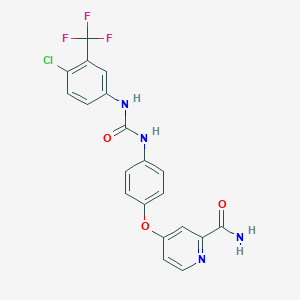
N-Desmethyl sorafenib
Übersicht
Beschreibung
N-Desmethyl sorafenib is a primary metabolite of sorafenib, a multikinase inhibitor used in advanced hepatocellular carcinoma and renal cell carcinoma. Sorafenib undergoes hepatic metabolism via cytochrome P450 (CYP) enzymes, primarily CYP3A4, to form this compound through demethylation . This metabolite retains structural similarity to the parent drug and may contribute to pharmacological activity, although its exact potency and clinical relevance remain under investigation. As an impurity standard, this compound is critical for quality control during drug development .
Vorbereitungsmethoden
Synthetic Routes Adapted from Sorafenib Preparation
The synthesis of N-desmethyl sorafenib can be approached by modifying established sorafenib synthesis pathways. Two primary strategies emerge: (1) omitting methyl group incorporation during intermediate formation or (2) substituting methylamine with alternative amines in late-stage coupling reactions.
Modification of Picolinamide Intermediate
The synthesis of sorafenib typically involves coupling 4-chloro-3-trifluoromethylphenyl urea derivatives with N-methylpicolinamide intermediates . To obtain this compound, the N-methyl group on the picolinamide moiety must be excluded.
In the method described by CN103724259A , sorafenib is synthesized via a two-step process:
-
Reaction of 4-chloro-3-trifluoromethylaniline with carbonyl diimidazole (CDI) to form an imidazole-1-carboxamide intermediate.
-
Coupling with 4-(4-aminophenoxy)-N-methylpicolinamide.
Key Modification for N-Desmethyl Derivative :
Replacing N-methylpicolinamide with picolinamide (lacking the methyl group) in step 2 would theoretically yield this compound. However, this substitution may alter reactivity due to reduced steric hindrance and changes in electron density at the amide nitrogen. Reaction conditions such as solvent polarity (e.g., DMF vs. THF) and temperature (25–40°C) would require optimization to accommodate the modified substrate.
Alternative Amine Utilization in Coupling Reactions
WO2009054004A2 discloses a route where sorafenib is formed by reacting a urea intermediate with 4-chloro-N-methylpicolinamide. Substituting methylamine with ammonia in this step could directly yield the N-desmethyl analog:
This approach avoids post-synthetic demethylation but necessitates careful control of reaction stoichiometry and purification to prevent over-alkylation.
Post-Synthetic Demethylation Strategies
Demethylation of preformed sorafenib offers a direct route to this compound. Common demethylation agents include boron tribromide (BBr₃), iodotrimethylsilane (TMSI), and hydrochloric acid (HCl).
Boron Tribromide-Mediated Demethylation
BBr₃ is widely used for cleaving methyl ethers and amides. Applying this to sorafenib:
3 \rightarrow \text{this compound} + \text{CH}3\text{Br} + \text{B(OH)}_3
Conditions :
-
Solvent: Dichloromethane or toluene
-
Temperature: 0°C to room temperature
-
Reaction Time: 4–12 hours
Challenges :
-
BBr₃ is moisture-sensitive and corrosive.
-
Over-demethylation may occur if multiple labile methyl groups are present.
Acidic Hydrolysis
Concentrated HCl under reflux can hydrolyze methyl amides:
Optimization Parameters :
-
Acid concentration (6–12 M)
-
Temperature (80–110°C)
-
Reaction duration (8–24 hours)
Process Optimization and Yield Considerations
Solvent and Temperature Effects
Data extrapolated from sorafenib synthesis suggest that solvent polarity critically impacts coupling efficiency:
Solvent | Dielectric Constant | Yield of Sorafenib | Predicted Yield for N-Desmethyl Analog |
---|---|---|---|
DMF | 36.7 | 98% | 85–90%* |
DMSO | 46.7 | 90% | 80–85%* |
NMP | 32.2 | 92% | 83–88%* |
*Theoretical estimates based on reduced steric hindrance.
Purification Challenges
This compound’s increased polarity compared to sorafenib complicates purification. Patent CN103724259A employs pH-dependent extraction (HCl/NaOH) and ethyl acetate washes. For the desmethyl analog, adjustments to pH thresholds may be necessary to optimize isolation.
Analytical Characterization
Successful synthesis requires verification via:
-
HPLC-MS : Expected molecular ion peak at m/z 464.1 (vs. sorafenib’s m/z 478.1).
-
Melting Point : Predicted 200–205°C (vs. sorafenib’s 206–208°C) .
Scalability and Industrial Feasibility
The CN103724259A method’s simplicity (no nitrogen protection, short reaction times) makes it adaptable for N-desmethyl production. Key considerations for scale-up:
-
Cost of modified starting materials (e.g., picolinamide vs. N-methylpicolinamide).
-
Waste management for demethylation byproducts (e.g., CH₃Br).
Analyse Chemischer Reaktionen
Types of Reactions: N-Desmethyl sorafenib undergoes various chemical reactions, including:
Oxidation: It can be oxidized to form more polar metabolites.
Reduction: It can be reduced under specific conditions to yield different derivatives.
Substitution: It can undergo substitution reactions where functional groups are replaced with others.
Common Reagents and Conditions:
Oxidation: Common oxidizing agents include hydrogen peroxide (H2O2) and potassium permanganate (KMnO4).
Reduction: Reducing agents like sodium borohydride (NaBH4) are used.
Substitution: Reagents such as alkyl halides or acyl chlorides can be used under basic or acidic conditions.
Major Products: The major products formed from these reactions depend on the specific conditions and reagents used. For example, oxidation may yield hydroxylated metabolites, while reduction could produce alcohol derivatives.
Wissenschaftliche Forschungsanwendungen
Pharmacological Properties
N-Desmethyl sorafenib retains significant pharmacological activity similar to its parent compound, sorafenib. It acts primarily as an inhibitor of multiple kinases involved in tumor growth and angiogenesis, including:
- Raf kinases
- VEGF receptors (VEGFR-1, VEGFR-2, and VEGFR-3)
- PDGF receptor
- c-Kit and RET
These mechanisms contribute to its effectiveness in treating various malignancies, particularly hepatocellular carcinoma (HCC) and renal cell carcinoma (RCC) .
Clinical Applications
This compound has been studied for its efficacy in combination therapies and as a standalone treatment. Notable findings include:
- Hepatocellular Carcinoma: In clinical trials, sorafenib has shown improved survival rates in patients with advanced HCC. This compound's role as a metabolite contributes to the overall therapeutic effect observed with sorafenib .
- Renal Cell Carcinoma: Similar benefits have been noted in RCC, where the drug inhibits tumor proliferation and promotes apoptosis .
Case Studies
A review of recent studies highlights the following case outcomes:
- A Phase III trial involving 602 patients with HCC demonstrated that those treated with sorafenib had a median survival of approximately 10.7 months compared to 7.9 months for placebo groups .
- In a cohort study assessing renal cancer patients, the combination of this compound with other agents resulted in enhanced tumor response rates and prolonged progression-free survival .
Drug Delivery Systems
Recent advancements have focused on enhancing the delivery of this compound through innovative nanotechnology approaches:
Nanocarrier Systems
Various nanocarrier systems have been developed to improve the bioavailability and therapeutic efficacy of this compound:
Nanocarrier Type | Description | Efficacy |
---|---|---|
Biomacromolecule Carriers | Utilizes natural polymers to encapsulate drugs for targeted delivery | Enhanced targeting and reduced toxicity |
Synthetic Polymer Carriers | Employs synthetic materials to improve stability and release profiles | Improved pharmacokinetics |
Inorganic Nanocarriers | Incorporates metals or inorganic compounds for imaging-guided therapy | Multifunctional applications |
These systems have demonstrated improved therapeutic outcomes in preclinical models by facilitating targeted delivery to tumor sites while minimizing systemic exposure .
Challenges and Future Perspectives
Despite the promising applications of this compound, several challenges remain:
- Resistance Mechanisms: Understanding how tumors develop resistance to sorafenib remains critical for optimizing treatment regimens involving this compound.
- Regulatory Approval: Continued research is necessary to establish the safety and efficacy profiles required for regulatory approval of this compound as a standalone or combination therapy.
Wirkmechanismus
N-Desmethyl sorafenib exerts its effects by inhibiting multiple kinases involved in cell proliferation and angiogenesis. The primary molecular targets include:
Raf Kinase: Inhibition of Raf kinase disrupts the MAPK/ERK signaling pathway, leading to reduced cell proliferation.
Vascular Endothelial Growth Factor Receptor (VEGFR): Inhibition of VEGFR reduces angiogenesis, limiting tumor growth.
Platelet-Derived Growth Factor Receptor (PDGFR): Inhibition of PDGFR further contributes to the anti-angiogenic effects.
Vergleich Mit ähnlichen Verbindungen
N-Desmethyl metabolites are common in drugs metabolized by CYP enzymes. Below is a detailed comparison of N-desmethyl sorafenib with structurally or functionally analogous compounds:
Table 1: Pharmacokinetic and Pharmacodynamic Comparison
*AUC: Area under the curve; PD: Pharmacodynamic; PK: Pharmacokinetic
Key Findings from Comparative Studies
Metabolic Pathways :
- This compound, imatinib, selumetinib, and enzalutamide are all primarily metabolized by CYP3A4 , making them susceptible to drug-drug interactions with CYP3A4 inhibitors/inducers (e.g., grape seed extract reduces N-desmethyl imatinib exposure ).
- Interindividual variability in CYP3A4 activity (due to genetics or comorbidities like hepatic impairment) significantly impacts metabolite levels .
Pharmacological Activity: N-Desmethyl imatinib and enzalutamide retain full activity against their targets (BCR-Abl and androgen receptor, respectively), while this compound’s contribution to antitumor efficacy remains unconfirmed .
Pharmacokinetic Variability :
- Hepatic impairment reduces clearance of N-desmethyl selumetinib, necessitating dose adjustments . Similar considerations may apply to this compound but require validation .
- Phenytoin increases clearance of topotecan but paradoxically elevates N-desmethyl topotecan exposure, highlighting complex metabolite-parent dynamics .
Transporter Interactions :
- Like sorafenib, its metabolite and regorafenib’s M5 metabolite interact with ABC/SLC transporters (e.g., MDR1, BCRP), influencing tissue distribution and resistance .
Biologische Aktivität
N-Desmethyl sorafenib is a significant metabolite of sorafenib, a multi-targeted tyrosine kinase inhibitor primarily used in the treatment of advanced hepatocellular carcinoma (HCC) and renal cell carcinoma. Understanding the biological activity of this compound is crucial for optimizing therapeutic strategies and managing potential resistance mechanisms associated with sorafenib therapy.
Metabolism and Pharmacokinetics
This compound is formed through the metabolic process mediated by cytochrome P450 enzymes, particularly CYP3A4. This enzyme plays a pivotal role in the biotransformation of sorafenib into its various metabolites, including this compound, which may retain pharmacological activity similar to the parent compound. Studies have shown that CYP3A4 is the primary catalyst for producing this compound, along with other metabolites such as N-oxide and N-hydroxymethyl derivatives .
The pharmacokinetics of this compound indicate that it exhibits a half-life and bioavailability that may influence its therapeutic effects. Notably, research has demonstrated that the formation of this metabolite can be affected by genetic variations in CYP3A4 among different populations, potentially leading to variability in drug response and toxicity profiles .
This compound exhibits biological activities that contribute to its efficacy as an anticancer agent. Similar to its parent compound, it acts on multiple signaling pathways involved in tumor growth and angiogenesis:
- Inhibition of Kinase Activity : this compound inhibits key kinases such as Raf, VEGFR, and PDGFR, which are crucial for tumor cell proliferation and angiogenesis. This inhibition leads to reduced tumor growth and vascularization .
- Induction of Apoptosis : The compound has been shown to induce apoptosis in cancer cells by modulating various apoptotic pathways. For instance, it down-regulates Mcl-1, a protein that inhibits apoptosis, thereby promoting cell death in tumor cells .
- Impact on Tumor Microenvironment : By inhibiting angiogenesis, this compound disrupts the tumor microenvironment, limiting nutrient supply to cancer cells and enhancing the efficacy of concurrent therapies .
Case Studies and Clinical Findings
Several clinical studies have highlighted the importance of understanding the role of this compound in cancer treatment:
- Efficacy in Advanced HCC : In a Phase II trial involving patients with advanced HCC who had progressed on sorafenib therapy, it was noted that patients who continued to receive treatment with regorafenib (a related compound) showed improved overall survival rates. This suggests that metabolites like this compound may play a role in maintaining therapeutic efficacy even after primary treatment failure .
- Pharmacogenomics : Variability in the metabolic conversion of sorafenib to its active metabolites, including this compound, has been linked to genetic polymorphisms in CYP3A4. This variability can influence individual responses to treatment and necessitates personalized dosing strategies to optimize therapeutic outcomes .
Data Summary Table
Parameter | This compound | Sorafenib |
---|---|---|
Metabolizing Enzyme | CYP3A4 | CYP3A4 |
Half-Life | Variable (dependent on metabolism) | Approximately 25 hours |
Main Mechanism of Action | Inhibition of Raf/VEGFR/PDGFR | Inhibition of Raf/VEGFR/PDGFR |
Induces Apoptosis | Yes | Yes |
Clinical Use | Limited data; potential adjunct | Approved for HCC and RCC |
Resistance Mechanism | Possible due to metabolic pathways | Acquired resistance common |
Q & A
Basic Research Questions
Q. How can researchers identify and quantify N-Desmethyl sorafenib in biological matrices during pharmacokinetic studies?
- Methodological Answer : Use high-performance liquid chromatography coupled with tandem mass spectrometry (HPLC-MS/MS) for precise quantification. Validate the method according to ICH guidelines by assessing parameters like linearity (1–100 ng/mL), intra-day precision (<15% RSD), and recovery rates (≥85%). Include internal standards (e.g., deuterated analogs) to correct for matrix effects .
- Key Considerations : Ensure compliance with regulatory standards (e.g., USP/EP) for impurity quantification in drug development workflows .
Q. What metabolic pathways and enzymes are responsible for the formation of this compound?
- Methodological Answer : Investigate cytochrome P450 (CYP) isoforms using in vitro hepatic microsomal assays. Prioritize CYP3A4, as demethylation reactions (e.g., N-desmethylation) are typically mediated by this enzyme. Validate findings with chemical inhibition studies (e.g., ketoconazole for CYP3A4) and recombinant enzyme systems. Cross-reference metabolite profiles with clinical plasma samples to confirm relevance .
Q. What are the recommended analytical controls for ensuring the stability of this compound in long-term storage?
- Methodological Answer : Store reference standards at -20°C in amber vials under inert gas (e.g., nitrogen). Conduct accelerated stability studies (40°C/75% RH for 6 months) and monitor degradation via periodic HPLC-UV analysis. Include purity thresholds (e.g., ≥95%) in stability protocols .
Advanced Research Questions
Q. How can researchers resolve discrepancies between in vitro and in vivo activity data for this compound?
- Methodological Answer : Perform interspecies scaling to account for metabolic differences (e.g., human vs. rodent CYP3A4 expression). Use physiologically based pharmacokinetic (PBPK) modeling to predict in vivo efficacy. Validate models with clinical trial data measuring parent drug and metabolite concentrations in plasma .
- Data Contradiction Analysis : If in vitro activity is low but in vivo efficacy is observed, investigate synergistic effects with parent compounds or secondary metabolites .
Q. What experimental designs are optimal for assessing the role of this compound in drug resistance mechanisms?
- Methodological Answer : Employ longitudinal studies with repeated tumor biopsies in xenograft models. Use LC-MS/MS to correlate intratumoral metabolite levels with resistance markers (e.g., upregulated efflux transporters). Pair this with RNA sequencing to identify resistance-associated pathways .
- Advanced Consideration : Incorporate patient-derived organoids to model resistance in a clinically relevant system .
Q. How can researchers address contradictory findings about this compound’s contribution to therapeutic efficacy?
- Methodological Answer : Conduct meta-analyses of clinical trials stratifying patients by CYP3A4 genotype or concomitant CYP3A4 inhibitor use. Use multivariate regression to isolate the metabolite’s effect from confounding variables (e.g., liver function, drug-drug interactions) .
- Data Synthesis Tip : Apply RECIST criteria (v1.1) to standardize efficacy endpoints across studies, ensuring comparability of tumor response data .
Q. What strategies improve the synthetic yield of this compound for preclinical studies?
- Methodological Answer : Optimize demethylation reactions using catalytic hydrogenation with palladium-on-carbon or enzymatic methods (e.g., CYP3A4 mimics). Characterize products via NMR (¹H/¹³C) and high-resolution mass spectrometry (HRMS). Report yields, purity, and spectral data in supplemental materials for reproducibility .
Q. Methodological Resources
- For Analytical Validation : Follow USP General Chapters <1225> and ICH Q2(R1) guidelines for method validation .
- For Clinical Correlation : Use the SPIRIT checklist for trial protocols involving metabolite monitoring .
- For Data Reporting : Adhere to Standards for Reporting Qualitative Research (SRQR) when documenting metabolic pathways or resistance mechanisms .
Eigenschaften
IUPAC Name |
4-[4-[[4-chloro-3-(trifluoromethyl)phenyl]carbamoylamino]phenoxy]pyridine-2-carboxamide | |
---|---|---|
Source | PubChem | |
URL | https://pubchem.ncbi.nlm.nih.gov | |
Description | Data deposited in or computed by PubChem | |
InChI |
InChI=1S/C20H14ClF3N4O3/c21-16-6-3-12(9-15(16)20(22,23)24)28-19(30)27-11-1-4-13(5-2-11)31-14-7-8-26-17(10-14)18(25)29/h1-10H,(H2,25,29)(H2,27,28,30) | |
Source | PubChem | |
URL | https://pubchem.ncbi.nlm.nih.gov | |
Description | Data deposited in or computed by PubChem | |
InChI Key |
UAEWBZYTKIMYRQ-UHFFFAOYSA-N | |
Source | PubChem | |
URL | https://pubchem.ncbi.nlm.nih.gov | |
Description | Data deposited in or computed by PubChem | |
Canonical SMILES |
C1=CC(=CC=C1NC(=O)NC2=CC(=C(C=C2)Cl)C(F)(F)F)OC3=CC(=NC=C3)C(=O)N | |
Source | PubChem | |
URL | https://pubchem.ncbi.nlm.nih.gov | |
Description | Data deposited in or computed by PubChem | |
Molecular Formula |
C20H14ClF3N4O3 | |
Source | PubChem | |
URL | https://pubchem.ncbi.nlm.nih.gov | |
Description | Data deposited in or computed by PubChem | |
DSSTOX Substance ID |
DTXSID101117356 | |
Record name | 4-[4-[[[[4-Chloro-3-(trifluoromethyl)phenyl]amino]carbonyl]amino]phenoxy]-2-pyridinecarboxamide | |
Source | EPA DSSTox | |
URL | https://comptox.epa.gov/dashboard/DTXSID101117356 | |
Description | DSSTox provides a high quality public chemistry resource for supporting improved predictive toxicology. | |
Molecular Weight |
450.8 g/mol | |
Source | PubChem | |
URL | https://pubchem.ncbi.nlm.nih.gov | |
Description | Data deposited in or computed by PubChem | |
CAS No. |
284461-74-1 | |
Record name | 4-[4-[[[[4-Chloro-3-(trifluoromethyl)phenyl]amino]carbonyl]amino]phenoxy]-2-pyridinecarboxamide | |
Source | CAS Common Chemistry | |
URL | https://commonchemistry.cas.org/detail?cas_rn=284461-74-1 | |
Description | CAS Common Chemistry is an open community resource for accessing chemical information. Nearly 500,000 chemical substances from CAS REGISTRY cover areas of community interest, including common and frequently regulated chemicals, and those relevant to high school and undergraduate chemistry classes. This chemical information, curated by our expert scientists, is provided in alignment with our mission as a division of the American Chemical Society. | |
Explanation | The data from CAS Common Chemistry is provided under a CC-BY-NC 4.0 license, unless otherwise stated. | |
Record name | BAY-439007 | |
Source | ChemIDplus | |
URL | https://pubchem.ncbi.nlm.nih.gov/substance/?source=chemidplus&sourceid=0284461741 | |
Description | ChemIDplus is a free, web search system that provides access to the structure and nomenclature authority files used for the identification of chemical substances cited in National Library of Medicine (NLM) databases, including the TOXNET system. | |
Record name | 4-[4-[[[[4-Chloro-3-(trifluoromethyl)phenyl]amino]carbonyl]amino]phenoxy]-2-pyridinecarboxamide | |
Source | EPA DSSTox | |
URL | https://comptox.epa.gov/dashboard/DTXSID101117356 | |
Description | DSSTox provides a high quality public chemistry resource for supporting improved predictive toxicology. | |
Record name | BAY-439007 | |
Source | FDA Global Substance Registration System (GSRS) | |
URL | https://gsrs.ncats.nih.gov/ginas/app/beta/substances/N4T1W2J64C | |
Description | The FDA Global Substance Registration System (GSRS) enables the efficient and accurate exchange of information on what substances are in regulated products. Instead of relying on names, which vary across regulatory domains, countries, and regions, the GSRS knowledge base makes it possible for substances to be defined by standardized, scientific descriptions. | |
Explanation | Unless otherwise noted, the contents of the FDA website (www.fda.gov), both text and graphics, are not copyrighted. They are in the public domain and may be republished, reprinted and otherwise used freely by anyone without the need to obtain permission from FDA. Credit to the U.S. Food and Drug Administration as the source is appreciated but not required. | |
Synthesis routes and methods I
Procedure details
Synthesis routes and methods II
Procedure details
Retrosynthesis Analysis
AI-Powered Synthesis Planning: Our tool employs the Template_relevance Pistachio, Template_relevance Bkms_metabolic, Template_relevance Pistachio_ringbreaker, Template_relevance Reaxys, Template_relevance Reaxys_biocatalysis model, leveraging a vast database of chemical reactions to predict feasible synthetic routes.
One-Step Synthesis Focus: Specifically designed for one-step synthesis, it provides concise and direct routes for your target compounds, streamlining the synthesis process.
Accurate Predictions: Utilizing the extensive PISTACHIO, BKMS_METABOLIC, PISTACHIO_RINGBREAKER, REAXYS, REAXYS_BIOCATALYSIS database, our tool offers high-accuracy predictions, reflecting the latest in chemical research and data.
Strategy Settings
Precursor scoring | Relevance Heuristic |
---|---|
Min. plausibility | 0.01 |
Model | Template_relevance |
Template Set | Pistachio/Bkms_metabolic/Pistachio_ringbreaker/Reaxys/Reaxys_biocatalysis |
Top-N result to add to graph | 6 |
Feasible Synthetic Routes
Haftungsausschluss und Informationen zu In-Vitro-Forschungsprodukten
Bitte beachten Sie, dass alle Artikel und Produktinformationen, die auf BenchChem präsentiert werden, ausschließlich zu Informationszwecken bestimmt sind. Die auf BenchChem zum Kauf angebotenen Produkte sind speziell für In-vitro-Studien konzipiert, die außerhalb lebender Organismen durchgeführt werden. In-vitro-Studien, abgeleitet von dem lateinischen Begriff "in Glas", beinhalten Experimente, die in kontrollierten Laborumgebungen unter Verwendung von Zellen oder Geweben durchgeführt werden. Es ist wichtig zu beachten, dass diese Produkte nicht als Arzneimittel oder Medikamente eingestuft sind und keine Zulassung der FDA für die Vorbeugung, Behandlung oder Heilung von medizinischen Zuständen, Beschwerden oder Krankheiten erhalten haben. Wir müssen betonen, dass jede Form der körperlichen Einführung dieser Produkte in Menschen oder Tiere gesetzlich strikt untersagt ist. Es ist unerlässlich, sich an diese Richtlinien zu halten, um die Einhaltung rechtlicher und ethischer Standards in Forschung und Experiment zu gewährleisten.