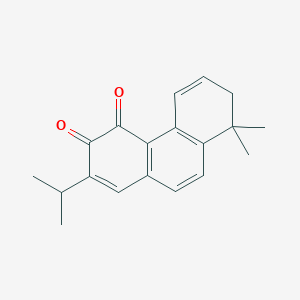
Dehydromiltirone
Übersicht
Beschreibung
Wissenschaftliche Forschungsanwendungen
Chemistry: Used as a precursor for synthesizing other bioactive compounds.
Biology: Investigated for its role in modulating cellular signaling pathways.
Medicine: Explored for its therapeutic potential in treating inflammatory diseases, osteoporosis, and cancer.
Industry: Utilized in the development of pharmaceuticals and nutraceuticals .
Wirkmechanismus
Target of Action
Dehydromiltirone, an active component of Salvia miltiorrhiza (SM), primarily targets PIK3CA . PIK3CA is a critical component of the PI3K-AKT signaling pathway , which plays a significant role in cellular functions such as cell growth, proliferation, differentiation, motility, survival and intracellular trafficking .
Mode of Action
This compound interacts with its target, PIK3CA, by binding to its functional pockets . This binding potentially inhibits PIK3CA, thereby downregulating the over-activation of the PI3K-AKT signaling pathway .
Biochemical Pathways
The primary biochemical pathways affected by this compound are the PI3K-AKT signaling pathway and the AGE-RAGE signaling pathway in diabetes complications . By inhibiting PIK3CA, this compound reduces the over-activation of the PI3K-AKT signaling pathway . This pathway is crucial for many cellular processes, including cell growth and survival .
Result of Action
This compound’s action results in the reduction of the expression of phenotypic switching markers α-SMA, Col-I, and FN in mesangial cells . This effect is achieved by downregulating the over-activation of the PI3K-AKT signaling pathway through the inhibition of PIK3CA . In a diabetic kidney disease (DKD) mouse model, this compound was found to reduce proteinuria and improve glomerular hypertrophy .
Biochemische Analyse
Biochemical Properties
Dehydromiltirone interacts with several enzymes and proteins. It has been shown to bind to functional PIK3CA pockets, thereby potentially inhibiting PIK3CA . This interaction plays a crucial role in the PI3K-AKT signaling pathway .
Cellular Effects
This compound has significant effects on various types of cells and cellular processes. For instance, it has been shown to reduce the expression of phenotypic switching markers α-SMA, Col-I, and FN in human mesangial cells (HMCs) by downregulating the over-activation of the PI3K-AKT signaling pathway . In osteoclasts, this compound inhibits differentiation by modulating MAPK and NF-κB activity .
Molecular Mechanism
This compound exerts its effects at the molecular level through several mechanisms. It binds to functional PIK3CA pockets, potentially inhibiting PIK3CA . This interaction plays a crucial role in the PI3K-AKT signaling pathway, influencing gene expression and enzyme activation .
Temporal Effects in Laboratory Settings
In laboratory settings, this compound has shown to have long-term effects on cellular function. For instance, it has been found to ameliorate renal function loss and renal tissue damage in a diabetic kidney disease mouse model .
Dosage Effects in Animal Models
In animal models, the effects of this compound vary with different dosages. For example, in a diabetic kidney disease mouse model, different concentrations of this compound could reduce body weight, serum creatinine, blood urea nitrogen, and proteinuria, with high-dose this compound having a more significant effect .
Metabolic Pathways
This compound is involved in several metabolic pathways. It plays a role in the PI3K-AKT signaling pathway and the AGE-RAGE signaling pathway in diabetes complications .
Transport and Distribution
Its ability to bind to functional PIK3CA pockets suggests that it may interact with transporters or binding proteins .
Vorbereitungsmethoden
Synthetic Routes and Reaction Conditions: Dehydromiltirone can be synthesized through various chemical reactions involving diterpenoid precursors. One common method involves the oxidative cyclization of miltirone, another diterpenoid quinone, under controlled conditions. The reaction typically requires the presence of oxidizing agents such as potassium permanganate or chromium trioxide in an organic solvent like dichloromethane .
Industrial Production Methods: Industrial production of this compound often involves the extraction and purification of the compound from Salvia miltiorrhiza roots. The roots are first dried and ground into a fine powder, which is then subjected to solvent extraction using ethanol or methanol. The extract is further purified through chromatographic techniques to isolate this compound .
Analyse Chemischer Reaktionen
Types of Reactions: Dehydromiltirone undergoes various chemical reactions, including:
Oxidation: this compound can be oxidized to form more complex quinone derivatives.
Reduction: Reduction reactions can convert this compound into its corresponding hydroquinone form.
Substitution: The compound can undergo substitution reactions, particularly at the quinone moiety, to form various derivatives
Common Reagents and Conditions:
Oxidation: Potassium permanganate, chromium trioxide, and hydrogen peroxide in organic solvents.
Reduction: Sodium borohydride or lithium aluminum hydride in ethanol or tetrahydrofuran.
Substitution: Halogenating agents like bromine or chlorine in the presence of a catalyst
Major Products Formed:
Oxidation: Complex quinone derivatives.
Reduction: Hydroquinone derivatives.
Substitution: Halogenated quinone derivatives
Vergleich Mit ähnlichen Verbindungen
Dehydromiltirone is unique compared to other diterpenoid quinones due to its specific molecular structure and pharmacological properties. Similar compounds include:
Miltirone: Another diterpenoid quinone with similar anti-inflammatory and anti-cancer activities.
Cryptotanshinone: Known for its anti-cancer and anti-inflammatory properties.
Tanshinone IIA: Widely studied for its cardiovascular protective effects .
Eigenschaften
IUPAC Name |
8,8-dimethyl-2-propan-2-yl-7H-phenanthrene-3,4-dione | |
---|---|---|
Source | PubChem | |
URL | https://pubchem.ncbi.nlm.nih.gov | |
Description | Data deposited in or computed by PubChem | |
InChI |
InChI=1S/C19H20O2/c1-11(2)14-10-12-7-8-15-13(6-5-9-19(15,3)4)16(12)18(21)17(14)20/h5-8,10-11H,9H2,1-4H3 | |
Source | PubChem | |
URL | https://pubchem.ncbi.nlm.nih.gov | |
Description | Data deposited in or computed by PubChem | |
InChI Key |
FQRLDPKLRMEKLQ-UHFFFAOYSA-N | |
Source | PubChem | |
URL | https://pubchem.ncbi.nlm.nih.gov | |
Description | Data deposited in or computed by PubChem | |
Canonical SMILES |
CC(C)C1=CC2=C(C3=C(C=C2)C(CC=C3)(C)C)C(=O)C1=O | |
Source | PubChem | |
URL | https://pubchem.ncbi.nlm.nih.gov | |
Description | Data deposited in or computed by PubChem | |
Molecular Formula |
C19H20O2 | |
Source | PubChem | |
URL | https://pubchem.ncbi.nlm.nih.gov | |
Description | Data deposited in or computed by PubChem | |
DSSTOX Substance ID |
DTXSID50151273 | |
Record name | Dehydromiltirone | |
Source | EPA DSSTox | |
URL | https://comptox.epa.gov/dashboard/DTXSID50151273 | |
Description | DSSTox provides a high quality public chemistry resource for supporting improved predictive toxicology. | |
Molecular Weight |
280.4 g/mol | |
Source | PubChem | |
URL | https://pubchem.ncbi.nlm.nih.gov | |
Description | Data deposited in or computed by PubChem | |
CAS No. |
116064-77-8 | |
Record name | Dehydromiltirone | |
Source | ChemIDplus | |
URL | https://pubchem.ncbi.nlm.nih.gov/substance/?source=chemidplus&sourceid=0116064778 | |
Description | ChemIDplus is a free, web search system that provides access to the structure and nomenclature authority files used for the identification of chemical substances cited in National Library of Medicine (NLM) databases, including the TOXNET system. | |
Record name | Dehydromiltirone | |
Source | EPA DSSTox | |
URL | https://comptox.epa.gov/dashboard/DTXSID50151273 | |
Description | DSSTox provides a high quality public chemistry resource for supporting improved predictive toxicology. | |
Record name | DEHYDROMILTIRONE | |
Source | FDA Global Substance Registration System (GSRS) | |
URL | https://gsrs.ncats.nih.gov/ginas/app/beta/substances/RPP9EW3OBQ | |
Description | The FDA Global Substance Registration System (GSRS) enables the efficient and accurate exchange of information on what substances are in regulated products. Instead of relying on names, which vary across regulatory domains, countries, and regions, the GSRS knowledge base makes it possible for substances to be defined by standardized, scientific descriptions. | |
Explanation | Unless otherwise noted, the contents of the FDA website (www.fda.gov), both text and graphics, are not copyrighted. They are in the public domain and may be republished, reprinted and otherwise used freely by anyone without the need to obtain permission from FDA. Credit to the U.S. Food and Drug Administration as the source is appreciated but not required. | |
Retrosynthesis Analysis
AI-Powered Synthesis Planning: Our tool employs the Template_relevance Pistachio, Template_relevance Bkms_metabolic, Template_relevance Pistachio_ringbreaker, Template_relevance Reaxys, Template_relevance Reaxys_biocatalysis model, leveraging a vast database of chemical reactions to predict feasible synthetic routes.
One-Step Synthesis Focus: Specifically designed for one-step synthesis, it provides concise and direct routes for your target compounds, streamlining the synthesis process.
Accurate Predictions: Utilizing the extensive PISTACHIO, BKMS_METABOLIC, PISTACHIO_RINGBREAKER, REAXYS, REAXYS_BIOCATALYSIS database, our tool offers high-accuracy predictions, reflecting the latest in chemical research and data.
Strategy Settings
Precursor scoring | Relevance Heuristic |
---|---|
Min. plausibility | 0.01 |
Model | Template_relevance |
Template Set | Pistachio/Bkms_metabolic/Pistachio_ringbreaker/Reaxys/Reaxys_biocatalysis |
Top-N result to add to graph | 6 |
Feasible Synthetic Routes
Q1: What are the known mechanisms of action for Dehydromiltirone (DHT)?
A1: DHT has been shown to exert its effects through multiple pathways. Studies suggest that DHT can inhibit the phenotypic switching of mesangial cells by targeting the PIK3CA signaling pathway []. It has also demonstrated the ability to modulate MAPK and NF-κB activity, impacting osteoclast differentiation []. Furthermore, research indicates DHT may protect against liver injury by inhibiting p38 and NFκB signaling in Kupffer cells [].
Q2: What is the molecular formula and weight of this compound?
A2: this compound has a molecular formula of C20H22O5 and a molecular weight of 342.39 g/mol.
Q3: Can you elaborate on the structural features of DHT and their relation to its antioxidant activity?
A3: DHT belongs to the abietane-type diterpenoid quinone family. Quantum chemical calculations suggest that DHT, like other quinone antioxidants, may exert its antioxidant effects by forming stable complex radicals with carbon-centered radicals in fats []. This interaction helps to retard lipid rancidity. The specific structural features of DHT, such as its quinone moiety and the presence of specific functional groups, contribute to its ability to scavenge free radicals and protect against oxidative damage.
Q4: What in vitro models have been used to investigate the effects of DHT?
A4: Researchers have utilized various in vitro models to study DHT's effects. These include:* Human Mesangial Cells (HMCs): DHT has been shown to reduce the expression of phenotypic switching markers (α-SMA, Col-I, and FN) in HMCs, suggesting its potential in addressing diabetic kidney disease [].* RAW264.7 and Bone Marrow Macrophages: In these models, DHT exhibited an inhibitory effect on osteoclast differentiation, suggesting its potential in osteoporosis treatment [].* HK-2 Cells: Pretreatment with DHT protected HK-2 cells from LPS-induced injury, decreasing oxidative stress, mitochondrial dysfunction, and apoptosis [].
Q5: How effective is DHT in in vivo models of disease?
A5: DHT has shown promising results in several in vivo models:
- Diabetic Kidney Disease (DKD) Mouse Model: DHT administration improved renal function, reduced proteinuria, and ameliorated glomerular hypertrophy [].
- Sepsis-Induced AKI Mouse Model: Pretreatment with DHT protected against renal function loss and tissue damage induced by CLP surgery. It also attenuated the production of inflammatory mediators (IL-6, IL-1β, TNF-α, MCP-1) [].
- Rat Model of Post-Hypoxie Cardiac Contractile Force Recovery: Tanshindiol B, a related compound, significantly improved cardiac contractile force recovery following hypoxic perfusion and reoxygenation [].
Q6: Have any studies investigated the potential of DHT in treating thrombotic diseases?
A6: While not directly investigated in clinical trials, network pharmacological analysis suggests that DHT, along with other compounds found in Salvia miltiorrhiza, may hold potential for treating thrombotic diseases. This prediction stems from its potential to interact with targets involved in pathways related to the endocrine system, signal transduction, cell motility, environmental adaptation, and the nervous system [].
Q7: What is the historical context of this compound research?
A7: DHT was first isolated and characterized from the roots of Salvia prionitis Hance []. Since then, research has expanded to explore its various pharmacological activities, including its antioxidant, anti-inflammatory, and cytoprotective effects in various disease models [, , , , ].
Q8: What are the limitations of current this compound research?
A8: While promising, current research on DHT has limitations. Most studies are preclinical, utilizing in vitro and in vivo models. Further research, including well-designed clinical trials, is necessary to determine its efficacy and safety in humans. Additionally, a comprehensive understanding of its pharmacokinetic properties, potential drug interactions, long-term effects, and optimal dosage regimens is crucial for its clinical translation.
Q9: Are there any analytical methods for identifying and quantifying this compound?
A9: Yes, researchers have successfully utilized ultra-high-performance liquid chromatography coupled with linear ion trap-Orbitrap mass spectrometry (UHPLC-LTQ-Orbitrap MS) for the rapid identification and quantification of DHT in complex mixtures, particularly from Salvia miltiorrhiza []. This advanced analytical technique allows for sensitive and specific detection of DHT, enabling researchers to study its presence and abundance in various samples.
Haftungsausschluss und Informationen zu In-Vitro-Forschungsprodukten
Bitte beachten Sie, dass alle Artikel und Produktinformationen, die auf BenchChem präsentiert werden, ausschließlich zu Informationszwecken bestimmt sind. Die auf BenchChem zum Kauf angebotenen Produkte sind speziell für In-vitro-Studien konzipiert, die außerhalb lebender Organismen durchgeführt werden. In-vitro-Studien, abgeleitet von dem lateinischen Begriff "in Glas", beinhalten Experimente, die in kontrollierten Laborumgebungen unter Verwendung von Zellen oder Geweben durchgeführt werden. Es ist wichtig zu beachten, dass diese Produkte nicht als Arzneimittel oder Medikamente eingestuft sind und keine Zulassung der FDA für die Vorbeugung, Behandlung oder Heilung von medizinischen Zuständen, Beschwerden oder Krankheiten erhalten haben. Wir müssen betonen, dass jede Form der körperlichen Einführung dieser Produkte in Menschen oder Tiere gesetzlich strikt untersagt ist. Es ist unerlässlich, sich an diese Richtlinien zu halten, um die Einhaltung rechtlicher und ethischer Standards in Forschung und Experiment zu gewährleisten.