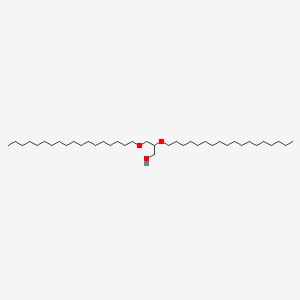
1,2-O-Dioctadecyl-rac-Glycerol
Übersicht
Beschreibung
1,2-O-Dioctadecyl-rac-glycerol is a saturated dialkyl glyceryl ether with octadecyl groups at the sn-1 and sn-2 positions, which mimics the structure of diacylglycerol. It has been incorporated into model lipid bilayers in order to study the dynamics of various biological membrane compositions.
Wissenschaftliche Forschungsanwendungen
Nachahmung von Diacylglycerol
“1,2-O-Dioctadecyl-rac-Glycerol” ist ein gesättigter Dialkylglycerylether mit Octadecylgruppen an den sn-1- und sn-2-Positionen, der die Struktur von Diacylglycerol nachahmt . Dies macht es nützlich in Studien, die ein stabiles, nicht metabolisierbares Analog von Diacylglycerol erfordern .
Studium von Lipiddoppelschichten
Diese Verbindung wurde in Modell-Lipiddoppelschichten integriert, um die Dynamik verschiedener biologischer Membranzusammensetzungen zu untersuchen . Durch die Integration von “this compound” in diese Modelle können Forscher Einblicke in das Verhalten von Lipiden in biologischen Membranen gewinnen .
Lipidbiochemie
“this compound” fällt unter die Kategorie der Glycerolipide, die eine Art von Lipidmolekül sind. Als solches wird es im Forschungsbereich der Lipidbiochemie verwendet . Dieser Bereich umfasst die Untersuchung der Struktur, Funktion und biologischen Rollen von Lipiden.
Lipidstandards
Als eine Art von Lipid könnte “this compound” möglicherweise als Standard in der Lipidforschung verwendet werden . Lipidstandards sind reine Substanzen, die zum Vergleich in der analytischen Chemie verwendet werden.
Lipide in Gesundheit & Krankheit
Aufgrund seiner Rolle als Nachahmung von Diacylglycerol könnte “this compound” möglicherweise in der Forschung verwendet werden, die die Rolle von Lipiden in Gesundheit und Krankheit untersucht
Wirkmechanismus
Target of Action
1,2-O-Dioctadecyl-rac-glycerol, also known as 2,3-Bis(octadecyloxy)propan-1-ol, is a saturated dialkyl glyceryl ether with octadecyl groups at the sn-1 and sn-2 positions . It mimics the structure of diacylglycerol , a secondary messenger in cells that activates protein kinase C (PKC). Therefore, the primary target of this compound can be inferred to be PKC.
Mode of Action
The compound interacts with its targets by mimicking the structure of diacylglycerol . Diacylglycerol activates PKC, which then phosphorylates a range of protein targets, leading to the activation or inhibition of these proteins . By mimicking diacylglycerol, this compound likely induces similar changes in protein activity.
Biochemical Pathways
The compound’s mimicry of diacylglycerol suggests it may affect the same biochemical pathways. Diacylglycerol is involved in the phosphatidylinositol signaling system, where it acts as a secondary messenger. This pathway plays a key role in various cellular processes, including cell growth, differentiation, and apoptosis .
Pharmacokinetics
Its solubility in various solvents has been reported: dmf: 20 mg/ml, dmso: 5 mg/ml, ethanol: 30 mg/ml, pbs (ph 72): 025 mg/ml . These solubility properties may influence its absorption, distribution, metabolism, and excretion (ADME) in the body.
Result of Action
The compound has been incorporated into model lipid bilayers to study the dynamics of various biological membrane compositions . The specific molecular and cellular effects of the compound’s action are likely dependent on the specific context of its use, such as the cell type and the presence of other signaling molecules.
Action Environment
Environmental factors such as temperature, pH, and the presence of other molecules can influence the compound’s action, efficacy, and stability. For instance, the compound’s solubility in different solvents suggests that it may be more effective in certain environments . .
Biochemische Analyse
Biochemical Properties
2,3-Bis(octadecyloxy)propan-1-ol plays a significant role in biochemical reactions, particularly in lipid metabolism. It interacts with enzymes such as lipases and esterases, which catalyze the hydrolysis of ester bonds in lipids . The compound’s long hydrophobic chains allow it to integrate into lipid bilayers, affecting membrane fluidity and permeability. Additionally, 2,3-Bis(octadecyloxy)propan-1-ol can interact with proteins involved in lipid transport and storage, influencing lipid homeostasis within cells .
Cellular Effects
The effects of 2,3-Bis(octadecyloxy)propan-1-ol on various cell types and cellular processes are profound. It has been shown to modulate cell signaling pathways, particularly those involved in lipid signaling . By altering the composition of cellular membranes, it can impact the activity of membrane-bound receptors and enzymes, leading to changes in gene expression and cellular metabolism. For instance, 2,3-Bis(octadecyloxy)propan-1-ol can influence the expression of genes involved in lipid synthesis and degradation, thereby affecting overall lipid metabolism .
Molecular Mechanism
At the molecular level, 2,3-Bis(octadecyloxy)propan-1-ol exerts its effects through several mechanisms. It can bind to specific lipid-binding domains in proteins, altering their conformation and activity . This binding can either inhibit or activate enzymes, depending on the context. For example, the compound may inhibit lipases by blocking their active sites, preventing the hydrolysis of triglycerides . Additionally, 2,3-Bis(octadecyloxy)propan-1-ol can modulate gene expression by interacting with transcription factors and other regulatory proteins involved in lipid metabolism .
Temporal Effects in Laboratory Settings
In laboratory settings, the effects of 2,3-Bis(octadecyloxy)propan-1-ol can change over time. The compound is relatively stable under standard storage conditions, but its activity may decrease upon prolonged exposure to light and air . In in vitro studies, the compound has been observed to maintain its effects on cellular function for several hours, with gradual degradation over time . Long-term studies in vivo have shown that 2,3-Bis(octadecyloxy)propan-1-ol can have lasting effects on lipid metabolism, although its impact may diminish as the compound is metabolized and excreted .
Dosage Effects in Animal Models
The effects of 2,3-Bis(octadecyloxy)propan-1-ol vary with different dosages in animal models. At low doses, the compound can enhance lipid metabolism and improve lipid homeostasis . At higher doses, it may exhibit toxic effects, such as liver damage and disruption of normal lipid metabolism . Threshold effects have been observed, where the compound’s beneficial effects plateau and adverse effects become more pronounced with increasing dosage .
Metabolic Pathways
2,3-Bis(octadecyloxy)propan-1-ol is involved in several metabolic pathways related to lipid metabolism. It interacts with enzymes such as acyl-CoA synthetases and acyltransferases, which are involved in the activation and transfer of fatty acids . The compound can also affect metabolic flux by altering the levels of key metabolites, such as triglycerides and phospholipids . These interactions can influence the overall balance of lipid synthesis and degradation within cells .
Transport and Distribution
Within cells and tissues, 2,3-Bis(octadecyloxy)propan-1-ol is transported and distributed through interactions with lipid transport proteins and lipoproteins . It can be incorporated into lipid droplets and transported to various cellular compartments, including the endoplasmic reticulum and mitochondria . The compound’s hydrophobic nature allows it to accumulate in lipid-rich tissues, such as adipose tissue and the liver .
Subcellular Localization
The subcellular localization of 2,3-Bis(octadecyloxy)propan-1-ol is primarily within lipid droplets and cellular membranes . It can be targeted to specific compartments through post-translational modifications and interactions with targeting signals . The compound’s localization can influence its activity and function, as it may interact with different sets of proteins and enzymes depending on its subcellular distribution .
Biologische Aktivität
2,3-Bis(octadecyloxy)propan-1-ol, a compound with the molecular formula , is recognized for its significant biological activities attributed to its unique structural characteristics. This article explores its biological activity, mechanisms of action, and potential applications in various fields, particularly in cellular signaling and membrane dynamics.
Structural Characteristics
The compound features two long octadecyloxy chains attached to a propane backbone. This structure enhances its hydrophobic properties, allowing it to integrate into lipid bilayers and mimic diacylglycerols, which are crucial components of cellular membranes. The hydrophobic nature of 2,3-Bis(octadecyloxy)propan-1-ol plays a vital role in its interaction with biological membranes and proteins.
2,3-Bis(octadecyloxy)propan-1-ol's biological activity can be primarily attributed to its ability to influence membrane dynamics and protein interactions. The following mechanisms have been identified:
- Membrane Integration : By incorporating into lipid bilayers, it alters membrane fluidity and permeability, which can affect cellular signaling pathways.
- Mimicking Glycerol : Its structural similarity to glycerol allows it to participate in various biochemical processes, including lipid metabolism and cell signaling.
- Interaction with Antimicrobial Peptides : Research indicates that this compound can modulate the activity of antimicrobial peptides by affecting their interaction with membranes, providing insights into their mechanisms of action against pathogens .
Biological Activity Overview
The biological activities of 2,3-Bis(octadecyloxy)propan-1-ol include:
- Gene Silencing : Studies have demonstrated that derivatives of this compound can effectively facilitate gene silencing in HeLa cells without the need for transfection agents. This capability suggests potential applications in gene therapy and molecular biology .
- Antimicrobial Properties : Its role in enhancing the efficacy of antimicrobial peptides indicates potential applications in developing new antimicrobial agents .
Gene Silencing Efficacy
In a study focused on siRNA delivery systems, 2,3-Bis(octadecyloxy)propan-1-ol was used as a carrier for siRNAs. The results showed that siRNAs modified with this compound exhibited effective gene silencing capabilities comparable to traditional methods. The IC50 values ranged from 56 nM to 141 nM across different formulations, demonstrating its potential as a non-toxic delivery vehicle for therapeutic nucleic acids .
Antimicrobial Interaction Studies
Research involving the interaction of 2,3-Bis(octadecyloxy)propan-1-ol with various antimicrobial peptides revealed significant insights into how these peptides disrupt bacterial membranes. The compound's ability to alter membrane properties enhanced the penetration and efficacy of these peptides against resistant strains of bacteria .
Comparative Analysis
The following table summarizes key characteristics and biological activities of similar compounds:
Compound Name | Molecular Formula | Unique Features | Biological Activity |
---|---|---|---|
2,3-Bis(octadecyloxy)propan-1-ol | C39H80O3 | Long alkyl chains; hydrophobic | Gene silencing; antimicrobial interactions |
1,2-Dihexadecyl-sn-glycero-3-phosphocholine | C36H75NO8P | Contains a phosphate group; involved in signaling | Membrane dynamics; cell signaling |
1,3-Bis(hexadecyloxy)propan-2-ol | C35H72O3 | Similar structure but different alkoxy positioning | Lipid metabolism; membrane integration |
Eigenschaften
IUPAC Name |
2,3-dioctadecoxypropan-1-ol | |
---|---|---|
Source | PubChem | |
URL | https://pubchem.ncbi.nlm.nih.gov | |
Description | Data deposited in or computed by PubChem | |
InChI |
InChI=1S/C39H80O3/c1-3-5-7-9-11-13-15-17-19-21-23-25-27-29-31-33-35-41-38-39(37-40)42-36-34-32-30-28-26-24-22-20-18-16-14-12-10-8-6-4-2/h39-40H,3-38H2,1-2H3 | |
Source | PubChem | |
URL | https://pubchem.ncbi.nlm.nih.gov | |
Description | Data deposited in or computed by PubChem | |
InChI Key |
QEHCYTDFERPPPU-UHFFFAOYSA-N | |
Source | PubChem | |
URL | https://pubchem.ncbi.nlm.nih.gov | |
Description | Data deposited in or computed by PubChem | |
Canonical SMILES |
CCCCCCCCCCCCCCCCCCOCC(CO)OCCCCCCCCCCCCCCCCCC | |
Source | PubChem | |
URL | https://pubchem.ncbi.nlm.nih.gov | |
Description | Data deposited in or computed by PubChem | |
Molecular Formula |
C39H80O3 | |
Source | PubChem | |
URL | https://pubchem.ncbi.nlm.nih.gov | |
Description | Data deposited in or computed by PubChem | |
DSSTOX Substance ID |
DTXSID40884220 | |
Record name | 1-Propanol, 2,3-bis(octadecyloxy)- | |
Source | EPA DSSTox | |
URL | https://comptox.epa.gov/dashboard/DTXSID40884220 | |
Description | DSSTox provides a high quality public chemistry resource for supporting improved predictive toxicology. | |
Molecular Weight |
597.0 g/mol | |
Source | PubChem | |
URL | https://pubchem.ncbi.nlm.nih.gov | |
Description | Data deposited in or computed by PubChem | |
CAS No. |
6076-38-6 | |
Record name | 2,3-Bis(octadecyloxy)-1-propanol | |
Source | CAS Common Chemistry | |
URL | https://commonchemistry.cas.org/detail?cas_rn=6076-38-6 | |
Description | CAS Common Chemistry is an open community resource for accessing chemical information. Nearly 500,000 chemical substances from CAS REGISTRY cover areas of community interest, including common and frequently regulated chemicals, and those relevant to high school and undergraduate chemistry classes. This chemical information, curated by our expert scientists, is provided in alignment with our mission as a division of the American Chemical Society. | |
Explanation | The data from CAS Common Chemistry is provided under a CC-BY-NC 4.0 license, unless otherwise stated. | |
Record name | 1-Propanol, 2,3-bis(octadecyloxy)- | |
Source | ChemIDplus | |
URL | https://pubchem.ncbi.nlm.nih.gov/substance/?source=chemidplus&sourceid=0006076386 | |
Description | ChemIDplus is a free, web search system that provides access to the structure and nomenclature authority files used for the identification of chemical substances cited in National Library of Medicine (NLM) databases, including the TOXNET system. | |
Record name | 1-Propanol, 2,3-bis(octadecyloxy)- | |
Source | EPA Chemicals under the TSCA | |
URL | https://www.epa.gov/chemicals-under-tsca | |
Description | EPA Chemicals under the Toxic Substances Control Act (TSCA) collection contains information on chemicals and their regulations under TSCA, including non-confidential content from the TSCA Chemical Substance Inventory and Chemical Data Reporting. | |
Record name | 1-Propanol, 2,3-bis(octadecyloxy)- | |
Source | EPA DSSTox | |
URL | https://comptox.epa.gov/dashboard/DTXSID40884220 | |
Description | DSSTox provides a high quality public chemistry resource for supporting improved predictive toxicology. | |
Record name | 2,3-bis(octadecyloxy)propan-1-ol | |
Source | European Chemicals Agency (ECHA) | |
URL | https://echa.europa.eu/substance-information/-/substanceinfo/100.025.466 | |
Description | The European Chemicals Agency (ECHA) is an agency of the European Union which is the driving force among regulatory authorities in implementing the EU's groundbreaking chemicals legislation for the benefit of human health and the environment as well as for innovation and competitiveness. | |
Explanation | Use of the information, documents and data from the ECHA website is subject to the terms and conditions of this Legal Notice, and subject to other binding limitations provided for under applicable law, the information, documents and data made available on the ECHA website may be reproduced, distributed and/or used, totally or in part, for non-commercial purposes provided that ECHA is acknowledged as the source: "Source: European Chemicals Agency, http://echa.europa.eu/". Such acknowledgement must be included in each copy of the material. ECHA permits and encourages organisations and individuals to create links to the ECHA website under the following cumulative conditions: Links can only be made to webpages that provide a link to the Legal Notice page. | |
Retrosynthesis Analysis
AI-Powered Synthesis Planning: Our tool employs the Template_relevance Pistachio, Template_relevance Bkms_metabolic, Template_relevance Pistachio_ringbreaker, Template_relevance Reaxys, Template_relevance Reaxys_biocatalysis model, leveraging a vast database of chemical reactions to predict feasible synthetic routes.
One-Step Synthesis Focus: Specifically designed for one-step synthesis, it provides concise and direct routes for your target compounds, streamlining the synthesis process.
Accurate Predictions: Utilizing the extensive PISTACHIO, BKMS_METABOLIC, PISTACHIO_RINGBREAKER, REAXYS, REAXYS_BIOCATALYSIS database, our tool offers high-accuracy predictions, reflecting the latest in chemical research and data.
Strategy Settings
Precursor scoring | Relevance Heuristic |
---|---|
Min. plausibility | 0.01 |
Model | Template_relevance |
Template Set | Pistachio/Bkms_metabolic/Pistachio_ringbreaker/Reaxys/Reaxys_biocatalysis |
Top-N result to add to graph | 6 |
Feasible Synthetic Routes
Haftungsausschluss und Informationen zu In-Vitro-Forschungsprodukten
Bitte beachten Sie, dass alle Artikel und Produktinformationen, die auf BenchChem präsentiert werden, ausschließlich zu Informationszwecken bestimmt sind. Die auf BenchChem zum Kauf angebotenen Produkte sind speziell für In-vitro-Studien konzipiert, die außerhalb lebender Organismen durchgeführt werden. In-vitro-Studien, abgeleitet von dem lateinischen Begriff "in Glas", beinhalten Experimente, die in kontrollierten Laborumgebungen unter Verwendung von Zellen oder Geweben durchgeführt werden. Es ist wichtig zu beachten, dass diese Produkte nicht als Arzneimittel oder Medikamente eingestuft sind und keine Zulassung der FDA für die Vorbeugung, Behandlung oder Heilung von medizinischen Zuständen, Beschwerden oder Krankheiten erhalten haben. Wir müssen betonen, dass jede Form der körperlichen Einführung dieser Produkte in Menschen oder Tiere gesetzlich strikt untersagt ist. Es ist unerlässlich, sich an diese Richtlinien zu halten, um die Einhaltung rechtlicher und ethischer Standards in Forschung und Experiment zu gewährleisten.