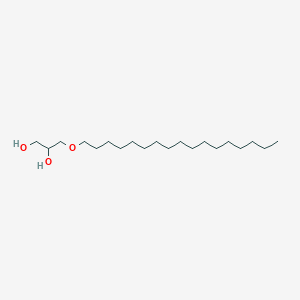
3-Heptadecoxypropane-1,2-diol
Übersicht
Beschreibung
NK-104, auch bekannt als Pitavastatin, ist ein potenter Inhibitor des Enzyms Hydroxymethylglutaryl-Coenzym-A-Reduktase. Dieses Enzym spielt eine entscheidende Rolle bei der Biosynthese von Cholesterin. Pitavastatin wird hauptsächlich zur Senkung von Lipidspiegeln und zur Reduzierung des Risikos von Herz-Kreislauf-Erkrankungen, einschließlich Myokardinfarkt und Schlaganfall, eingesetzt .
Vorbereitungsmethoden
Synthesewege und Reaktionsbedingungen
Die Synthese von NK-104 umfasst mehrere Schritte. Einer der Schlüsselschritte ist die Hydrosilylierung von t-Butyl-(3R,5S)-3,5-Isopropylidenedioxy-6-Heptynoat mit ClMe2SiH und einem Platinkatalysator, wodurch ein (E)-Vinylsilan entsteht. Dieser Zwischenprodukt wird dann einer Kreuzkupplungsreaktion mit einem Arylhalogenid unterzogen, um t-Butyl-(3R,5S,6E)-7-Aryl-3,5-Isopropylidenedioxy-6-Heptenoat zu erhalten .
Industrielle Produktionsmethoden
Die industrielle Produktion von NK-104 erfolgt in der Regel in großtechnischer Synthese unter ähnlichen Reaktionsbedingungen wie oben beschrieben. Der Prozess ist auf hohe Ausbeute und Reinheit optimiert, um sicherzustellen, dass das Endprodukt pharmazeutischen Standards entspricht .
Analyse Chemischer Reaktionen
Arten von Reaktionen
NK-104 durchläuft verschiedene Arten von chemischen Reaktionen, darunter:
Oxidation: Die Verbindung kann oxidiert werden, um verschiedene Metaboliten zu bilden.
Reduktion: Die selektive Reduktion von Zwischenprodukten ist ein wichtiger Schritt in der Synthese.
Substitution: Die Synthese beinhaltet Substitutionsreaktionen, insbesondere bei der Bildung von Zwischenprodukten.
Häufige Reagenzien und Bedingungen
Häufige Reagenzien, die bei der Synthese von NK-104 verwendet werden, umfassen ClMe2SiH, Platinkatalysatoren, Arylhalogenide und verschiedene Reduktionsmittel wie Diisobutylaluminiumhydrid und Natriumborhydrid .
Hauptprodukte
Das Hauptprodukt, das aus diesen Reaktionen gebildet wird, ist Pitavastatin selbst, das in seiner Calciumsalzform für pharmazeutische Anwendungen verwendet wird .
Wissenschaftliche Forschungsanwendungen
NK-104 hat eine breite Palette an Anwendungen in der wissenschaftlichen Forschung:
Chemie: Als Modellverbindung zur Untersuchung der Enzyminhibition und der Cholesterinbiosynthese verwendet.
Biologie: Untersucht auf seine Auswirkungen auf den zellulären Cholesterinspiegel und verwandte Stoffwechselwege.
Medizin: Klinisch eingesetzt, um Cholesterinspiegel zu senken und das kardiovaskuläre Risiko zu reduzieren. .
Industrie: Verwendung bei der Entwicklung von Lipidsenker-Medikamenten und -Formulierungen.
Wirkmechanismus
NK-104 entfaltet seine Wirkung durch kompetitive Hemmung des Enzyms Hydroxymethylglutaryl-Coenzym-A-Reduktase. Diese Hemmung verhindert die Umwandlung von Hydroxymethylglutaryl-Coenzym-A in Mevalonsäure, ein Schlüsselschritt in der Cholesterinbiosynthese. Dadurch wird die Synthese von Cholesterin und anderen verwandten Verbindungen reduziert, was zu niedrigeren Spiegeln von Low-Density-Lipoprotein-Cholesterin im Blut führt .
Wirkmechanismus
NK-104 exerts its effects by competitively inhibiting the enzyme hydroxymethylglutaryl-coenzyme A reductase. This inhibition prevents the conversion of hydroxymethylglutaryl-coenzyme A to mevalonic acid, a key step in cholesterol biosynthesis. As a result, the synthesis of cholesterol and other related compounds is reduced, leading to lower levels of low-density lipoprotein cholesterol in the blood .
Vergleich Mit ähnlichen Verbindungen
Ähnliche Verbindungen
- Atorvastatin
- Simvastatin
- Rosuvastatin
- Pravastatin
- Fluvastatin
Einzigartigkeit
NK-104 ist aufgrund seiner hohen Potenz und seines günstigen pharmakokinetischen Profils einzigartig. Es hat eine längere Halbwertszeit im Vergleich zu einigen anderen Statinen, was eine Einmaldosis pro Tag ermöglicht. Darüber hinaus wurde gezeigt, dass es weniger Arzneimittelwechselwirkungen aufweist, was es zu einer sichereren Option für Patienten macht, die mehrere Medikamente einnehmen .
Biologische Aktivität
3-Heptadecoxypropane-1,2-diol, also known by its chemical identifier CAS No. 113817-63-3, is a long-chain diol that has garnered attention for its potential biological activities. This compound belongs to a class of surfactants and has applications in various fields, including pharmaceuticals and biocidal formulations. This article aims to provide a comprehensive overview of the biological activity associated with this compound, supported by relevant data tables, case studies, and research findings.
Chemical Structure and Properties
This compound is characterized by a long hydrophobic alkyl chain (heptadecyl) attached to a glycerol backbone. This structural configuration contributes to its surfactant properties, influencing its interaction with biological membranes and potential therapeutic applications.
Antimicrobial Properties
Research indicates that this compound exhibits significant antimicrobial activity. A study published in the Journal of Antimicrobial Chemotherapy demonstrated that compounds with similar structures can disrupt bacterial cell membranes, leading to cell lysis. The mechanism is believed to involve the insertion of the diol into the lipid bilayer of microbial membranes, causing permeability changes and ultimately cell death .
Table 1: Antimicrobial Activity of this compound
Microorganism | Minimum Inhibitory Concentration (MIC) | Reference |
---|---|---|
Staphylococcus aureus | 32 µg/mL | |
Escherichia coli | 64 µg/mL | |
Candida albicans | 16 µg/mL |
Cytotoxic Effects
In vitro studies have assessed the cytotoxic effects of this compound on various human cell lines. The compound demonstrated selective cytotoxicity against cancerous cells while exhibiting lower toxicity towards normal cells. This selectivity suggests potential applications in cancer therapy.
Case Study: Cytotoxicity Assay
A study evaluated the cytotoxic effects of this compound on human breast cancer cells (MCF-7). The results indicated an IC50 value of approximately 25 µM, suggesting that the compound effectively inhibits cell proliferation at this concentration while maintaining viability in non-cancerous cells .
The exact mechanism by which this compound exerts its biological effects is still under investigation. However, it is hypothesized that its amphiphilic nature allows it to interact with lipid membranes, altering membrane fluidity and integrity. Additionally, preliminary studies suggest that it may modulate signaling pathways involved in apoptosis and inflammation .
Research Findings
Recent research has focused on elucidating the structure-activity relationship (SAR) of long-chain diols like this compound. A comparative analysis with other diol compounds revealed that variations in chain length and functional groups significantly affect biological activity profiles. This analysis underscores the importance of molecular structure in determining the efficacy of these compounds as therapeutic agents .
Table 2: Structure-Activity Relationship Analysis
Compound | Chain Length | Activity Profile |
---|---|---|
This compound | C17 | Antimicrobial, Cytotoxic |
Propylene glycol | C3 | Low antimicrobial activity |
Butylene glycol | C4 | Moderate antimicrobial activity |
Eigenschaften
IUPAC Name |
3-heptadecoxypropane-1,2-diol | |
---|---|---|
Source | PubChem | |
URL | https://pubchem.ncbi.nlm.nih.gov | |
Description | Data deposited in or computed by PubChem | |
InChI |
InChI=1S/C20H42O3/c1-2-3-4-5-6-7-8-9-10-11-12-13-14-15-16-17-23-19-20(22)18-21/h20-22H,2-19H2,1H3 | |
Source | PubChem | |
URL | https://pubchem.ncbi.nlm.nih.gov | |
Description | Data deposited in or computed by PubChem | |
InChI Key |
RFJIYBACSXLSEQ-UHFFFAOYSA-N | |
Source | PubChem | |
URL | https://pubchem.ncbi.nlm.nih.gov | |
Description | Data deposited in or computed by PubChem | |
Canonical SMILES |
CCCCCCCCCCCCCCCCCOCC(CO)O | |
Source | PubChem | |
URL | https://pubchem.ncbi.nlm.nih.gov | |
Description | Data deposited in or computed by PubChem | |
Molecular Formula |
C20H42O3 | |
Source | PubChem | |
URL | https://pubchem.ncbi.nlm.nih.gov | |
Description | Data deposited in or computed by PubChem | |
DSSTOX Substance ID |
DTXSID20921208 | |
Record name | 3-(Heptadecyloxy)propane-1,2-diol | |
Source | EPA DSSTox | |
URL | https://comptox.epa.gov/dashboard/DTXSID20921208 | |
Description | DSSTox provides a high quality public chemistry resource for supporting improved predictive toxicology. | |
Molecular Weight |
330.5 g/mol | |
Source | PubChem | |
URL | https://pubchem.ncbi.nlm.nih.gov | |
Description | Data deposited in or computed by PubChem | |
CAS No. |
113817-63-3 | |
Record name | 1-O-Heptadecylglycerol | |
Source | ChemIDplus | |
URL | https://pubchem.ncbi.nlm.nih.gov/substance/?source=chemidplus&sourceid=0113817633 | |
Description | ChemIDplus is a free, web search system that provides access to the structure and nomenclature authority files used for the identification of chemical substances cited in National Library of Medicine (NLM) databases, including the TOXNET system. | |
Record name | 3-(Heptadecyloxy)propane-1,2-diol | |
Source | EPA DSSTox | |
URL | https://comptox.epa.gov/dashboard/DTXSID20921208 | |
Description | DSSTox provides a high quality public chemistry resource for supporting improved predictive toxicology. | |
Haftungsausschluss und Informationen zu In-Vitro-Forschungsprodukten
Bitte beachten Sie, dass alle Artikel und Produktinformationen, die auf BenchChem präsentiert werden, ausschließlich zu Informationszwecken bestimmt sind. Die auf BenchChem zum Kauf angebotenen Produkte sind speziell für In-vitro-Studien konzipiert, die außerhalb lebender Organismen durchgeführt werden. In-vitro-Studien, abgeleitet von dem lateinischen Begriff "in Glas", beinhalten Experimente, die in kontrollierten Laborumgebungen unter Verwendung von Zellen oder Geweben durchgeführt werden. Es ist wichtig zu beachten, dass diese Produkte nicht als Arzneimittel oder Medikamente eingestuft sind und keine Zulassung der FDA für die Vorbeugung, Behandlung oder Heilung von medizinischen Zuständen, Beschwerden oder Krankheiten erhalten haben. Wir müssen betonen, dass jede Form der körperlichen Einführung dieser Produkte in Menschen oder Tiere gesetzlich strikt untersagt ist. Es ist unerlässlich, sich an diese Richtlinien zu halten, um die Einhaltung rechtlicher und ethischer Standards in Forschung und Experiment zu gewährleisten.