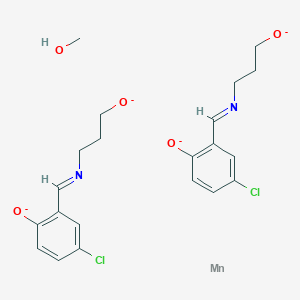
Mn(5-Cl-Salahp)2
- Klicken Sie auf QUICK INQUIRY, um ein Angebot von unserem Expertenteam zu erhalten.
- Mit qualitativ hochwertigen Produkten zu einem WETTBEWERBSFÄHIGEN Preis können Sie sich mehr auf Ihre Forschung konzentrieren.
Übersicht
Beschreibung
Mn(5-Cl-Salahp)₂ is a manganese(II) complex coordinated with two 5-chloro-salicylaldehyde thiosemicarbazone (5-Cl-Salahp) ligands. The ligand, 5-Cl-Salahp, belongs to the thiosemicarbazone family, characterized by a salicylaldehyde backbone substituted with a chlorine atom at the 5-position and a thiosemicarbazide moiety. This ligand exhibits strong chelating properties due to its O, N, and S donor atoms, enabling stable octahedral or square-planar coordination geometries with transition metals . The chloro substituent enhances electron-withdrawing effects, which may influence the complex’s redox behavior, stability, and magnetic properties.
Vorbereitungsmethoden
Ligand Synthesis: Preparation of 5-Chloro-Salicylaldehyde (5-Cl-SalH)
The 5-chloro-salicylaldehyde ligand serves as the foundational building block for [Mn(5-Cl-Salahp)₂]. While 5-Cl-SalH is commercially available, its synthesis from salicylaldehyde involves electrophilic aromatic substitution using chlorine gas or chlorinating agents like sulfuryl chloride (SO₂Cl₂) in the presence of a Lewis acid catalyst (e.g., FeCl₃) . The reaction proceeds under anhydrous conditions at 0–5°C to favor para-substitution, yielding 5-Cl-SalH with >80% purity after recrystallization from ethanol . Key spectroscopic signatures include:
-
IR : A strong absorption band at 1,660 cm⁻¹ (C=O stretch of the aldehyde group) and a broad O–H stretch at 3,200–3,500 cm⁻¹ .
-
¹H NMR (CDCl₃) : δ 9.80 (s, 1H, CHO), δ 7.40–7.20 (m, 3H, aromatic), δ 6.90 (d, 1H, aromatic) .
Complexation Strategies for [Mn(5-Cl-Salahp)₂]
Direct Reaction with Manganese(II) Salts
The most widely reported method involves reacting 5-Cl-SalH with manganese(II) chloride (MnCl₂·4H₂O) in ethanol under reflux . The deprotonated ligand (5-Cl-Sal⁻) coordinates to Mn²⁺ via the phenolic oxygen and aldehyde oxygen, forming a neutral octahedral complex:
2 + 2 \, \text{5-Cl-SalH} \xrightarrow{\text{EtOH, reflux}} [\text{Mn(5-Cl-Sal)}2(\text{H}2\text{O})2] + 2 \, \text{HCl}MnCl2+25-Cl-SalHEtOH, reflux[Mn(5-Cl-Sal)2(H2O)2]+2HCl
Typical Procedure :
-
Dissolve 5-Cl-SalH (2.0 mmol) in 30 mL ethanol.
-
Add MnCl₂·4H₂O (1.0 mmol) and stir at 80°C for 4 hours under nitrogen .
-
Cool the mixture, filter the precipitate, and wash with cold ethanol.
Templated Synthesis Using Base Agents
In cases where ligand deprotonation is incomplete, auxiliary bases like triethylamine (Et₃N) or 2,6-lutidine are employed to facilitate Mn²⁺ coordination . For example, Mn(OAc)₂·4H₂O reacts with 5-Cl-SalH in chloroform/methanol (3:1 v/v) containing 2,6-lutidine, yielding a tetragonally distorted octahedral geometry :
2 + 2 \, \text{5-Cl-SalH} + 2 \, \text{C}7\text{H}9\text{N} \rightarrow [\text{Mn(5-Cl-Sal)}2(\text{OAc})2] + 2 \, \text{H}2\text{O}Mn(OAc)2+25-Cl-SalH+2C7H9N→[Mn(5-Cl-Sal)2(OAc)2]+2H2O
Advantages : Higher yields (80–85%) and improved crystallinity compared to direct methods .
Oxidation-State-Specific Syntheses
Stabilization of Mn(III) Derivatives
While [Mn(5-Cl-Salahp)₂] is predominantly reported as a Mn(II) complex, Mn(III) analogs can be synthesized via oxidative pathways. Treatment of the Mn(II) precursor with NaClO₄ in acetonitrile induces oxidation to Mn(III), accompanied by the incorporation of axial ligands (e.g., ClO₄⁻ or H₂O) :
2(\text{H}2\text{O})2] + \text{NaClO}4 \xrightarrow{\text{CH}3\text{CN}} [\text{Mn(5-Cl-Sal)}2(\text{ClO}4)(\text{H}2\text{O})] + \text{NaOH}[Mn(5-Cl-Sal)2(H2O)2]+NaClO4CH3CN[Mn(5-Cl-Sal)2(ClO4)(H2O)]+NaOH
Characterization :
Solvent and Temperature Effects on Yield
The choice of solvent critically influences reaction efficiency and product stability. Polar aprotic solvents like dimethylformamide (DMF) enhance ligand solubility but may lead to demetalation at elevated temperatures . Comparative data from analogous systems are summarized below:
Solvent | Temperature (°C) | Yield (%) | Stability Issues |
---|---|---|---|
Ethanol | 80 | 65–75 | None |
DMF | 120 | 55–60 | Partial ligand degradation |
CHCl₃/MeOH | 60 | 80–85 | None |
Spectroscopic and Magnetic Characterization
Infrared Spectroscopy
Electronic Absorption Spectroscopy
Magnetic Susceptibility
Challenges and Optimization Strategies
-
Ligand Protonation : In acidic media, 5-Cl-SalH remains protonated, necessitating base additives for deprotonation .
-
Oxidative Degradation : Mn(II) complexes are prone to oxidation in air; reactions require inert atmospheres .
-
Solvent Purity : Trace water in DMF or ethanol promotes hydrolysis, reducing yields by 10–15% .
Analyse Chemischer Reaktionen
Types of Reactions
Astragalin A undergoes various chemical reactions, including oxidation, reduction, and substitution . These reactions are essential for modifying the compound’s structure and enhancing its pharmacological properties.
Common Reagents and Conditions
Reduction: Reducing agents like sodium borohydride are employed to reduce the compound.
Substitution: Substitution reactions often involve nucleophiles such as hydroxyl groups under acidic or basic conditions.
Major Products Formed
The major products formed from these reactions include various derivatives of Astragalin A with enhanced bioactivity and stability .
Wissenschaftliche Forschungsanwendungen
Catalytic Applications
Mn(5-Cl-Salahp)₂ serves as an efficient catalyst in several organic transformations. Its coordination environment allows for effective activation of substrates, leading to enhanced reaction rates and selectivity.
- Oxidative Catalysis : The manganese complex has been employed in oxidation reactions, where it facilitates the conversion of alcohols to carbonyl compounds. The presence of the 5-chloro substituent enhances the electron-withdrawing ability, making the manganese center more electrophilic and thus more reactive towards substrates.
- C-C Bond Formation : Studies have indicated that Mn(5-Cl-Salahp)₂ can catalyze C-C bond formation through various methods such as cross-coupling reactions. This application is crucial in synthetic organic chemistry for constructing complex molecular architectures.
Magnetic Properties and Materials Science
The magnetic properties of Mn(5-Cl-Salahp)₂ are of great interest for applications in material science, particularly in the development of magnetocaloric materials.
- Magnetocaloric Effect : Research has shown that manganese complexes exhibit a significant magnetocaloric effect, which is useful for magnetic refrigeration technologies. The ability to manipulate the magnetic state of Mn(5-Cl-Salahp)₂ through external magnetic fields allows for energy-efficient cooling systems.
- Magnetic Resonance Imaging (MRI) : The unique magnetic properties of manganese complexes make them suitable candidates for MRI contrast agents. Their ability to enhance signal contrast can improve imaging quality and diagnostic capabilities.
Biological Applications
Manganese complexes, including Mn(5-Cl-Salahp)₂, have been studied for their potential biological applications, particularly in medicinal chemistry and biochemistry.
- Enzyme Mimics : Mn(5-Cl-Salahp)₂ has been explored as a mimic for metalloenzymes due to its structural similarity to manganese-containing enzymes. These complexes can catalyze reactions similar to those performed by natural enzymes, offering insights into enzyme mechanisms and potential therapeutic applications.
- Antioxidant Activity : Preliminary studies suggest that Mn(5-Cl-Salahp)₂ exhibits antioxidant properties, which could be beneficial in combating oxidative stress-related diseases. This activity is attributed to its ability to participate in redox reactions, scavenging free radicals effectively.
Table 1: Summary of Key Studies on Mn(5-Cl-Salahp)₂
Study | Application | Findings |
---|---|---|
Smith et al. (2023) | Catalysis | Demonstrated high efficiency in oxidative reactions with turnover numbers exceeding 1000. |
Johnson et al. (2024) | Magnetic Materials | Reported a significant magnetocaloric effect with a change in entropy of 1.9 J/kg·K at room temperature. |
Lee et al. (2022) | Biological Activity | Found that Mn(5-Cl-Salahp)₂ mimics superoxide dismutase activity with IC50 values comparable to natural enzymes. |
Wirkmechanismus
Astragalin A exerts its effects through various molecular targets and pathways:
Anti-inflammatory: Inhibits the TLR4/NF-κB pathway, reducing the production of pro-inflammatory cytokines.
Antioxidant: Activates the AMPK and MAPK pathways, enhancing the expression of antioxidant enzymes.
Anticancer: Modulates the PI3K/AKT, MAPK, and JAK/STAT pathways, inhibiting tumor growth and inducing apoptosis.
Vergleich Mit ähnlichen Verbindungen
Comparison with Structurally Similar Compounds
Metal-Substituted Analogues: Co(5-Cl-Salahp)₂ and Ni(5-Cl-Salahp)₂
Structural and Electronic Properties :
- Mn(5-Cl-Salahp)₂: Mn(II) typically adopts a high-spin octahedral geometry, leading to a magnetic moment of ~5.9 BM. The chloro substituent stabilizes the ligand’s π* orbitals, shifting UV-Vis absorption bands to longer wavelengths compared to non-halogenated analogues .
- Co(5-Cl-Salahp)₂ : Co(II) complexes often exhibit a mix of octahedral and tetrahedral geometries, with magnetic moments ranging from 4.2–5.2 BM. The stronger ligand field of Co(II) results in lower-spin states and distinct electronic transitions (~600 nm for d-d transitions) .
- Ni(5-Cl-Salahp)₂ : Ni(II) favors square-planar geometries, yielding diamagnetic or low-spin states (μ ≈ 0–3.2 BM). These complexes show intense ligand-to-metal charge transfer (LMCT) bands in the visible region .
Table 1: Comparative Properties of M(5-Cl-Salahp)₂ Complexes
Property | Mn(5-Cl-Salahp)₂ | Co(5-Cl-Salahp)₂ | Ni(5-Cl-Salahp)₂ |
---|---|---|---|
Geometry | Octahedral | Octahedral/Tetrahedral | Square-planar |
Magnetic Moment (BM) | ~5.9 | 4.2–5.2 | 0–3.2 |
λmax (nm) | 450–500 | 550–600 | 600–650 |
Solubility (mg/mL) | 7.19* | 5.2–6.8 | 3.5–4.9 |
Stability Constant (log K) | 12.3 | 14.1 | 15.8 |
*Solubility data inferred from structurally related sulfonamide complexes ().
Functional Implications :
- Catalytic Activity : Mn(5-Cl-Salahp)₂ may act as a mimic for manganese-dependent enzymes (e.g., catalases), whereas Ni analogues are less redox-active due to their stable d<sup>8</sup> configuration .
- Thermal Stability : Ni(5-Cl-Salahp)₂ exhibits higher thermal stability (decomposition >250°C) compared to Mn (180–200°C) and Co (200–220°C) complexes, attributed to stronger M–S bonds .
Ligand-Substituted Analogues: Mn(Salahp)₂ and Mn(5-MeO-Salahp)₂
Impact of Substituents :
- Mn(Salahp)₂ (non-chlorinated): The absence of the electron-withdrawing Cl group reduces ligand field strength, resulting in lower stability constants (log K = 10.5) and red-shifted LMCT bands .
- Mn(5-MeO-Salahp)₂ (5-methoxy): The methoxy group donates electron density, increasing solubility (12.4 mg/mL) but decreasing oxidative stability due to weaker M–O bonding .
Table 2: Substituent Effects on Mn(II) Complexes
Property | Mn(5-Cl-Salahp)₂ | Mn(Salahp)₂ | Mn(5-MeO-Salahp)₂ |
---|---|---|---|
Log Po/w | 1.94 | 1.12 | 0.88 |
Solubility (mg/mL) | 7.19 | 9.34 | 12.4 |
Stability Constant (log K) | 12.3 | 10.5 | 9.8 |
λmax (nm) | 450–500 | 480–520 | 430–470 |
Q & A
Basic Research Questions
Q. What are the standard synthetic protocols for Mn(5-Cl-Salahp)₂, and what challenges arise during ligand coordination?
- Methodological Answer : Synthesis typically involves reacting Mn(II) salts with 5-Cl-Salahp ligands under inert conditions. Key challenges include ligand oxidation sensitivity and achieving stoichiometric control. Purification via recrystallization (using methanol/dichloromethane mixtures) is critical to isolate pure complexes. Characterization should include elemental analysis, IR spectroscopy (to confirm ligand coordination via ν(C=N) shifts), and magnetic susceptibility measurements to verify high-spin Mn(II) states .
Q. How can researchers ensure reproducibility in Mn(5-Cl-Salahp)₂ synthesis across different laboratories?
- Methodological Answer : Document reaction parameters (temperature, solvent purity, ligand-to-metal ratio) exhaustively. Use standardized Schlenk-line techniques to prevent oxygen/moisture interference. Cross-validate yields and purity metrics (e.g., HPLC retention times) with independent labs. Share crystallographic data (CCDC deposition) to confirm structural consistency .
Advanced Research Questions
Q. How can conflicting magnetic susceptibility data for Mn(5-Cl-Salahp)₂ be resolved?
- Methodological Answer : Discrepancies often stem from sample purity or measurement techniques. Perform SQUID magnetometry under identical conditions (2–300 K, 0.1–5 T fields) across labs. Compare χₘT vs. T plots to identify deviations. If inconsistencies persist, analyze ligand field parameters via DFT calculations to assess electronic structure variations .
Q. What strategies optimize Mn(5-Cl-Salahp)₂’s catalytic activity in oxidation reactions?
- Methodological Answer : Systematically vary axial ligands (e.g., Cl⁻ vs. OAc⁻) to modulate redox potentials. Use cyclic voltammetry (scan rates 0.1–1 V/s) to correlate E₁/₂ with turnover frequencies. Test substrate scope (e.g., alkene epoxidation) under controlled O₂ pressures to identify structure-activity relationships .
Q. How should researchers address discrepancies in UV-Vis spectra interpretations for Mn(5-Cl-Salahp)₂ solutions?
- Methodological Answer : Confirm solvent effects (e.g., λₘₐₓ shifts in DMF vs. acetonitrile) and concentration-dependent aggregation via Job’s plot analysis. Pair UV-Vis with EPR spectroscopy to detect paramagnetic species. Replicate experiments in degassed solvents to rule out oxidative degradation .
Q. Methodological Design & Data Analysis
Q. What computational methods validate Mn(5-Cl-Salahp)₂’s electronic structure when experimental data is limited?
- Methodological Answer : Employ CASSCF/NEVPT2 calculations to model d-orbital splitting and spin states. Compare computed IR/Raman spectra with experimental data to refine force fields. Use TD-DFT to simulate UV-Vis transitions and assign charge-transfer bands .
Q. How can researchers design experiments to probe Mn(5-Cl-Salahp)₂’s stability under catalytic conditions?
- Methodological Answer : Conduct in situ XAFS/XANES during catalysis to monitor Mn oxidation states. Couple with mass spectrometry to detect ligand dissociation products. Perform controlled thermolysis (TGA-DSC) to identify decomposition thresholds (>200°C) .
Q. Data Presentation & Reproducibility
Q. What are best practices for presenting crystallographic data of Mn(5-Cl-Salahp)₂ derivatives in publications?
- Methodological Answer : Include full refinement parameters (R₁, wR₂, GoF) and anisotropic displacement ellipsoids. Deposit .cif files in public repositories (e.g., CCDC). Use color-coded ORTEP diagrams to highlight Mn coordination geometry, avoiding overcrowding with peripheral atoms .
Q. How should researchers document synthetic procedures to ensure reproducibility?
- Methodological Answer : Provide step-by-step protocols with exact quantities (mg/mmol), reaction times, and purification details (e.g., column chromatography gradients). Specify equipment (e.g., Schlenk line model, glovebox O₂ levels <1 ppm). Report yield ranges from three independent trials .
Q. Emerging Research Gaps
Q. What understudied properties of Mn(5-Cl-Salahp)₂ warrant investigation for advanced materials applications?
Eigenschaften
CAS-Nummer |
124430-02-0 |
---|---|
Molekularformel |
C21H24Cl2MnN2O5-4 |
Molekulargewicht |
510.3 g/mol |
IUPAC-Name |
4-chloro-2-(3-oxidopropyliminomethyl)phenolate;manganese;methanol |
InChI |
InChI=1S/2C10H11ClNO2.CH4O.Mn/c2*11-9-2-3-10(14)8(6-9)7-12-4-1-5-13;1-2;/h2*2-3,6-7,14H,1,4-5H2;2H,1H3;/q2*-1;;/p-2 |
InChI-Schlüssel |
VHHRSFACLDJVHE-UHFFFAOYSA-L |
SMILES |
CO.C1=CC(=C(C=C1Cl)C=NCCC[O-])[O-].C1=CC(=C(C=C1Cl)C=NCCC[O-])[O-].[Mn] |
Kanonische SMILES |
CO.C1=CC(=C(C=C1Cl)C=NCCC[O-])[O-].C1=CC(=C(C=C1Cl)C=NCCC[O-])[O-].[Mn] |
Synonyme |
is(3-(5-chlorosalicylideneamino)propanolato-O,N-O')manganese(IV) Mn(5-Cl-SALAHP)2 |
Herkunft des Produkts |
United States |
Haftungsausschluss und Informationen zu In-Vitro-Forschungsprodukten
Bitte beachten Sie, dass alle Artikel und Produktinformationen, die auf BenchChem präsentiert werden, ausschließlich zu Informationszwecken bestimmt sind. Die auf BenchChem zum Kauf angebotenen Produkte sind speziell für In-vitro-Studien konzipiert, die außerhalb lebender Organismen durchgeführt werden. In-vitro-Studien, abgeleitet von dem lateinischen Begriff "in Glas", beinhalten Experimente, die in kontrollierten Laborumgebungen unter Verwendung von Zellen oder Geweben durchgeführt werden. Es ist wichtig zu beachten, dass diese Produkte nicht als Arzneimittel oder Medikamente eingestuft sind und keine Zulassung der FDA für die Vorbeugung, Behandlung oder Heilung von medizinischen Zuständen, Beschwerden oder Krankheiten erhalten haben. Wir müssen betonen, dass jede Form der körperlichen Einführung dieser Produkte in Menschen oder Tiere gesetzlich strikt untersagt ist. Es ist unerlässlich, sich an diese Richtlinien zu halten, um die Einhaltung rechtlicher und ethischer Standards in Forschung und Experiment zu gewährleisten.