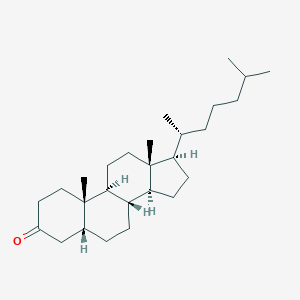
5beta-Cholestan-3-one
Übersicht
Beschreibung
Cholestan-3-one is a steroidal compound with the molecular formula C₂₇H₄₆O. It is a derivative of cholesterol, featuring a ketone group at the third carbon position. This compound is known for its role in various biochemical processes and its presence in certain metabolic pathways. Cholestan-3-one is also referred to as 3-oxocholestane or cholestanone.
Vorbereitungsmethoden
Synthetische Wege und Reaktionsbedingungen: Cholestan-3-on kann durch verschiedene Verfahren synthetisiert werden. Ein gängiger Ansatz beinhaltet die Oxidation von Cholesterin. Dieser Prozess verwendet typischerweise Reagenzien wie Chromtrioxid (CrO₃) in Essigsäure oder Pyridiniumchlorchromat (PCC) in Dichlormethan. Eine andere Methode beinhaltet die Reduktion von Cholest-4-en-3-on unter Verwendung von Zink und Salzsäure in Essigsäureanhydrid, wodurch Cholestan-3-on als eines der Produkte entsteht .
Industrielle Herstellungsverfahren: In industriellen Umgebungen wird Cholestan-3-on häufig durch die Oxidation von Cholesterin unter Verwendung skalierbarer und effizienterer Methoden hergestellt. Diese Methoden können die Verwendung katalytischer Systeme und optimierter Reaktionsbedingungen umfassen, um hohe Ausbeuten und Reinheit des Endprodukts zu gewährleisten.
Analyse Chemischer Reaktionen
Reaktionstypen: Cholestan-3-on unterliegt verschiedenen chemischen Reaktionen, darunter:
Oxidation: Cholestan-3-on kann weiter oxidiert werden, um Cholest-4-en-3-on zu erzeugen.
Reduktion: Es kann mit Reduktionsmitteln wie Natriumborhydrid (NaBH₄) oder Lithiumaluminiumhydrid (LiAlH₄) zu Cholestan-3-ol reduziert werden.
Substitution: Die Ketogruppe am dritten Kohlenstoff kann nukleophile Substitutionsreaktionen eingehen.
Gängige Reagenzien und Bedingungen:
Oxidation: Chromtrioxid (CrO₃) in Essigsäure oder Pyridiniumchlorchromat (PCC) in Dichlormethan.
Reduktion: Natriumborhydrid (NaBH₄) oder Lithiumaluminiumhydrid (LiAlH₄) in wasserfreien Lösungsmitteln.
Substitution: Verschiedene Nukleophile können unter sauren oder basischen Bedingungen verwendet werden, um die Ketogruppe zu substituieren.
Hauptsächlich gebildete Produkte:
Oxidation: Cholest-4-en-3-on.
Reduktion: Cholestan-3-ol.
Substitution: Abhängig vom verwendeten Nukleophil können verschiedene substituierte Produkte gebildet werden.
Wissenschaftliche Forschungsanwendungen
Cholestan-3-on hat verschiedene Anwendungen in der wissenschaftlichen Forschung:
Chemie: Es wird als Zwischenprodukt bei der Synthese anderer steroidaler Verbindungen verwendet.
Biologie: Cholestan-3-on wird für seine Rolle in Stoffwechselwegen und seine Auswirkungen auf zelluläre Prozesse untersucht.
Industrie: Cholestan-3-on wird bei der Herstellung verschiedener steroidbasierter Pharmazeutika und als Vorläufer bei der Synthese anderer bioaktiver Verbindungen verwendet.
5. Wirkmechanismus
Der Wirkmechanismus von Cholestan-3-on beinhaltet seine Wechselwirkung mit spezifischen molekularen Zielstrukturen und -wegen. Es ist bekannt, den Cholesterinstoffwechsel zu beeinflussen und kann die Aktivität von Enzymen beeinflussen, die an der Lipidbiosynthese beteiligt sind. Cholestan-3-on kann auch seine Wirkungen durch Bindung an Kernrezeptoren und Veränderung der Genexpression im Zusammenhang mit dem Lipidstoffwechsel ausüben .
Wirkmechanismus
The mechanism of action of cholestan-3-one involves its interaction with specific molecular targets and pathways. It is known to influence cholesterol metabolism and can modulate the activity of enzymes involved in lipid biosynthesis. Cholestan-3-one may also exert its effects by binding to nuclear receptors and altering gene expression related to lipid metabolism .
Vergleich Mit ähnlichen Verbindungen
Cholestan-3-on kann mit anderen ähnlichen Verbindungen verglichen werden, wie zum Beispiel:
Cholest-4-en-3-on: Diese Verbindung hat eine Doppelbindung an der vierten Kohlenstoffposition, wodurch sie in bestimmten chemischen Reaktionen reaktiver ist.
Cholestan-3-ol: Die reduzierte Form von Cholestan-3-on, die eine Hydroxylgruppe anstelle einer Ketogruppe an der dritten Kohlenstoffposition aufweist.
Coprostan-3-on: Ein Stereoisomer von Cholestan-3-on mit unterschiedlicher räumlicher Anordnung der Atome.
Eindeutigkeit: Cholestan-3-on ist aufgrund seiner spezifischen Struktur und des Vorhandenseins einer Ketogruppe an der dritten Kohlenstoffposition einzigartig. Diese strukturelle Eigenschaft ermöglicht es ihm, an verschiedenen biochemischen Reaktionen teilzunehmen, und macht es zu einem wertvollen Zwischenprodukt bei der Synthese anderer steroidaler Verbindungen .
Eigenschaften
CAS-Nummer |
601-53-6 |
---|---|
Molekularformel |
C27H46O |
Molekulargewicht |
386.7 g/mol |
IUPAC-Name |
10,13-dimethyl-17-(6-methylheptan-2-yl)-1,2,4,5,6,7,8,9,11,12,14,15,16,17-tetradecahydrocyclopenta[a]phenanthren-3-one |
InChI |
InChI=1S/C27H46O/c1-18(2)7-6-8-19(3)23-11-12-24-22-10-9-20-17-21(28)13-15-26(20,4)25(22)14-16-27(23,24)5/h18-20,22-25H,6-17H2,1-5H3 |
InChI-Schlüssel |
PESKGJQREUXSRR-UHFFFAOYSA-N |
SMILES |
CC(C)CCCC(C)C1CCC2C1(CCC3C2CCC4C3(CCC(=O)C4)C)C |
Isomerische SMILES |
C[C@H](CCCC(C)C)[C@H]1CC[C@@H]2[C@@]1(CC[C@H]3[C@H]2CC[C@H]4[C@@]3(CCC(=O)C4)C)C |
Kanonische SMILES |
CC(C)CCCC(C)C1CCC2C1(CCC3C2CCC4C3(CCC(=O)C4)C)C |
601-53-6 | |
Physikalische Beschreibung |
Solid |
Synonyme |
(5β,17β)-17-Octylandrostan-3-one; 3-Coprostanone; 5β-Cholestanone; 5β-Coprostan-3-one; Coprostanone; NSC 119031; β-Cholestanone |
Herkunft des Produkts |
United States |
Synthesis routes and methods
Procedure details
Retrosynthesis Analysis
AI-Powered Synthesis Planning: Our tool employs the Template_relevance Pistachio, Template_relevance Bkms_metabolic, Template_relevance Pistachio_ringbreaker, Template_relevance Reaxys, Template_relevance Reaxys_biocatalysis model, leveraging a vast database of chemical reactions to predict feasible synthetic routes.
One-Step Synthesis Focus: Specifically designed for one-step synthesis, it provides concise and direct routes for your target compounds, streamlining the synthesis process.
Accurate Predictions: Utilizing the extensive PISTACHIO, BKMS_METABOLIC, PISTACHIO_RINGBREAKER, REAXYS, REAXYS_BIOCATALYSIS database, our tool offers high-accuracy predictions, reflecting the latest in chemical research and data.
Strategy Settings
Precursor scoring | Relevance Heuristic |
---|---|
Min. plausibility | 0.01 |
Model | Template_relevance |
Template Set | Pistachio/Bkms_metabolic/Pistachio_ringbreaker/Reaxys/Reaxys_biocatalysis |
Top-N result to add to graph | 6 |
Feasible Synthetic Routes
Haftungsausschluss und Informationen zu In-Vitro-Forschungsprodukten
Bitte beachten Sie, dass alle Artikel und Produktinformationen, die auf BenchChem präsentiert werden, ausschließlich zu Informationszwecken bestimmt sind. Die auf BenchChem zum Kauf angebotenen Produkte sind speziell für In-vitro-Studien konzipiert, die außerhalb lebender Organismen durchgeführt werden. In-vitro-Studien, abgeleitet von dem lateinischen Begriff "in Glas", beinhalten Experimente, die in kontrollierten Laborumgebungen unter Verwendung von Zellen oder Geweben durchgeführt werden. Es ist wichtig zu beachten, dass diese Produkte nicht als Arzneimittel oder Medikamente eingestuft sind und keine Zulassung der FDA für die Vorbeugung, Behandlung oder Heilung von medizinischen Zuständen, Beschwerden oder Krankheiten erhalten haben. Wir müssen betonen, dass jede Form der körperlichen Einführung dieser Produkte in Menschen oder Tiere gesetzlich strikt untersagt ist. Es ist unerlässlich, sich an diese Richtlinien zu halten, um die Einhaltung rechtlicher und ethischer Standards in Forschung und Experiment zu gewährleisten.