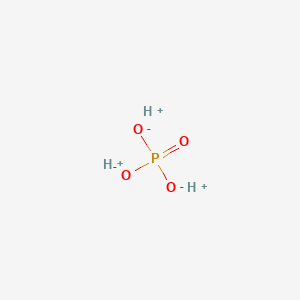
Hydron;phosphate
Übersicht
Beschreibung
Phosphoric acid, also known as phosphate or [po(OH)3], belongs to the class of inorganic compounds known as non-metal phosphates. These are inorganic non-metallic compounds containing a phosphate as its largest oxoanion. Phosphoric acid has been detected in multiple biofluids, such as feces, urine, and blood. Within the cell, phosphoric acid is primarily located in the cytoplasm. Phosphoric acid exists in all eukaryotes, ranging from yeast to humans. In humans, phosphoric acid is involved in the glutamate metabolism pathway, the glutathione metabolism pathway, the glycerol phosphate shuttle pathway, and the purine metabolism pathway. Phosphoric acid is also involved in several metabolic disorders, some of which include the hypophosphatasia pathway, the leigh syndrome pathway, creatine deficiency, guanidinoacetate methyltransferase deficiency, and L-arginine:glycine amidinotransferase deficiency. Phosphoric acid is a potentially toxic compound.
Wissenschaftliche Forschungsanwendungen
Raman Spectroscopy in Hydrolysis Studies Hydron phosphate's hydrolysis was monitored using Raman spectroscopy. This study identified that hydrolysis predominantly occurs at terminal phosphate units. It's a zeroth-order reaction, obeying the Arrhenius equation (H. D. de Jager & A. Heyns, 1998).
Phosphorus Reclamation from Animal Manures Hydrothermal carbonization of animal manures is an innovative method for reclaiming phosphate. This process captures and recycles phosphate, mitigating environmental impacts of phosphate pollution (S. Heilmann et al., 2014).
Use in Sunscreens Hydroxyapatite doped with elements like Zn2+, Fe3+, and Cr3+ demonstrates potential for use in sunscreens. The biocompatible phosphate materials, when doped, may have protective effects against UV rays (T. Araujo et al., 2010).
Biological Phosphate Removal Processes Biological phosphate removal is a key process in wastewater treatment. Understanding the microbiological and engineering aspects of this process is crucial for efficient operation and design (M. V. van Loosdrecht et al., 1997).
Sensor Arrays for Phosphates Detection Metal-organic-framework (MOF) nanozyme-based sensor arrays have been developed for discriminating and probing phosphates. These sensors are useful in detecting phosphates involved in enzymatic hydrolysis (Li Qin et al., 2018).
Agricultural Applications Synthetic apatite nanoparticles, a form of phosphate, have shown potential as an effective phosphorus fertilizer for crops like soybean, enhancing growth rate and yield while reducing environmental risks (Ruiqiang Liu & R. Lal, 2014).
Bone Cell Attachment and Differentiation Phosphate group incorporation in hydrogels has been investigated for its potential in bone cell attachment and differentiation, showing promising results in bone regeneration applications (M. Dadsetan et al., 2012).
Oral Care Products Hydroxyapatite, a calcium phosphate, is used in dentistry and oral care products for tooth remineralization, sensitivity reduction, and biofilm control (Lijie Chen et al., 2021).
Geochemical Behavior Studies The study of polyphosphate adsorption and hydrolysis on aluminum oxides is essential for understanding phosphate's geochemical behaviors and environmental impacts (B. Wan et al., 2019).
Phosphate Crisis and Sustainability Investigating the depletion of phosphate reserves and finding sustainable solutions is crucial for future generations. This includes understanding the role of phosphate in agriculture and the environment (P. Abelson, 1999).
Eigenschaften
IUPAC Name |
hydron;phosphate | |
---|---|---|
Details | Computed by LexiChem 2.6.6 (PubChem release 2019.06.18) | |
Source | PubChem | |
URL | https://pubchem.ncbi.nlm.nih.gov | |
Description | Data deposited in or computed by PubChem | |
InChI |
InChI=1S/H3O4P/c1-5(2,3)4/h(H3,1,2,3,4) | |
Details | Computed by InChI 1.0.5 (PubChem release 2019.06.18) | |
Source | PubChem | |
URL | https://pubchem.ncbi.nlm.nih.gov | |
Description | Data deposited in or computed by PubChem | |
InChI Key |
NBIIXXVUZAFLBC-UHFFFAOYSA-N | |
Details | Computed by InChI 1.0.5 (PubChem release 2019.06.18) | |
Source | PubChem | |
URL | https://pubchem.ncbi.nlm.nih.gov | |
Description | Data deposited in or computed by PubChem | |
Canonical SMILES |
[H+].[H+].[H+].[O-]P(=O)([O-])[O-] | |
Details | Computed by OEChem 2.1.5 (PubChem release 2019.06.18) | |
Source | PubChem | |
URL | https://pubchem.ncbi.nlm.nih.gov | |
Description | Data deposited in or computed by PubChem | |
Molecular Formula |
H3O4P | |
Details | Computed by PubChem 2.1 (PubChem release 2019.06.18) | |
Source | PubChem | |
URL | https://pubchem.ncbi.nlm.nih.gov | |
Description | Data deposited in or computed by PubChem | |
Molecular Weight |
97.995 g/mol | |
Details | Computed by PubChem 2.1 (PubChem release 2021.05.07) | |
Source | PubChem | |
URL | https://pubchem.ncbi.nlm.nih.gov | |
Description | Data deposited in or computed by PubChem | |
Retrosynthesis Analysis
AI-Powered Synthesis Planning: Our tool employs the Template_relevance Pistachio, Template_relevance Bkms_metabolic, Template_relevance Pistachio_ringbreaker, Template_relevance Reaxys, Template_relevance Reaxys_biocatalysis model, leveraging a vast database of chemical reactions to predict feasible synthetic routes.
One-Step Synthesis Focus: Specifically designed for one-step synthesis, it provides concise and direct routes for your target compounds, streamlining the synthesis process.
Accurate Predictions: Utilizing the extensive PISTACHIO, BKMS_METABOLIC, PISTACHIO_RINGBREAKER, REAXYS, REAXYS_BIOCATALYSIS database, our tool offers high-accuracy predictions, reflecting the latest in chemical research and data.
Strategy Settings
Precursor scoring | Relevance Heuristic |
---|---|
Min. plausibility | 0.01 |
Model | Template_relevance |
Template Set | Pistachio/Bkms_metabolic/Pistachio_ringbreaker/Reaxys/Reaxys_biocatalysis |
Top-N result to add to graph | 6 |
Feasible Synthetic Routes
Haftungsausschluss und Informationen zu In-Vitro-Forschungsprodukten
Bitte beachten Sie, dass alle Artikel und Produktinformationen, die auf BenchChem präsentiert werden, ausschließlich zu Informationszwecken bestimmt sind. Die auf BenchChem zum Kauf angebotenen Produkte sind speziell für In-vitro-Studien konzipiert, die außerhalb lebender Organismen durchgeführt werden. In-vitro-Studien, abgeleitet von dem lateinischen Begriff "in Glas", beinhalten Experimente, die in kontrollierten Laborumgebungen unter Verwendung von Zellen oder Geweben durchgeführt werden. Es ist wichtig zu beachten, dass diese Produkte nicht als Arzneimittel oder Medikamente eingestuft sind und keine Zulassung der FDA für die Vorbeugung, Behandlung oder Heilung von medizinischen Zuständen, Beschwerden oder Krankheiten erhalten haben. Wir müssen betonen, dass jede Form der körperlichen Einführung dieser Produkte in Menschen oder Tiere gesetzlich strikt untersagt ist. Es ist unerlässlich, sich an diese Richtlinien zu halten, um die Einhaltung rechtlicher und ethischer Standards in Forschung und Experiment zu gewährleisten.