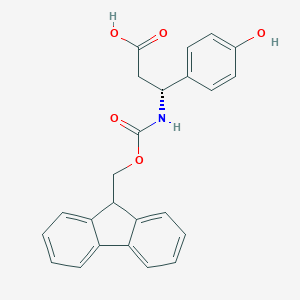
Fmoc-(R)-3-Amino-3-(4-hydroxy-phenyl)-propionsäure
Übersicht
Beschreibung
(R)-3-((((9H-Fluoren-9-yl)methoxy)carbonyl)amino)-3-(4-hydroxyphenyl)propanoic acid, also known as (R)-3-((((9H-Fluoren-9-yl)methoxy)carbonyl)amino)-3-(4-hydroxyphenyl)propanoic acid, is a useful research compound. Its molecular formula is C24H21NO5 and its molecular weight is 403.4 g/mol. The purity is usually 95%.
BenchChem offers high-quality (R)-3-((((9H-Fluoren-9-yl)methoxy)carbonyl)amino)-3-(4-hydroxyphenyl)propanoic acid suitable for many research applications. Different packaging options are available to accommodate customers' requirements. Please inquire for more information about (R)-3-((((9H-Fluoren-9-yl)methoxy)carbonyl)amino)-3-(4-hydroxyphenyl)propanoic acid including the price, delivery time, and more detailed information at info@benchchem.com.
Wissenschaftliche Forschungsanwendungen
Peptidsynthese
Fmoc-®-3-Amino-3-(4-hydroxy-phenyl)-propionsäure wird oft in der Fmoc-basierten Festphasenpeptidsynthese verwendet . Diese Methode wird üblicherweise für die Herstellung von Peptiden verwendet, die Verbindungen sind, die aus zwei oder mehr Aminosäuren bestehen, die in einer Kette verbunden sind. Die Fmoc-Gruppe fungiert während des Syntheseprozesses als Schutzgruppe für die Aminosäure .
Medizinische Chemie
Phenylglycin-haltige Peptide, die mit Fmoc-®-3-Amino-3-(4-hydroxy-phenyl)-propionsäure synthetisiert werden können, haben breite Anwendungen in der medizinischen Chemie . Sie wurden bei der Entwicklung verschiedener antimikrobieller Peptide verwendet, wie z. B. Streptogramine und Glykopeptide .
Arzneimittelentwicklung
Boronsäuren und ihre Ester, die möglicherweise aus Fmoc-®-3-Amino-3-(4-hydroxy-phenyl)-propionsäure synthetisiert werden können, werden als vielversprechende Verbindungen für die Entwicklung neuer Medikamente und Medikamentenabgabesysteme angesehen . Sie eignen sich besonders für die Neutronen-Einfangtherapie .
Biologische Assays
Die mit Fmoc-®-3-Amino-3-(4-hydroxy-phenyl)-propionsäure synthetisierten Peptide haben in Pufferlösungen, die für biologische Assays verwendet werden, Stabilität gezeigt . Dadurch eignen sie sich für verschiedene biologische Forschungsanwendungen .
Biochemische Forschung
Fmoc-®-3-Amino-3-(4-hydroxy-phenyl)-propionsäure wird aufgrund ihrer Eigenschaften oft in der biochemischen Forschung verwendet . Es kann verwendet werden, um die Eigenschaften von Proteinen und anderen Biomolekülen zu untersuchen<a aria-label="3: Fmoc-®-3-Amino-3-(4-hydroxy-phenyl)-propionsäure wird aufgrund ihrer Eigenschaften oft in der biochemischen Forschung verwendet3" data-citationid="b74f8
Wirkmechanismus
Target of Action
The primary target of Fmoc-®-3-Amino-3-(4-hydroxy-phenyl)-propionic acid is 4-Hydroxyphenylpyruvate dioxygenase (HPPD) . HPPD is an enzyme found in nearly all aerobic forms of life . It catalyzes the second reaction in the catabolism of tyrosine - the conversion of 4-hydroxyphenylpyruvate into homogentisate .
Mode of Action
Fmoc-®-3-Amino-3-(4-hydroxy-phenyl)-propionic acid interacts with its target, HPPD, by inhibiting its function . HPPD is categorized within a class of oxygenase enzymes that usually utilize α-ketoglutarate and diatomic oxygen to oxygenate or oxidize a target molecule .
Biochemical Pathways
The inhibition of HPPD affects the catabolism of the amino acid tyrosine . This prevents the conversion of 4-hydroxyphenylpyruvate into homogentisate, which is a key precursor for the biosynthesis of both tocopherols and plastoquinone . Plastoquinone is a critical co-factor in the formation of carotenoids, which protect chlorophyll in plants from being destroyed by sunlight .
Pharmacokinetics
It’s known that the stereochemical stability of phenylglycine derivatives like this compound can be achieved by the choice of reaction conditions during synthesis .
Result of Action
The result of the action of Fmoc-®-3-Amino-3-(4-hydroxy-phenyl)-propionic acid is the inhibition of the HPPD enzyme . This leads to a disruption in the catabolism of tyrosine and the biosynthesis of tocopherols and plastoquinone . In plants, this can lead to a loss of chlorophyll due to the lack of protective carotenoids .
Biologische Aktivität
(R)-3-((((9H-Fluoren-9-yl)methoxy)carbonyl)amino)-3-(4-hydroxyphenyl)propanoic acid, often referred to as a fluorenyl derivative, is a compound of significant interest in medicinal chemistry due to its unique structural features and potential therapeutic applications. This article examines its biological activity, focusing on its antimicrobial, anti-inflammatory, and anticancer properties, supported by data tables and relevant case studies.
Structural Characteristics
The compound consists of several key components:
- Fluorenyl Group : Enhances lipophilicity and cellular uptake.
- Methoxycarbonyl Moiety : Contributes to the compound's solubility and reactivity.
- Amino Acid Backbone : Essential for biological interactions.
Biological Activity Overview
The biological activities of (R)-3-((((9H-Fluoren-9-yl)methoxy)carbonyl)amino)-3-(4-hydroxyphenyl)propanoic acid can be categorized as follows:
1. Antimicrobial Activity
Recent studies have demonstrated that derivatives of this compound exhibit notable antimicrobial properties. For instance, compounds structurally related to this fluorenyl derivative showed activity against various multidrug-resistant pathogens.
Pathogen | Minimum Inhibitory Concentration (MIC) |
---|---|
Methicillin-resistant S. aureus | 1 - 8 µg/mL |
Vancomycin-resistant E. faecalis | 0.5 - 2 µg/mL |
Gram-negative pathogens | 8 - 64 µg/mL |
Drug-resistant Candida species | 8 - 64 µg/mL |
These findings indicate the potential utility of this compound as a foundational platform for developing novel antimicrobial agents targeting emerging resistant strains .
2. Anti-inflammatory Properties
The fluorenyl derivatives have been shown to modulate inflammatory pathways, which may involve the inhibition of pro-inflammatory cytokines. Research indicates that the introduction of hydroxyl groups enhances anti-inflammatory activity, making these compounds promising candidates for treating inflammatory diseases .
3. Anticancer Activity
Compounds with similar structures have been investigated for their anticancer properties. The fluorenyl moiety is believed to interact with various signaling pathways involved in tumor growth and proliferation. For example, certain derivatives demonstrated significant antiproliferative activity against cancer cell lines through mechanisms involving topoisomerase inhibition .
Case Study 1: Antimicrobial Screening
In a study assessing the antimicrobial efficacy of various fluorenyl derivatives, one compound was tested against a panel of clinically relevant bacterial strains, including MRSA and drug-resistant E. faecalis. The results revealed that modifications in the phenolic ring significantly enhanced antimicrobial potency, particularly against Gram-positive bacteria .
Case Study 2: Anti-inflammatory Mechanism Exploration
Another study focused on the anti-inflammatory effects of (R)-3-((((9H-Fluoren-9-yl)methoxy)carbonyl)amino)-3-(4-hydroxyphenyl)propanoic acid. The researchers found that the compound reduced the production of TNF-alpha and IL-6 in activated macrophages, suggesting a potential mechanism for its anti-inflammatory action .
Eigenschaften
IUPAC Name |
(3R)-3-(9H-fluoren-9-ylmethoxycarbonylamino)-3-(4-hydroxyphenyl)propanoic acid | |
---|---|---|
Source | PubChem | |
URL | https://pubchem.ncbi.nlm.nih.gov | |
Description | Data deposited in or computed by PubChem | |
InChI |
InChI=1S/C24H21NO5/c26-16-11-9-15(10-12-16)22(13-23(27)28)25-24(29)30-14-21-19-7-3-1-5-17(19)18-6-2-4-8-20(18)21/h1-12,21-22,26H,13-14H2,(H,25,29)(H,27,28)/t22-/m1/s1 | |
Source | PubChem | |
URL | https://pubchem.ncbi.nlm.nih.gov | |
Description | Data deposited in or computed by PubChem | |
InChI Key |
VJELJMCALKYCRN-JOCHJYFZSA-N | |
Source | PubChem | |
URL | https://pubchem.ncbi.nlm.nih.gov | |
Description | Data deposited in or computed by PubChem | |
Canonical SMILES |
C1=CC=C2C(=C1)C(C3=CC=CC=C32)COC(=O)NC(CC(=O)O)C4=CC=C(C=C4)O | |
Source | PubChem | |
URL | https://pubchem.ncbi.nlm.nih.gov | |
Description | Data deposited in or computed by PubChem | |
Isomeric SMILES |
C1=CC=C2C(=C1)C(C3=CC=CC=C32)COC(=O)N[C@H](CC(=O)O)C4=CC=C(C=C4)O | |
Source | PubChem | |
URL | https://pubchem.ncbi.nlm.nih.gov | |
Description | Data deposited in or computed by PubChem | |
Molecular Formula |
C24H21NO5 | |
Source | PubChem | |
URL | https://pubchem.ncbi.nlm.nih.gov | |
Description | Data deposited in or computed by PubChem | |
DSSTOX Substance ID |
DTXSID20375904 | |
Record name | (3R)-3-({[(9H-Fluoren-9-yl)methoxy]carbonyl}amino)-3-(4-hydroxyphenyl)propanoic acid | |
Source | EPA DSSTox | |
URL | https://comptox.epa.gov/dashboard/DTXSID20375904 | |
Description | DSSTox provides a high quality public chemistry resource for supporting improved predictive toxicology. | |
Molecular Weight |
403.4 g/mol | |
Source | PubChem | |
URL | https://pubchem.ncbi.nlm.nih.gov | |
Description | Data deposited in or computed by PubChem | |
CAS No. |
511272-36-9 | |
Record name | (βR)-β-[[(9H-Fluoren-9-ylmethoxy)carbonyl]amino]-4-hydroxybenzenepropanoic acid | |
Source | CAS Common Chemistry | |
URL | https://commonchemistry.cas.org/detail?cas_rn=511272-36-9 | |
Description | CAS Common Chemistry is an open community resource for accessing chemical information. Nearly 500,000 chemical substances from CAS REGISTRY cover areas of community interest, including common and frequently regulated chemicals, and those relevant to high school and undergraduate chemistry classes. This chemical information, curated by our expert scientists, is provided in alignment with our mission as a division of the American Chemical Society. | |
Explanation | The data from CAS Common Chemistry is provided under a CC-BY-NC 4.0 license, unless otherwise stated. | |
Record name | (3R)-3-({[(9H-Fluoren-9-yl)methoxy]carbonyl}amino)-3-(4-hydroxyphenyl)propanoic acid | |
Source | EPA DSSTox | |
URL | https://comptox.epa.gov/dashboard/DTXSID20375904 | |
Description | DSSTox provides a high quality public chemistry resource for supporting improved predictive toxicology. | |
Haftungsausschluss und Informationen zu In-Vitro-Forschungsprodukten
Bitte beachten Sie, dass alle Artikel und Produktinformationen, die auf BenchChem präsentiert werden, ausschließlich zu Informationszwecken bestimmt sind. Die auf BenchChem zum Kauf angebotenen Produkte sind speziell für In-vitro-Studien konzipiert, die außerhalb lebender Organismen durchgeführt werden. In-vitro-Studien, abgeleitet von dem lateinischen Begriff "in Glas", beinhalten Experimente, die in kontrollierten Laborumgebungen unter Verwendung von Zellen oder Geweben durchgeführt werden. Es ist wichtig zu beachten, dass diese Produkte nicht als Arzneimittel oder Medikamente eingestuft sind und keine Zulassung der FDA für die Vorbeugung, Behandlung oder Heilung von medizinischen Zuständen, Beschwerden oder Krankheiten erhalten haben. Wir müssen betonen, dass jede Form der körperlichen Einführung dieser Produkte in Menschen oder Tiere gesetzlich strikt untersagt ist. Es ist unerlässlich, sich an diese Richtlinien zu halten, um die Einhaltung rechtlicher und ethischer Standards in Forschung und Experiment zu gewährleisten.