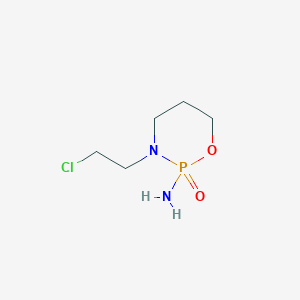
2-Dechloroethylifosfamide
Übersicht
Beschreibung
However, based on nomenclature conventions, it may relate to metabolites of alkylating agents like ifosfamide, which undergo dechloroethylation during metabolism. Such metabolites are critical in oncology, as they influence therapeutic efficacy and toxicity profiles. These analogs provide a basis for inferring properties of 2-Dechloroethylifosfamide, assuming structural similarities .
Vorbereitungsmethoden
Enzymatic Biosynthesis via Cytochrome P450-Mediated N-Dechloroethylation
Metabolic Pathway and Enzyme Specificity
The primary method for generating 2-dechloroethylifosfamide involves the hepatic metabolism of ifosfamide. CYP3A4 and CYP2B6 catalyze the N-dechloroethylation reaction, removing a chloroethyl group from ifosfamide to yield this compound and chloroacetaldehyde.
Key Reaction:
This pathway competes with ifosfamide’s activation to its cytotoxic metabolite, ifosforamide mustard, making enzymatic selectivity critical for modulating therapeutic efficacy and toxicity.
In Vitro Preparation Using Liver Microsomes
In laboratory settings, this compound is often synthesized using liver microsomes or recombinant CYP enzymes.
Typical Protocol:
-
Substrate Preparation : Ifosfamide is dissolved in a buffer (e.g., potassium phosphate, pH 7.4) at concentrations of 0.1–1.0 mM.
-
Enzyme Source : Human liver microsomes or recombinant CYP3A4/2B6 are added (0.5–2.0 mg/mL protein).
-
Cofactors : NADPH (1–2 mM) is included to sustain enzymatic activity.
-
Incubation : Reactions proceed at 37°C for 30–120 minutes, followed by termination via acetonitrile or methanol.
-
Analysis : Metabolites are quantified using LC-MS/MS or HPLC.
Table 1: Optimization Parameters for In Vitro Synthesis
Parameter | Optimal Range | Impact on Yield |
---|---|---|
pH | 7.0–7.6 | Affects enzyme stability |
Temperature | 35–37°C | Higher temperatures denature enzymes |
NADPH Concentration | 1.0–2.0 mM | Essential for redox cycling |
Incubation Time | 60–90 minutes | Longer durations increase conversion |
Influence of Ifosfamide Purity on Metabolite Yield
Crystal Property | Effect on Metabolism |
---|---|
Particle Size | Larger crystals slow dissolution, reducing metabolic rate |
Dust Content | High dust increases variability in enzyme-substrate interactions |
Solubility | Enhanced solubility improves enzymatic access |
Chemoselective Modulation of Metabolic Pathways
Competitive Pathways and Yield Optimization
The balance between N-dechloroethylation (yielding this compound) and 4-hydroxylation (activating ifosfamide) is influenced by:
-
Enzyme Inhibitors/Inducers : Ketoconazole (CYP3A4 inhibitor) reduces this compound formation, while phenobarbital (CYP2B6 inducer) increases it.
-
Stereochemistry : The (S)-enantiomer of ifosfamide undergoes faster N-dechloroethylation than the (R)-enantiomer, affecting metabolite ratios.
Table 3: Strategies to Modulate Metabolite Formation
Strategy | Effect on this compound Yield |
---|---|
CYP3A4 Inhibition | ↓ 50–70% |
CYP2B6 Induction | ↑ 30–50% |
Use of (S)-Ifosfamide | ↑ 20–40% |
Challenges in Industrial-Scale Production
Research-Scale Limitations
-
Low Yields : Typical conversions range from 5–15% in vitro, necessitating large substrate quantities.
-
Byproduct Toxicity : Chloroacetaldehyde, a byproduct, requires careful handling due to nephrotoxic and neurotoxic effects.
Emerging Analytical Techniques for Characterization
LC-MS/MS Quantification
Modern workflows employ tandem mass spectrometry to detect this compound at nanomolar concentrations, enabling precise pharmacokinetic studies.
Typical Parameters:
-
Column : C18 reverse-phase (2.1 × 50 mm, 1.7 µm).
-
Mobile Phase : 0.1% formic acid in water/acetonitrile gradient.
-
Detection : MRM transition m/z 284.1 → 154.1 (this compound).
NMR Spectroscopy
While less common due to sensitivity limitations, -NMR can distinguish this compound from ifosfamide based on phosphorus chemical shifts.
Analyse Chemischer Reaktionen
Types of Reactions: 2-Dechloroethylifosfamide primarily undergoes metabolic reactions rather than chemical reactions in a laboratory setting. The key reaction is the N-dechloroethylation of ifosfamide, which is a metabolic process.
Common Reagents and Conditions: The primary reagents involved in the formation of this compound are the cytochrome P450 enzymes, particularly CYP3A4 and CYP2B6. These enzymes facilitate the removal of the chloroethyl group from ifosfamide.
Major Products Formed: The major product formed from the N-dechloroethylation of ifosfamide is this compound. This metabolite is considered inactive compared to the parent compound ifosfamide, which exerts its therapeutic effects through DNA alkylation.
Wissenschaftliche Forschungsanwendungen
Pharmacokinetics and Metabolism
The pharmacokinetics of 2-DCEIF are essential for understanding its efficacy and safety profile in patients undergoing ifosfamide treatment. Studies have demonstrated that 2-DCEIF is primarily formed through the dealkylation of ifosfamide, a process that can vary significantly between individuals due to genetic factors affecting drug metabolism.
Key Findings:
- Metabolic Pathways: A comparative study highlighted that ifosfamide preferentially undergoes N-dechloroethylation, leading to the production of both 2-DCEIF and 3-dechloroethylifosfamide (3-DCEIF) as major metabolites. This metabolic pathway has been linked to the systemic toxicity observed with ifosfamide administration, particularly neurotoxicity and nephrotoxicity .
- Analytical Methods: Advanced bioanalytical techniques such as gas chromatography with nitrogen-phosphorus detection (GC-NPD) and mass spectrometry (GC-MS) have been employed to quantify levels of 2-DCEIF in plasma and urine. These methods allow for precise monitoring of drug levels, which is crucial for dose adjustments during treatment .
Toxicity Studies
Toxicity associated with ifosfamide treatment has been a significant concern, particularly regarding central neurotoxicity. Research indicates that monitoring levels of 2-DCEIF can provide insights into the risk of adverse effects.
Case Studies:
- A study investigated the relationship between plasma concentrations of 2-DCEIF and neurotoxic effects in patients receiving ifosfamide. The findings suggested that higher levels of this metabolite correlated with increased risk of encephalopathy, emphasizing the need for careful monitoring during treatment .
- Another research effort focused on the carcinogenic potential of ifosfamide and its metabolites, including 2-DCEIF. Long-term studies in animal models have indicated a dose-dependent increase in tumor incidence associated with ifosfamide exposure, highlighting the importance of understanding its metabolites' roles in toxicity .
Therapeutic Combinations
Exploring combination therapies involving ifosfamide and other agents has shown promise in enhancing antitumor efficacy while potentially reducing toxicity.
Research Insights:
- A study evaluated the combined use of metformin and ifosfamide in targeting hepatocellular carcinoma (HCC). The results indicated that co-administration could enhance therapeutic outcomes while possibly mitigating some side effects associated with high doses of ifosfamide .
- Investigations into using 2-DCEIF as a biomarker for tailoring combination therapies are ongoing. By correlating metabolite levels with treatment responses, clinicians may optimize dosing regimens to improve patient outcomes.
Summary Table: Key Research Findings on 2-Dechloroethylifosfamide
Wirkmechanismus
2-Dechloroethylifosfamide itself does not have a direct mechanism of action as it is an inactive metabolite. its formation is a result of the metabolic processing of ifosfamide. Ifosfamide exerts its effects through DNA alkylation, leading to the formation of cross-links that inhibit DNA replication and transcription, ultimately causing cell death. The metabolic pathway involving this compound is part of the detoxification process, reducing the active concentration of ifosfamide and its toxic metabolites.
Vergleich Mit ähnlichen Verbindungen
The following table compares key organophosphorus compounds from the evidence, highlighting their structural and functional differences:
Compound Name | CAS Registry Number | Molecular Formula | Synonyms | Key Functional Groups |
---|---|---|---|---|
Ethylphosphonous dichloride | 1498–40–4 | C₂H₅Cl₂P | Dichloroethylphosphine, Ethyl phosphonous dichloride, Ethyldichlorophosphine | P–Cl bonds, ethyl group |
Ethylphosphonyl dichloride | 1066–50–8 | C₂H₅Cl₂OP | Dichloroethylphosphine oxide, Ethylphosphonic dichloride | P=O bond, P–Cl bonds, ethyl group |
2-Ethylhexyl methylphosphonofluoridate | 458-71-9 | C₉H₂₀FO₂P | O-2-Ethylhexyl methylphosphonofluoridate, Phosphonofluoridic acid methyl ester | P–F bond, branched alkyl chain |
Key Findings:
Functional Group Variability: Ethylphosphonous dichloride lacks an oxygen atom on phosphorus, distinguishing it from ethylphosphonyl dichloride, which has a P=O bond. This difference impacts reactivity; the latter is more polar and likely less volatile . 2-Ethylhexyl methylphosphonofluoridate contains a P–F bond, which is rare in the other analogs. Fluorine’s electronegativity enhances stability and toxicity, as seen in nerve agents like sarin .
Structural and Application Differences: Ethylphosphonous dichloride and ethylphosphonyl dichloride are precursors in synthesizing pesticides or pharmaceuticals. The former’s lack of an oxide group may make it more reactive in alkylation reactions .
Toxicity and Handling: Compounds with P–Cl bonds (e.g., ethylphosphonous dichloride) require stringent handling due to hydrolytic release of HCl. In contrast, P–F bonds (e.g., 2-Ethylhexyl methylphosphonofluoridate) resist hydrolysis but pose neurotoxic risks .
Biologische Aktivität
2-Dechloroethylifosfamide (2-DCE-IF) is a significant metabolite of the chemotherapeutic agent ifosfamide, which is widely used in the treatment of various malignancies, including testicular cancer and sarcomas. Understanding the biological activity of 2-DCE-IF is crucial for evaluating its potential therapeutic implications and toxicity profiles. This article reviews the mechanisms of action, pharmacokinetics, and clinical case studies associated with 2-DCE-IF, emphasizing its role in cancer treatment.
The primary biological activity of this compound is its alkylating ability , which is essential for its anticancer effects. Alkylating agents work by adding alkyl groups to DNA, leading to cross-linking and subsequent disruption of DNA replication and transcription processes. This results in cell death, particularly in rapidly dividing cancer cells.
Key Mechanisms:
- DNA Alkylation : 2-DCE-IF interacts with DNA, forming covalent bonds that hinder replication.
- Cytotoxicity : The compound exhibits differential cytotoxicity compared to ifosfamide, suggesting that its effects may vary based on tumor type and treatment regimen.
Pharmacokinetics
The pharmacokinetics of 2-DCE-IF are influenced by the metabolism of ifosfamide. Upon administration, ifosfamide is metabolized primarily in the liver by cytochrome P450 enzymes, leading to several metabolites, including 2-DCE-IF. The balance between active and inactive metabolites can significantly affect therapeutic outcomes and toxicity profiles .
Table 1: Metabolism Pathways of Ifosfamide
Pathway | Metabolite | Activity Level | Toxicity Potential |
---|---|---|---|
Activation | 4-Hydroxyifosfamide | Active | Low |
Inactivation | Chloroacetaldehyde | Inactive | High |
Dechloroethylation | This compound | Moderate | Moderate |
Clinical Case Studies
Several clinical studies have explored the effects and risks associated with ifosfamide and its metabolites, including 2-DCE-IF.
Case Study: Ifosfamide-Induced Encephalopathy
A notable case involved a 55-year-old female patient who developed encephalopathy after receiving ifosfamide. Despite dose adjustments based on renal function, she exhibited symptoms consistent with ifosfamide-induced encephalopathy (IIE), highlighting the neurotoxic potential of its metabolites. The patient was treated successfully with methylene blue and thiamine . This case underscores the importance of monitoring for neurological side effects in patients receiving ifosfamide-based therapies.
Research Findings
Recent studies have focused on understanding the broader implications of 2-DCE-IF's biological activity:
- Differential Cytotoxicity : Research indicates that 2-DCE-IF may have unique cytotoxic profiles depending on the tumor type, suggesting tailored approaches to chemotherapy could enhance efficacy while minimizing side effects.
- Protein Interaction Studies : Proteomic analyses have revealed that exposure to ifosfamide and its metabolites alters protein expression profiles linked to cellular stress responses, potentially contributing to both therapeutic effects and adverse reactions .
Q & A
Basic Research Questions
Q. What analytical techniques are used to identify and quantify 2-dechloroethylifosfamide in biological samples?
- Methodological Answer : High-performance liquid chromatography–nuclear magnetic resonance (HPLC-NMR) spectroscopy is a primary method for isolating and identifying this compound in urine samples. Continuous-flow ³¹P HPLC-NMR determines retention times of phosphorus-containing metabolites, followed by stop-flow ¹H NMR for structural confirmation . Quantification can be achieved using LC-MS/MS, with calibration against reference standards under controlled pH and temperature conditions to ensure stability.
Q. What is the metabolic pathway leading to this compound formation from ifosfamide?
- Methodological Answer : this compound is a secondary metabolite of ifosfamide, formed via oxidative dechlorination catalyzed by hepatic cytochrome P450 enzymes (e.g., CYP3A4). Its generation competes with other pathways, such as 4-hydroxylation (yielding 4-hydroxyifosfamide) and 3-dechloroethylation. Metabolic studies in rats and humans show that coadministration of mesna alters the redox environment, potentially modulating metabolite ratios .
Q. How do this compound concentrations vary with ifosfamide dosing regimens?
- Methodological Answer : In pancreatic cancer models, this compound levels scale nonlinearly with ifosfamide dose. At 250 µM ifosfamide, concentrations are 6.75 ± 1.2 ng/mL, rising to 32.2 ± 4.0 ng/mL at 3 mM. This nonlinearity suggests saturation of metabolic enzymes or competitive inhibition by other metabolites like 4-hydroxyifosfamide . Pharmacokinetic models should account for hepatic enzyme kinetics and renal clearance rates.
Advanced Research Questions
Q. How can researchers resolve contradictions in reported nephrotoxicity mechanisms linked to this compound?
- Methodological Answer : Discrepancies arise from interspecies variability (e.g., rats vs. humans) and differences in mesna coadministration protocols. To address this, design cross-species studies with standardized mesna dosing and monitor urinary biomarkers (e.g., citrate, hippurate) via ¹H NMR to correlate metabolite shifts with renal toxicity . Parallel in vitro assays using renal proximal tubule cells can isolate direct toxic effects of this compound from systemic variables.
Q. What integrated analytical workflows improve sensitivity in detecting trace this compound levels?
- Methodological Answer : Combine HPLC-NMR for structural identification with ultra-high-resolution LC-MS/MS (e.g., Q-TOF instruments) for quantification at ng/mL levels. Sample preparation should include solid-phase extraction (SPE) to remove matrix interferents and stabilize labile metabolites. Validate recovery rates using isotopically labeled internal standards (e.g., ²H₄-2-dechloroethylifosfamide) .
Q. How should in vivo studies be designed to assess this compound’s role in ifosfamide-induced neurotoxicity?
- Methodological Answer : Use knockout rodent models (e.g., CYP3A4-deficient mice) to isolate this compound’s effects. Employ microdialysis for real-time monitoring of cerebral spinal fluid (CSF) metabolite levels. Pair this with behavioral assays (e.g., rotarod tests) and histopathological analysis of brain tissue. Ensure ethical compliance by adhering to ICH guidelines for animal welfare and sample size justification .
Q. What statistical methods validate the reproducibility of this compound quantification across laboratories?
- Methodological Answer : Perform interlaboratory studies using blinded replicate samples. Apply Bland-Altman analysis to assess agreement and calculate intraclass correlation coefficients (ICC). Use multivariate ANOVA to identify sources of variability (e.g., extraction protocols, instrument calibration). Reporting should follow CONSORT guidelines for analytical studies, with raw data shared via repositories like Zenodo .
Q. Methodological Best Practices
Q. How to optimize NMR parameters for tracking this compound in complex biofluids?
- Answer : Use ¹H NMR at 600 MHz or higher with presaturation to suppress water signals. For urine, apply Carr-Purcell-Meiboom-Gill (CPMG) pulse sequences to reduce macromolecule interference. Spectral assignments should reference spiked standards (e.g., this compound at 1.2 ppm for methyl groups) and cross-validate with 2D NMR (e.g., COSY, HSQC) .
Q. What protocols ensure metabolite stability during sample storage and processing?
- Answer : Immediately freeze urine samples at -80°C to prevent degradation. Avoid repeated freeze-thaw cycles. For LC-MS analysis, add 0.1% formic acid to stabilize pH and chelating agents (e.g., EDTA) to inhibit metal-catalyzed oxidation. Validate stability under storage conditions via spike-and-recovery experiments .
Eigenschaften
IUPAC Name |
3-(2-chloroethyl)-2-oxo-1,3,2λ5-oxazaphosphinan-2-amine | |
---|---|---|
Source | PubChem | |
URL | https://pubchem.ncbi.nlm.nih.gov | |
Description | Data deposited in or computed by PubChem | |
InChI |
InChI=1S/C5H12ClN2O2P/c6-2-4-8-3-1-5-10-11(8,7)9/h1-5H2,(H2,7,9) | |
Source | PubChem | |
URL | https://pubchem.ncbi.nlm.nih.gov | |
Description | Data deposited in or computed by PubChem | |
InChI Key |
ROGLJLJCDSTWBN-UHFFFAOYSA-N | |
Source | PubChem | |
URL | https://pubchem.ncbi.nlm.nih.gov | |
Description | Data deposited in or computed by PubChem | |
Canonical SMILES |
C1CN(P(=O)(OC1)N)CCCl | |
Source | PubChem | |
URL | https://pubchem.ncbi.nlm.nih.gov | |
Description | Data deposited in or computed by PubChem | |
Molecular Formula |
C5H12ClN2O2P | |
Source | PubChem | |
URL | https://pubchem.ncbi.nlm.nih.gov | |
Description | Data deposited in or computed by PubChem | |
DSSTOX Substance ID |
DTXSID90909417 | |
Record name | 2-Dechloroethylifosfamide | |
Source | EPA DSSTox | |
URL | https://comptox.epa.gov/dashboard/DTXSID90909417 | |
Description | DSSTox provides a high quality public chemistry resource for supporting improved predictive toxicology. | |
Molecular Weight |
198.59 g/mol | |
Source | PubChem | |
URL | https://pubchem.ncbi.nlm.nih.gov | |
Description | Data deposited in or computed by PubChem | |
CAS No. |
53459-55-5, 105308-45-0 | |
Record name | Dechloroethylifosfamide | |
Source | CAS Common Chemistry | |
URL | https://commonchemistry.cas.org/detail?cas_rn=53459-55-5 | |
Description | CAS Common Chemistry is an open community resource for accessing chemical information. Nearly 500,000 chemical substances from CAS REGISTRY cover areas of community interest, including common and frequently regulated chemicals, and those relevant to high school and undergraduate chemistry classes. This chemical information, curated by our expert scientists, is provided in alignment with our mission as a division of the American Chemical Society. | |
Explanation | The data from CAS Common Chemistry is provided under a CC-BY-NC 4.0 license, unless otherwise stated. | |
Record name | Dechloroethylifosfamide | |
Source | ChemIDplus | |
URL | https://pubchem.ncbi.nlm.nih.gov/substance/?source=chemidplus&sourceid=0053459555 | |
Description | ChemIDplus is a free, web search system that provides access to the structure and nomenclature authority files used for the identification of chemical substances cited in National Library of Medicine (NLM) databases, including the TOXNET system. | |
Record name | 3-(2-Chloroethyl)-1,3,2-oxazaphosphinan-2-amine 2-oxide | |
Source | ChemIDplus | |
URL | https://pubchem.ncbi.nlm.nih.gov/substance/?source=chemidplus&sourceid=0105308450 | |
Description | ChemIDplus is a free, web search system that provides access to the structure and nomenclature authority files used for the identification of chemical substances cited in National Library of Medicine (NLM) databases, including the TOXNET system. | |
Record name | 2-Dechloroethylifosfamide | |
Source | EPA DSSTox | |
URL | https://comptox.epa.gov/dashboard/DTXSID90909417 | |
Description | DSSTox provides a high quality public chemistry resource for supporting improved predictive toxicology. | |
Record name | 2-amino-3-(2-chloroethyl)-1,3,2-oxazaphosphinane 2-oxide | |
Source | European Chemicals Agency (ECHA) | |
URL | https://echa.europa.eu/information-on-chemicals | |
Description | The European Chemicals Agency (ECHA) is an agency of the European Union which is the driving force among regulatory authorities in implementing the EU's groundbreaking chemicals legislation for the benefit of human health and the environment as well as for innovation and competitiveness. | |
Explanation | Use of the information, documents and data from the ECHA website is subject to the terms and conditions of this Legal Notice, and subject to other binding limitations provided for under applicable law, the information, documents and data made available on the ECHA website may be reproduced, distributed and/or used, totally or in part, for non-commercial purposes provided that ECHA is acknowledged as the source: "Source: European Chemicals Agency, http://echa.europa.eu/". Such acknowledgement must be included in each copy of the material. ECHA permits and encourages organisations and individuals to create links to the ECHA website under the following cumulative conditions: Links can only be made to webpages that provide a link to the Legal Notice page. | |
Record name | 2-DECHLOROETHYLIFOSFAMIDE | |
Source | FDA Global Substance Registration System (GSRS) | |
URL | https://gsrs.ncats.nih.gov/ginas/app/beta/substances/IIB78D4DQA | |
Description | The FDA Global Substance Registration System (GSRS) enables the efficient and accurate exchange of information on what substances are in regulated products. Instead of relying on names, which vary across regulatory domains, countries, and regions, the GSRS knowledge base makes it possible for substances to be defined by standardized, scientific descriptions. | |
Explanation | Unless otherwise noted, the contents of the FDA website (www.fda.gov), both text and graphics, are not copyrighted. They are in the public domain and may be republished, reprinted and otherwise used freely by anyone without the need to obtain permission from FDA. Credit to the U.S. Food and Drug Administration as the source is appreciated but not required. | |
Record name | 2-Dechloroethylifosfamide | |
Source | Human Metabolome Database (HMDB) | |
URL | http://www.hmdb.ca/metabolites/HMDB0013859 | |
Description | The Human Metabolome Database (HMDB) is a freely available electronic database containing detailed information about small molecule metabolites found in the human body. | |
Explanation | HMDB is offered to the public as a freely available resource. Use and re-distribution of the data, in whole or in part, for commercial purposes requires explicit permission of the authors and explicit acknowledgment of the source material (HMDB) and the original publication (see the HMDB citing page). We ask that users who download significant portions of the database cite the HMDB paper in any resulting publications. | |
Retrosynthesis Analysis
AI-Powered Synthesis Planning: Our tool employs the Template_relevance Pistachio, Template_relevance Bkms_metabolic, Template_relevance Pistachio_ringbreaker, Template_relevance Reaxys, Template_relevance Reaxys_biocatalysis model, leveraging a vast database of chemical reactions to predict feasible synthetic routes.
One-Step Synthesis Focus: Specifically designed for one-step synthesis, it provides concise and direct routes for your target compounds, streamlining the synthesis process.
Accurate Predictions: Utilizing the extensive PISTACHIO, BKMS_METABOLIC, PISTACHIO_RINGBREAKER, REAXYS, REAXYS_BIOCATALYSIS database, our tool offers high-accuracy predictions, reflecting the latest in chemical research and data.
Strategy Settings
Precursor scoring | Relevance Heuristic |
---|---|
Min. plausibility | 0.01 |
Model | Template_relevance |
Template Set | Pistachio/Bkms_metabolic/Pistachio_ringbreaker/Reaxys/Reaxys_biocatalysis |
Top-N result to add to graph | 6 |
Feasible Synthetic Routes
Haftungsausschluss und Informationen zu In-Vitro-Forschungsprodukten
Bitte beachten Sie, dass alle Artikel und Produktinformationen, die auf BenchChem präsentiert werden, ausschließlich zu Informationszwecken bestimmt sind. Die auf BenchChem zum Kauf angebotenen Produkte sind speziell für In-vitro-Studien konzipiert, die außerhalb lebender Organismen durchgeführt werden. In-vitro-Studien, abgeleitet von dem lateinischen Begriff "in Glas", beinhalten Experimente, die in kontrollierten Laborumgebungen unter Verwendung von Zellen oder Geweben durchgeführt werden. Es ist wichtig zu beachten, dass diese Produkte nicht als Arzneimittel oder Medikamente eingestuft sind und keine Zulassung der FDA für die Vorbeugung, Behandlung oder Heilung von medizinischen Zuständen, Beschwerden oder Krankheiten erhalten haben. Wir müssen betonen, dass jede Form der körperlichen Einführung dieser Produkte in Menschen oder Tiere gesetzlich strikt untersagt ist. Es ist unerlässlich, sich an diese Richtlinien zu halten, um die Einhaltung rechtlicher und ethischer Standards in Forschung und Experiment zu gewährleisten.