computed by PubChem | |
InChI Key |
RDNZDMDLRIQQAX-UHFFFAOYSA-N | |
Source | PubChem | |
URL | https://pubchem.ncbi.nlm.nih.gov | |
Description | Data deposited in or computed by PubChem | |
Canonical SMILES |
C1CNC(=O)CC1=O | |
Source | PubChem | |
URL | https://pubchem.ncbi.nlm.nih.gov | |
Description | Data deposited in or computed by PubChem | |
Molecular Formula |
C5H7NO2 | |
Source | PubChem | |
URL | https://pubchem.ncbi.nlm.nih.gov | |
Description | Data deposited in or computed by PubChem | |
DSSTOX Substance ID |
DTXSID30447085 | |
Record name | 2,4-Piperidinedione | |
Source | EPA DSSTox | |
URL | https://comptox.epa.gov/dashboard/DTXSID30447085 | |
Description | DSSTox provides a high quality public chemistry resource for supporting improved predictive toxicology. | |
Molecular Weight |
113.11 g/mol | |
Source | PubChem | |
URL | https://pubchem.ncbi.nlm.nih.gov | |
Description | Data deposited in or computed by PubChem | |
CAS No. |
50607-30-2 | |
Record name | 2,4-Piperidinedione | |
Source | EPA DSSTox | |
URL | https://comptox.epa.gov/dashboard/DTXSID30447085 | |
Description | DSSTox provides a high quality public chemistry resource for supporting improved predictive toxicology. | |
Record name | 2,4-Piperidinedione | |
Source | European Chemicals Agency (ECHA) | |
URL | https://echa.europa.eu/information-on-chemicals | |
Description | The European Chemicals Agency (ECHA) is an agency of the European Union which is the driving force among regulatory authorities in implementing the EU's groundbreaking chemicals legislation for the benefit of human health and the environment as well as for innovation and competitiveness. | |
Explanation | Use of the information, documents and data from the ECHA website is subject to the terms and conditions of this Legal Notice, and subject to other binding limitations provided for under applicable law, the information, documents and data made available on the ECHA website may be reproduced, distributed and/or used, totally or in part, for non-commercial purposes provided that ECHA is acknowledged as the source: "Source: European Chemicals Agency, http://echa.europa.eu/". Such acknowledgement must be included in each copy of the material. ECHA permits and encourages organisations and individuals to create links to the ECHA website under the following cumulative conditions: Links can only be made to webpages that provide a link to the Legal Notice page. | |
Retrosynthesis Analysis
AI-Powered Synthesis Planning: Our tool employs the Template_relevance Pistachio, Template_relevance Bkms_metabolic, Template_relevance Pistachio_ringbreaker, Template_relevance Reaxys, Template_relevance Reaxys_biocatalysis model, leveraging a vast database of chemical reactions to predict feasible synthetic routes.
One-Step Synthesis Focus: Specifically designed for one-step synthesis, it provides concise and direct routes for your target compounds, streamlining the synthesis process.
Accurate Predictions: Utilizing the extensive PISTACHIO, BKMS_METABOLIC, PISTACHIO_RINGBREAKER, REAXYS, REAXYS_BIOCATALYSIS database, our tool offers high-accuracy predictions, reflecting the latest in chemical research and data.
Strategy Settings
Precursor scoring | Relevance Heuristic |
---|---|
Min. plausibility | 0.01 |
Model | Template_relevance |
Template Set | Pistachio/Bkms_metabolic/Pistachio_ringbreaker/Reaxys/Reaxys_biocatalysis |
Top-N result to add to graph | 6 |
Feasible Synthetic Routes
Haftungsausschluss und Informationen zu In-Vitro-Forschungsprodukten
Bitte beachten Sie, dass alle Artikel und Produktinformationen, die auf BenchChem präsentiert werden, ausschließlich zu Informationszwecken bestimmt sind. Die auf BenchChem zum Kauf angebotenen Produkte sind speziell für In-vitro-Studien konzipiert, die außerhalb lebender Organismen durchgeführt werden. In-vitro-Studien, abgeleitet von dem lateinischen Begriff "in Glas", beinhalten Experimente, die in kontrollierten Laborumgebungen unter Verwendung von Zellen oder Geweben durchgeführt werden. Es ist wichtig zu beachten, dass diese Produkte nicht als Arzneimittel oder Medikamente eingestuft sind und keine Zulassung der FDA für die Vorbeugung, Behandlung oder Heilung von medizinischen Zuständen, Beschwerden oder Krankheiten erhalten haben. Wir müssen betonen, dass jede Form der körperlichen Einführung dieser Produkte in Menschen oder Tiere gesetzlich strikt untersagt ist. Es ist unerlässlich, sich an diese Richtlinien zu halten, um die Einhaltung rechtlicher und ethischer Standards in Forschung und Experiment zu gewährleisten.