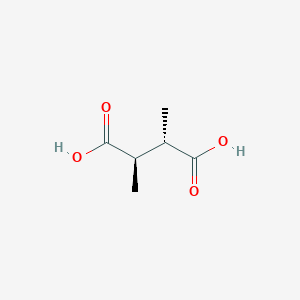
meso-2,3-Dimethylbernsteinsäure
Übersicht
Beschreibung
trans-Oxyresveratrol: (systematischer Name: 4-[(E)-2-(3,5-Dihydroxyphenyl)-ethenyl]benzol-1,3-diol) ist eine natürlich vorkommende Stilbenverbindung. Es ist bekannt für seine einfache chemische Struktur und seine vielfältigen therapeutischen Potenziale. trans-Oxyresveratrol findet sich in verschiedenen Pflanzen, darunter Maulbeerbaum (Morus alba L.), und wurde ausgiebig auf seine biologischen und pharmakologischen Aktivitäten untersucht .
Wissenschaftliche Forschungsanwendungen
Wirkmechanismus
Target of Action
Meso-2,3-Dimethylsuccinic acid, also known as DMSA, is primarily used as a chelating agent . It binds with high specificity to ions of heavy metals such as lead, mercury, cadmium, and arsenic in the blood .
Mode of Action
DMSA forms a water-soluble complex with these heavy metal ions . This complex formation results in the neutralization of the heavy metals, preventing them from causing further harm to the body .
Biochemical Pathways
The primary biochemical pathway affected by DMSA is the body’s detoxification process. By binding to heavy metals, DMSA facilitates their removal from the body, primarily through urinary excretion . This helps to reduce the concentration of these toxic substances in the body, thereby mitigating their harmful effects.
Pharmacokinetics
It is known that dmsa is rapidly and extensively metabolized .
Result of Action
The primary result of DMSA’s action is the reduction of heavy metal toxicity in the body. By chelating these metals and facilitating their excretion, DMSA helps to mitigate the harmful effects of heavy metal poisoning .
Action Environment
The efficacy and stability of DMSA can be influenced by various environmental factors. For instance, the pH of the solution can affect the release of sulfur from DMSA, which is crucial for its chelating action . Additionally, the reaction temperature can also influence this process
Biochemische Analyse
Biochemical Properties
Meso-2,3-Dimethylsuccinic acid plays a role in biochemical reactions, particularly in the context of nanoparticle functionalization . It is often used to improve the colloidal stability or biocompatibility of nanoparticles
Cellular Effects
It is known that it can influence cell function by interacting with certain cellular components .
Molecular Mechanism
The molecular mechanism of action of meso-2,3-Dimethylsuccinic acid involves its interaction with biomolecules at the molecular level. It can bind to certain molecules, potentially influencing enzyme activity and gene expression .
Temporal Effects in Laboratory Settings
In laboratory settings, the effects of meso-2,3-Dimethylsuccinic acid can change over time. This includes its stability, degradation, and long-term effects on cellular function .
Metabolic Pathways
Meso-2,3-Dimethylsuccinic acid is involved in certain metabolic pathways. It interacts with various enzymes and cofactors, and can influence metabolic flux or metabolite levels .
Transport and Distribution
Meso-2,3-Dimethylsuccinic acid is transported and distributed within cells and tissues. It interacts with certain transporters or binding proteins, which can affect its localization or accumulation .
Subcellular Localization
It is known that it can interact with certain cellular components .
Vorbereitungsmethoden
Synthetische Wege und Reaktionsbedingungen:
Perkin-Kondensationsreaktion: Einer der synthetischen Wege für trans-Oxyresveratrol beinhaltet die Perkin-Kondensationsreaktion von 3,5-Dimethoxyphenylessigsäure mit einem entsprechenden substituierten Phenylaldehyd.
Mikrobielle Biosynthese: Ein weiteres Verfahren beinhaltet die Biosynthese von Resveratrol unter Verwendung von metabolisch verändertem Escherichia coli. Dieses Verfahren umfasst die Manipulation von Genen im Shikimat-Weg von E.
Industrielle Produktionsmethoden: Die industrielle Produktion von trans-Oxyresveratrol kann durch Extraktion aus pflanzlichen Materialien unter Verwendung konventioneller Methoden erreicht werden. Es gibt verschiedene Systeme für die qualitative und quantitative Analyse des trans-Oxyresveratrol-Gehalts in pflanzlichen Materialien und Pflanzenprodukten .
Chemische Reaktionsanalyse
Arten von Reaktionen:
Oxidation: trans-Oxyresveratrol unterliegt Oxidationsreaktionen, die zur Bildung verschiedener oxidierter Produkte führen können.
Reduktion: Reduktionsreaktionen können trans-Oxyresveratrol in seine reduzierten Formen umwandeln.
Substitution: Substitutionsreaktionen beinhalten den Austausch einer funktionellen Gruppe durch eine andere.
Gängige Reagenzien und Bedingungen:
Oxidationsmittel: Häufige Oxidationsmittel sind Wasserstoffperoxid und Kaliumpermanganat.
Reduktionsmittel: Natriumborhydrid und Lithiumaluminiumhydrid sind häufig verwendete Reduktionsmittel.
Katalysatoren: Verschiedene Katalysatoren, wie z. B. Palladium auf Kohlenstoff, können verwendet werden, um diese Reaktionen zu erleichtern.
Hauptprodukte: Die Hauptprodukte, die aus diesen Reaktionen gebildet werden, hängen von den spezifischen verwendeten Reagenzien und Bedingungen ab. So kann die Oxidation zur Bildung von Chinonen führen, während die Reduktion Dihydroxyderivate produzieren kann .
Wissenschaftliche Forschungsanwendungen
trans-Oxyresveratrol hat eine breite Palette von wissenschaftlichen Forschungsanwendungen, darunter, aber nicht beschränkt auf:
Chemie: Es wird als Vorläufer bei der Synthese verschiedener chemischer Verbindungen verwendet.
Biologie: trans-Oxyresveratrol zeigt antioxidative, entzündungshemmende und neuroprotektive Aktivitäten.
Wirkmechanismus
Der Wirkmechanismus von trans-Oxyresveratrol beinhaltet mehrere molekulare Ziele und Signalwege:
Antioxidative Aktivität: trans-Oxyresveratrol wirkt als starkes Antioxidans und Radikalfänger.
Entzündungshemmende Aktivität: Die Verbindung reduziert die Produktion von proinflammatorischen Zytokinen und hemmt die Aktivierung von Entzündungswegen.
Neuroprotektive Aktivität: trans-Oxyresveratrol schützt Neuronen vor oxidativem Stress und Apoptose durch Modulation verschiedener Signalwege, darunter der AMPK/ULK1/mTOR-vermittelten Autophie-Weg.
Analyse Chemischer Reaktionen
Types of Reactions:
Oxidation: trans-Oxyresveratrol undergoes oxidation reactions, which can lead to the formation of various oxidized products.
Reduction: Reduction reactions can convert trans-Oxyresveratrol into its reduced forms.
Substitution: Substitution reactions involve the replacement of one functional group with another.
Common Reagents and Conditions:
Oxidizing Agents: Common oxidizing agents include hydrogen peroxide and potassium permanganate.
Reducing Agents: Sodium borohydride and lithium aluminum hydride are commonly used reducing agents.
Catalysts: Various catalysts, such as palladium on carbon, can be used to facilitate these reactions.
Major Products: The major products formed from these reactions depend on the specific reagents and conditions used. For example, oxidation can lead to the formation of quinones, while reduction can produce dihydroxy derivatives .
Vergleich Mit ähnlichen Verbindungen
trans-Oxyresveratrol ist strukturell ähnlich anderen Stilbenverbindungen wie Resveratrol und Gnetol. Es hat einzigartige Eigenschaften, die es von diesen Verbindungen unterscheiden:
Gnetol: Gnetol ist eine weitere Stilbenverbindung mit einer ähnlichen Struktur wie trans-Oxyresveratrol.
Liste ähnlicher Verbindungen:
- Resveratrol
- Gnetol
- Piceatannol
- Pterostilben
Zusammenfassend lässt sich sagen, dass trans-Oxyresveratrol eine vielseitige Verbindung mit einem erheblichen Potenzial in verschiedenen wissenschaftlichen Forschungsanwendungen ist. Seine einzigartige chemische Struktur und seine vielfältigen biologischen Aktivitäten machen es zu einer wertvollen Verbindung für weitere Studien und Entwicklungen.
Biologische Aktivität
Meso-2,3-Dimethylsuccinic acid (DMSA) is an alpha,omega-dicarboxylic acid with significant potential in various biological applications. This compound has garnered attention due to its unique structural properties and biological activities, particularly in the fields of toxicology and drug delivery. This article explores the biological activity of DMSA, emphasizing its mechanisms of action, applications in chelation therapy, and implications in environmental stability.
Chemical Structure and Properties
Meso-2,3-Dimethylsuccinic acid is characterized by two methyl groups at the 2 and 3 positions of the succinic acid backbone. Its chemical formula is and it possesses two carboxylic acid functional groups that contribute to its chelating properties.
Chelation Therapy
DMSA is primarily known for its role as a chelating agent. It has been extensively studied for its efficacy in treating heavy metal poisoning, particularly lead and cadmium toxicity. The compound facilitates the mobilization of heavy metals from biological tissues, thereby enhancing their excretion.
- Lead Poisoning Treatment : A comparative study indicated that DMSA can be administered orally with minimal toxicity while being as effective as traditional parenteral agents like ethylene diamine tetraacetic acid (EDTA) for lead detoxification . In a clinical case, DMSA was shown to reduce blood lead levels effectively while allowing for easier administration compared to EDTA.
- Cadmium Mobilization : Research highlighted that meso-2,3-dimercaptosuccinic acid mono-N-alkylamides derived from DMSA demonstrated significant cadmium mobilizing efficacy in vivo. In experiments with cadmium-loaded rats, certain derivatives of DMSA were more effective than the standard drug sodium N-benzyl-D-glucamine-N-carbodithioate (BGDTC) in reducing hepatic cadmium levels .
Biodegradability and Environmental Impact
The biodegradability of DMSA has been assessed to understand its environmental stability. Studies indicate that both free and nanoparticle-bound forms of DMSA exhibit low biodegradation rates (below 10%) when subjected to microbial communities from wastewater treatment plants. This suggests that DMSA may persist in the environment longer than predicted by quantitative structure–activity relationship models .
The biological activity of DMSA is largely attributed to its ability to form stable complexes with metal ions through its carboxylate and thiol groups. These interactions not only facilitate the detoxification process but also influence the compound's pharmacokinetics.
Table 1: Comparative Efficacy of DMSA Derivatives in Cadmium Mobilization
Compound | Dosage (mmol/kg) | Hepatic Cadmium Reduction (%) | Renal Cadmium Reduction (%) |
---|---|---|---|
Mi-PDMA | 4 x 1.5 | 88% | 30% |
Mi-BDMA | 4 x 1.5 | 85% | 25% |
Mi-ADMA | 4 x 1.5 | 70% | 20% |
BGDTC | 4 x 1.5 | 50% | 13% |
Case Studies
- Lead Poisoning : A clinical trial documented the effectiveness of oral DMSA in children with elevated blood lead levels. The results showed a significant decrease in blood lead concentration over a treatment period, highlighting DMSA's safety profile compared to traditional chelators .
- Cadmium Toxicity : In animal studies, various formulations of DMSA were tested for their ability to mobilize cadmium from liver and kidney tissues. The findings demonstrated that certain derivatives could achieve superior mobilization compared to standard treatments, suggesting potential for improved therapeutic strategies .
Eigenschaften
IUPAC Name |
(2R,3S)-2,3-dimethylbutanedioic acid | |
---|---|---|
Source | PubChem | |
URL | https://pubchem.ncbi.nlm.nih.gov | |
Description | Data deposited in or computed by PubChem | |
InChI |
InChI=1S/C6H10O4/c1-3(5(7)8)4(2)6(9)10/h3-4H,1-2H3,(H,7,8)(H,9,10)/t3-,4+ | |
Source | PubChem | |
URL | https://pubchem.ncbi.nlm.nih.gov | |
Description | Data deposited in or computed by PubChem | |
InChI Key |
KLZYRCVPDWTZLH-ZXZARUISSA-N | |
Source | PubChem | |
URL | https://pubchem.ncbi.nlm.nih.gov | |
Description | Data deposited in or computed by PubChem | |
Canonical SMILES |
CC(C(C)C(=O)O)C(=O)O | |
Source | PubChem | |
URL | https://pubchem.ncbi.nlm.nih.gov | |
Description | Data deposited in or computed by PubChem | |
Isomeric SMILES |
C[C@H]([C@H](C)C(=O)O)C(=O)O | |
Source | PubChem | |
URL | https://pubchem.ncbi.nlm.nih.gov | |
Description | Data deposited in or computed by PubChem | |
Molecular Formula |
C6H10O4 | |
Source | PubChem | |
URL | https://pubchem.ncbi.nlm.nih.gov | |
Description | Data deposited in or computed by PubChem | |
DSSTOX Substance ID |
DTXSID601016985 | |
Record name | rel-(2R,3S)-2,3-Dimethylbutanedioic acid | |
Source | EPA DSSTox | |
URL | https://comptox.epa.gov/dashboard/DTXSID601016985 | |
Description | DSSTox provides a high quality public chemistry resource for supporting improved predictive toxicology. | |
Molecular Weight |
146.14 g/mol | |
Source | PubChem | |
URL | https://pubchem.ncbi.nlm.nih.gov | |
Description | Data deposited in or computed by PubChem | |
CAS No. |
608-40-2 | |
Record name | rel-(2R,3S)-2,3-Dimethylbutanedioic acid | |
Source | CAS Common Chemistry | |
URL | https://commonchemistry.cas.org/detail?cas_rn=608-40-2 | |
Description | CAS Common Chemistry is an open community resource for accessing chemical information. Nearly 500,000 chemical substances from CAS REGISTRY cover areas of community interest, including common and frequently regulated chemicals, and those relevant to high school and undergraduate chemistry classes. This chemical information, curated by our expert scientists, is provided in alignment with our mission as a division of the American Chemical Society. | |
Explanation | The data from CAS Common Chemistry is provided under a CC-BY-NC 4.0 license, unless otherwise stated. | |
Record name | rel-(2R,3S)-2,3-Dimethylbutanedioic acid | |
Source | EPA DSSTox | |
URL | https://comptox.epa.gov/dashboard/DTXSID601016985 | |
Description | DSSTox provides a high quality public chemistry resource for supporting improved predictive toxicology. | |
Record name | (R*,S*)-2,3-dimethylsuccinic acid | |
Source | European Chemicals Agency (ECHA) | |
URL | https://echa.europa.eu/substance-information/-/substanceinfo/100.009.240 | |
Description | The European Chemicals Agency (ECHA) is an agency of the European Union which is the driving force among regulatory authorities in implementing the EU's groundbreaking chemicals legislation for the benefit of human health and the environment as well as for innovation and competitiveness. | |
Explanation | Use of the information, documents and data from the ECHA website is subject to the terms and conditions of this Legal Notice, and subject to other binding limitations provided for under applicable law, the information, documents and data made available on the ECHA website may be reproduced, distributed and/or used, totally or in part, for non-commercial purposes provided that ECHA is acknowledged as the source: "Source: European Chemicals Agency, http://echa.europa.eu/". Such acknowledgement must be included in each copy of the material. ECHA permits and encourages organisations and individuals to create links to the ECHA website under the following cumulative conditions: Links can only be made to webpages that provide a link to the Legal Notice page. | |
Retrosynthesis Analysis
AI-Powered Synthesis Planning: Our tool employs the Template_relevance Pistachio, Template_relevance Bkms_metabolic, Template_relevance Pistachio_ringbreaker, Template_relevance Reaxys, Template_relevance Reaxys_biocatalysis model, leveraging a vast database of chemical reactions to predict feasible synthetic routes.
One-Step Synthesis Focus: Specifically designed for one-step synthesis, it provides concise and direct routes for your target compounds, streamlining the synthesis process.
Accurate Predictions: Utilizing the extensive PISTACHIO, BKMS_METABOLIC, PISTACHIO_RINGBREAKER, REAXYS, REAXYS_BIOCATALYSIS database, our tool offers high-accuracy predictions, reflecting the latest in chemical research and data.
Strategy Settings
Precursor scoring | Relevance Heuristic |
---|---|
Min. plausibility | 0.01 |
Model | Template_relevance |
Template Set | Pistachio/Bkms_metabolic/Pistachio_ringbreaker/Reaxys/Reaxys_biocatalysis |
Top-N result to add to graph | 6 |
Feasible Synthetic Routes
Q1: How does meso-2,3-dimethylsuccinic acid interact with metal surfaces, and does this differ from its chiral counterpart?
A1: Research indicates that both meso-2,3-dimethylsuccinic acid and its racemic mixture (containing both chiral enantiomers) form ordered structures on a Cu(110) surface. [] Interestingly, despite being achiral, the meso form also creates enantiomorphous structures on the copper surface, coexisting with structures that retain the substrate's mirror symmetry. [] This suggests that the methyl groups influence the adsorption geometry, leading to chiral expressions on the surface even for the achiral molecule. The study also found a significant difference in the thermal decomposition behavior between the meso and racemic forms, indicating a distinct influence of chirality on this process. []
Q2: Can you provide an example of how meso-2,3-dimethylsuccinic acid is used in organic synthesis?
A2: meso-2,3-Dimethylsuccinic acid serves as a valuable starting material for constructing complex molecules. For example, it was employed in the synthesis of (±)-faranal, a naturally occurring insect pheromone. [] The meso configuration of the acid was strategically used to introduce two erythro-configured methyl groups into the target molecule, showcasing its utility in stereoselective synthesis. []
Haftungsausschluss und Informationen zu In-Vitro-Forschungsprodukten
Bitte beachten Sie, dass alle Artikel und Produktinformationen, die auf BenchChem präsentiert werden, ausschließlich zu Informationszwecken bestimmt sind. Die auf BenchChem zum Kauf angebotenen Produkte sind speziell für In-vitro-Studien konzipiert, die außerhalb lebender Organismen durchgeführt werden. In-vitro-Studien, abgeleitet von dem lateinischen Begriff "in Glas", beinhalten Experimente, die in kontrollierten Laborumgebungen unter Verwendung von Zellen oder Geweben durchgeführt werden. Es ist wichtig zu beachten, dass diese Produkte nicht als Arzneimittel oder Medikamente eingestuft sind und keine Zulassung der FDA für die Vorbeugung, Behandlung oder Heilung von medizinischen Zuständen, Beschwerden oder Krankheiten erhalten haben. Wir müssen betonen, dass jede Form der körperlichen Einführung dieser Produkte in Menschen oder Tiere gesetzlich strikt untersagt ist. Es ist unerlässlich, sich an diese Richtlinien zu halten, um die Einhaltung rechtlicher und ethischer Standards in Forschung und Experiment zu gewährleisten.