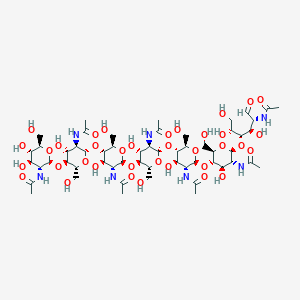
Hepta-N-acetylchitoheptaose
- Klicken Sie auf QUICK INQUIRY, um ein Angebot von unserem Expertenteam zu erhalten.
- Mit qualitativ hochwertigen Produkten zu einem WETTBEWERBSFÄHIGEN Preis können Sie sich mehr auf Ihre Forschung konzentrieren.
Übersicht
Beschreibung
Hepta-N-acetylchitoheptaose is a heptamer of N-acetylglucosamine, a derivative of chitin This compound is composed of seven N-acetylglucosamine units linked by β-1,4-glycosidic bonds
Wissenschaftliche Forschungsanwendungen
Hepta-N-acetylchitoheptaose has a wide range of applications in scientific research, including:
Chemistry: It is used as a model compound to study the properties and reactions of chitooligosaccharides.
Biology: It plays a role in plant-microbe interactions, acting as an elicitor of plant defense responses.
Industry: It is used in the production of biodegradable materials and as a component in cosmetic formulations.
Wirkmechanismus
Zukünftige Richtungen
The method for functional testing constitutive and ligand-induced interactions of LysM-type proteins could serve as a powerful tool to identify and characterize co-receptors or scaffold proteins in receptor complexes . This could provide clues for homodimerization of a given LysM-type protein or to test specific candidate receptor pairs for functionality .
Vorbereitungsmethoden
Synthetic Routes and Reaction Conditions: Hepta-N-acetylchitoheptaose can be synthesized through the partial hydrolysis of chitin or chitosan. The hydrolysis can be achieved using chemical methods such as acid hydrolysis or enzymatic methods using chitinase enzymes. The reaction conditions typically involve controlled temperature and pH to ensure selective cleavage of the glycosidic bonds.
Industrial Production Methods: Industrial production of this compound often involves the use of chitinase enzymes to hydrolyze chitin or chitosan. The process includes:
Substrate Preparation: Chitin or chitosan is prepared from crustacean shells.
Enzymatic Hydrolysis: Chitinase enzymes are used to hydrolyze the chitin or chitosan into chitooligosaccharides, including this compound.
Purification: The resulting mixture is purified using techniques such as chromatography to isolate this compound.
Analyse Chemischer Reaktionen
Types of Reactions: Hepta-N-acetylchitoheptaose undergoes various chemical reactions, including:
Oxidation: It can be oxidized to produce chitooligosaccharide derivatives with different functional groups.
Reduction: Reduction reactions can modify the acetyl groups or the glycosidic bonds.
Substitution: Substitution reactions can introduce new functional groups into the molecule, enhancing its biological activity.
Common Reagents and Conditions:
Oxidation: Reagents such as hydrogen peroxide or periodate are commonly used under controlled pH and temperature conditions.
Reduction: Reducing agents like sodium borohydride are used under mild conditions to avoid degradation of the oligosaccharide.
Substitution: Various reagents, including acylating agents or alkylating agents, are used under specific conditions to achieve the desired substitutions.
Major Products: The major products formed from these reactions include oxidized chitooligosaccharides, reduced chitooligosaccharides, and substituted derivatives with enhanced biological activities.
Vergleich Mit ähnlichen Verbindungen
Chitohexaose: A hexamer of N-acetylglucosamine with similar biological activities.
Chitopentaose: A pentamer of N-acetylglucosamine with slightly different properties.
Chitotetraose: A tetramer of N-acetylglucosamine with distinct biological effects.
Uniqueness: Hepta-N-acetylchitoheptaose is unique due to its specific length and structure, which confer distinct biological activities and interactions compared to shorter or longer chitooligosaccharides
Eigenschaften
IUPAC Name |
N-[(2S,3R,4R,5S,6R)-2-[(2R,3S,4R,5R,6S)-5-acetamido-6-[(2R,3S,4R,5R,6S)-5-acetamido-6-[(2R,3S,4R,5R,6S)-5-acetamido-6-[(2R,3S,4R,5R,6S)-5-acetamido-6-[(2R,3S,4R,5R,6S)-5-acetamido-6-[(2R,3S,4R,5R)-5-acetamido-1,2,4-trihydroxy-6-oxohexan-3-yl]oxy-4-hydroxy-2-(hydroxymethyl)oxan-3-yl]oxy-4-hydroxy-2-(hydroxymethyl)oxan-3-yl]oxy-4-hydroxy-2-(hydroxymethyl)oxan-3-yl]oxy-4-hydroxy-2-(hydroxymethyl)oxan-3-yl]oxy-4-hydroxy-2-(hydroxymethyl)oxan-3-yl]oxy-4,5-dihydroxy-6-(hydroxymethyl)oxan-3-yl]acetamide |
Source
|
---|---|---|
Source | PubChem | |
URL | https://pubchem.ncbi.nlm.nih.gov | |
Description | Data deposited in or computed by PubChem | |
InChI |
InChI=1S/C56H93N7O36/c1-16(72)57-23(8-64)37(80)45(24(79)9-65)94-52-32(59-18(3)74)40(83)47(26(11-67)89-52)96-54-34(61-20(5)76)42(85)49(28(13-69)91-54)98-56-36(63-22(7)78)44(87)50(30(15-71)93-56)99-55-35(62-21(6)77)43(86)48(29(14-70)92-55)97-53-33(60-19(4)75)41(84)46(27(12-68)90-53)95-51-31(58-17(2)73)39(82)38(81)25(10-66)88-51/h8,23-56,65-71,79-87H,9-15H2,1-7H3,(H,57,72)(H,58,73)(H,59,74)(H,60,75)(H,61,76)(H,62,77)(H,63,78)/t23-,24+,25+,26+,27+,28+,29+,30+,31+,32+,33+,34+,35+,36+,37+,38+,39+,40+,41+,42+,43+,44+,45+,46+,47+,48+,49+,50+,51-,52-,53-,54-,55-,56-/m0/s1 |
Source
|
Source | PubChem | |
URL | https://pubchem.ncbi.nlm.nih.gov | |
Description | Data deposited in or computed by PubChem | |
InChI Key |
UKZKHAFAYSQVFL-MBGZTGDNSA-N |
Source
|
Source | PubChem | |
URL | https://pubchem.ncbi.nlm.nih.gov | |
Description | Data deposited in or computed by PubChem | |
Canonical SMILES |
CC(=O)NC1C(C(C(OC1OC2C(OC(C(C2O)NC(=O)C)OC3C(OC(C(C3O)NC(=O)C)OC4C(OC(C(C4O)NC(=O)C)OC5C(OC(C(C5O)NC(=O)C)OC6C(OC(C(C6O)NC(=O)C)OC(C(CO)O)C(C(C=O)NC(=O)C)O)CO)CO)CO)CO)CO)CO)O)O |
Source
|
Source | PubChem | |
URL | https://pubchem.ncbi.nlm.nih.gov | |
Description | Data deposited in or computed by PubChem | |
Isomeric SMILES |
CC(=O)N[C@@H]1[C@H]([C@@H]([C@H](O[C@H]1O[C@@H]2[C@H](O[C@H]([C@@H]([C@H]2O)NC(=O)C)O[C@@H]3[C@H](O[C@H]([C@@H]([C@H]3O)NC(=O)C)O[C@@H]4[C@H](O[C@H]([C@@H]([C@H]4O)NC(=O)C)O[C@@H]5[C@H](O[C@H]([C@@H]([C@H]5O)NC(=O)C)O[C@@H]6[C@H](O[C@H]([C@@H]([C@H]6O)NC(=O)C)O[C@H]([C@@H](CO)O)[C@@H]([C@H](C=O)NC(=O)C)O)CO)CO)CO)CO)CO)CO)O)O |
Source
|
Source | PubChem | |
URL | https://pubchem.ncbi.nlm.nih.gov | |
Description | Data deposited in or computed by PubChem | |
Molecular Formula |
C56H93N7O36 |
Source
|
Source | PubChem | |
URL | https://pubchem.ncbi.nlm.nih.gov | |
Description | Data deposited in or computed by PubChem | |
Molecular Weight |
1440.4 g/mol |
Source
|
Source | PubChem | |
URL | https://pubchem.ncbi.nlm.nih.gov | |
Description | Data deposited in or computed by PubChem | |
CAS No. |
79127-58-5 |
Source
|
Record name | Hepta-N-acetylchitoheptaose | |
Source | ChemIDplus | |
URL | https://pubchem.ncbi.nlm.nih.gov/substance/?source=chemidplus&sourceid=0079127585 | |
Description | ChemIDplus is a free, web search system that provides access to the structure and nomenclature authority files used for the identification of chemical substances cited in National Library of Medicine (NLM) databases, including the TOXNET system. | |
Haftungsausschluss und Informationen zu In-Vitro-Forschungsprodukten
Bitte beachten Sie, dass alle Artikel und Produktinformationen, die auf BenchChem präsentiert werden, ausschließlich zu Informationszwecken bestimmt sind. Die auf BenchChem zum Kauf angebotenen Produkte sind speziell für In-vitro-Studien konzipiert, die außerhalb lebender Organismen durchgeführt werden. In-vitro-Studien, abgeleitet von dem lateinischen Begriff "in Glas", beinhalten Experimente, die in kontrollierten Laborumgebungen unter Verwendung von Zellen oder Geweben durchgeführt werden. Es ist wichtig zu beachten, dass diese Produkte nicht als Arzneimittel oder Medikamente eingestuft sind und keine Zulassung der FDA für die Vorbeugung, Behandlung oder Heilung von medizinischen Zuständen, Beschwerden oder Krankheiten erhalten haben. Wir müssen betonen, dass jede Form der körperlichen Einführung dieser Produkte in Menschen oder Tiere gesetzlich strikt untersagt ist. Es ist unerlässlich, sich an diese Richtlinien zu halten, um die Einhaltung rechtlicher und ethischer Standards in Forschung und Experiment zu gewährleisten.