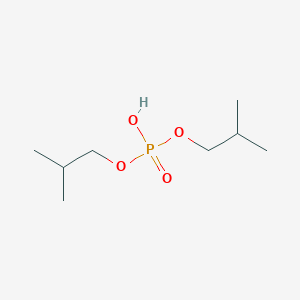
Diisobutyl hydrogen phosphate
- Klicken Sie auf QUICK INQUIRY, um ein Angebot von unserem Expertenteam zu erhalten.
- Mit qualitativ hochwertigen Produkten zu einem WETTBEWERBSFÄHIGEN Preis können Sie sich mehr auf Ihre Forschung konzentrieren.
Übersicht
Beschreibung
Kynurensäure-Natriumsalz ist ein Natriumsalzderivat der Kynurensäure, einem Metaboliten der Aminosäure L-Tryptophan. Kynurensäure ist bekannt für ihre neuroaktiven Eigenschaften und wirkt als Antagonist an exzitatorischen Aminosäure-Rezeptoren. Diese Verbindung wurde wegen ihrer potenziellen therapeutischen Anwendungen bei verschiedenen neurobiologischen Störungen aufgrund ihrer neuroprotektiven und antikonvulsiven Eigenschaften untersucht .
Herstellungsmethoden
Synthetische Routen und Reaktionsbedingungen
Kynurensäure-Natriumsalz kann durch Neutralisation von Kynurensäure mit Natriumhydroxid synthetisiert werden. Die Reaktion beinhaltet das Auflösen von Kynurensäure in Wasser und das Hinzufügen von Natriumhydroxid zur Bildung des Natriumsalzes. Die Lösung wird dann eingedampft, um das feste Kynurensäure-Natriumsalz zu erhalten .
Industrielle Produktionsmethoden
Die industrielle Produktion von Kynurensäure-Natriumsalz folgt einem ähnlichen Prozess, jedoch in größerem Maßstab. Die Reaktionsbedingungen werden optimiert, um eine hohe Ausbeute und Reinheit zu gewährleisten. Der Prozess beinhaltet eine präzise Kontrolle von Temperatur, pH-Wert und Konzentration der Reaktanten, um eine konstante Produktqualität zu erreichen .
Chemische Reaktionsanalyse
Reaktionstypen
Kynurensäure-Natriumsalz unterliegt verschiedenen chemischen Reaktionen, darunter:
Oxidation: Kynurensäure kann zu Chinolinsäure oxidiert werden.
Reduktion: Reduktionsreaktionen können Kynurensäure in ihren entsprechenden Alkohol umwandeln.
Substitution: Substitutionsreaktionen können an der Carboxylgruppe stattfinden und zur Bildung von Estern oder Amiden führen.
Häufige Reagenzien und Bedingungen
Oxidation: Häufige Oxidationsmittel sind Kaliumpermanganat und Wasserstoffperoxid.
Reduktion: Reduktionsmittel wie Natriumborhydrid oder Lithiumaluminiumhydrid werden verwendet.
Substitution: Reagenzien wie Alkohole oder Amine werden unter sauren oder basischen Bedingungen verwendet, um Substitutionsreaktionen zu ermöglichen.
Hauptprodukte, die gebildet werden
Oxidation: Chinolinsäure
Reduktion: Kynurensäurealkohol
Substitution: Ester oder Amide der Kynurensäure
Vorbereitungsmethoden
Synthetic Routes and Reaction Conditions
Kynurenic acid sodium salt can be synthesized by neutralizing kynurenic acid with sodium hydroxide. The reaction involves dissolving kynurenic acid in water and adding sodium hydroxide to form the sodium salt. The solution is then evaporated to obtain the solid kynurenic acid sodium salt .
Industrial Production Methods
Industrial production of kynurenic acid sodium salt follows a similar process but on a larger scale. The reaction conditions are optimized to ensure high yield and purity. The process involves precise control of temperature, pH, and concentration of reactants to achieve consistent product quality .
Analyse Chemischer Reaktionen
Types of Reactions
Kynurenic acid sodium salt undergoes various chemical reactions, including:
Oxidation: Kynurenic acid can be oxidized to form quinolinic acid.
Reduction: Reduction reactions can convert kynurenic acid to its corresponding alcohol.
Substitution: Substitution reactions can occur at the carboxyl group, leading to the formation of esters or amides.
Common Reagents and Conditions
Oxidation: Common oxidizing agents include potassium permanganate and hydrogen peroxide.
Reduction: Reducing agents such as sodium borohydride or lithium aluminum hydride are used.
Substitution: Reagents like alcohols or amines are used under acidic or basic conditions to facilitate substitution reactions.
Major Products Formed
Oxidation: Quinolinic acid
Reduction: Kynurenic alcohol
Substitution: Esters or amides of kynurenic acid
Wissenschaftliche Forschungsanwendungen
Kynurensäure-Natriumsalz hat eine breite Palette von Anwendungen in der wissenschaftlichen Forschung:
Chemie: Wird als Reagenz in verschiedenen chemischen Reaktionen und Studien verwendet.
Biologie: Wird auf seine Rolle im Kynurenin-Stoffwechselweg und seine Auswirkungen auf den Zellstoffwechsel untersucht.
Medizin: Wird wegen seiner neuroprotektiven Eigenschaften und potenziellen therapeutischen Anwendungen bei neurodegenerativen Erkrankungen, Epilepsie und Schizophrenie untersucht.
Industrie: Wird bei der Entwicklung von Pharmazeutika und als Forschungswerkzeug in der Medikamentenforschung verwendet.
Wirkmechanismus
Kynurensäure-Natriumsalz übt seine Wirkungen hauptsächlich durch seine Wirkung als Antagonist an ionotropen Glutamatrezeptoren aus, darunter AMPA-, NMDA- und Kainatrezeptoren. Es wirkt auch als nichtkompetitiver Antagonist an der Glycinstelle des NMDA-Rezeptors und als Antagonist des α7-nikotinergen Acetylcholinrezeptors. Zusätzlich dient es als Ligand für den Orphan-G-Protein-gekoppelten Rezeptor GPR35 und als Agonist für den G-Protein-gekoppelten Rezeptor HCAR3 .
Wirkmechanismus
Kynurenic acid sodium salt exerts its effects primarily through its action as an antagonist at ionotropic glutamate receptors, including AMPA, NMDA, and kainate receptors. It also acts as a noncompetitive antagonist at the glycine site of the NMDA receptor and as an antagonist of the α7 nicotinic acetylcholine receptor. Additionally, it serves as a ligand for the orphan G protein-coupled receptor GPR35 and an agonist for the G protein-coupled receptor HCAR3 .
Vergleich Mit ähnlichen Verbindungen
Ähnliche Verbindungen
Chinolinsäure: Ein weiterer Metabolit des Kynurenin-Stoffwechselwegs, bekannt für seine neurotoxischen Eigenschaften.
Xanthurensäure: Ein Metabolit mit ähnlichen strukturellen Merkmalen, aber unterschiedlichen biologischen Aktivitäten.
Anthranilsäure: Ein Vorläufer im Kynurenin-Stoffwechselweg mit unterschiedlichen chemischen Eigenschaften
Einzigartigkeit
Kynurensäure-Natriumsalz ist einzigartig aufgrund seines breiten Spektrums an antagonistischen Aktivitäten an exzitatorischen Aminosäure-Rezeptoren und seinen neuroprotektiven Eigenschaften. Im Gegensatz zu Chinolinsäure, die neurotoxisch ist, bietet Kynurensäure-Natriumsalz potenzielle therapeutische Vorteile in der Neuroprotektion und antikonvulsiven Aktivität .
Eigenschaften
CAS-Nummer |
6303-30-6 |
---|---|
Molekularformel |
C8H19O4P |
Molekulargewicht |
210.21 g/mol |
IUPAC-Name |
bis(2-methylpropyl) hydrogen phosphate |
InChI |
InChI=1S/C8H19O4P/c1-7(2)5-11-13(9,10)12-6-8(3)4/h7-8H,5-6H2,1-4H3,(H,9,10) |
InChI-Schlüssel |
PVQVJLCMPNEFPM-UHFFFAOYSA-N |
SMILES |
CC(C)COP(=O)(O)OCC(C)C |
Kanonische SMILES |
CC(C)COP(=O)(O)OCC(C)C |
Key on ui other cas no. |
6303-30-6 |
Piktogramme |
Corrosive |
Synonyme |
Phosphoric Acid Bis(2-methylpropyl) Ester; Phosphoric Acid Diisobutyl Ester; _x000B_NSC 41918 |
Herkunft des Produkts |
United States |
Haftungsausschluss und Informationen zu In-Vitro-Forschungsprodukten
Bitte beachten Sie, dass alle Artikel und Produktinformationen, die auf BenchChem präsentiert werden, ausschließlich zu Informationszwecken bestimmt sind. Die auf BenchChem zum Kauf angebotenen Produkte sind speziell für In-vitro-Studien konzipiert, die außerhalb lebender Organismen durchgeführt werden. In-vitro-Studien, abgeleitet von dem lateinischen Begriff "in Glas", beinhalten Experimente, die in kontrollierten Laborumgebungen unter Verwendung von Zellen oder Geweben durchgeführt werden. Es ist wichtig zu beachten, dass diese Produkte nicht als Arzneimittel oder Medikamente eingestuft sind und keine Zulassung der FDA für die Vorbeugung, Behandlung oder Heilung von medizinischen Zuständen, Beschwerden oder Krankheiten erhalten haben. Wir müssen betonen, dass jede Form der körperlichen Einführung dieser Produkte in Menschen oder Tiere gesetzlich strikt untersagt ist. Es ist unerlässlich, sich an diese Richtlinien zu halten, um die Einhaltung rechtlicher und ethischer Standards in Forschung und Experiment zu gewährleisten.