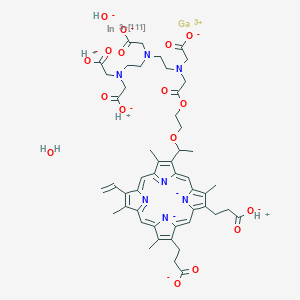
Indium(111)-atn-2
Übersicht
Beschreibung
Indium(111)-atn-2 is a radiopharmaceutical compound that incorporates the radioisotope indium-111. Indium-111 is a gamma-emitting radionuclide with a half-life of approximately 2.8 days. This compound is primarily used in diagnostic imaging and therapeutic applications due to its ability to emit gamma rays, which can be detected using gamma cameras. This compound is particularly valuable in nuclear medicine for labeling monoclonal antibodies, peptides, and other biomolecules for imaging and therapeutic purposes .
Vorbereitungsmethoden
Synthetic Routes and Reaction Conditions: Indium(111)-atn-2 is synthesized by radiolabeling a suitable ligand with indium-111. The process typically involves the following steps:
Production of Indium-111: Indium-111 is produced by bombarding a cadmium target with protons in a cyclotron.
Purification of Indium-111: The produced indium-111 is separated from the target material using column chromatography to obtain high-purity indium-111 chloride.
Radiolabeling: The purified indium-111 chloride is then reacted with a ligand (such as a monoclonal antibody or peptide) under specific conditions to form this compound.
Industrial Production Methods: Industrial production of this compound follows similar steps but on a larger scale. Automated systems and stringent quality control measures ensure the consistent production of high-purity radiopharmaceuticals .
Analyse Chemischer Reaktionen
Types of Reactions: Indium(111)-atn-2 undergoes various chemical reactions, including:
Substitution: Ligands can be substituted with other chelating agents to modify the properties of the radiopharmaceutical.
Common Reagents and Conditions:
Major Products: The major products of these reactions are radiolabeled complexes that retain the biological activity of the ligand while incorporating the gamma-emitting properties of indium-111 .
Wissenschaftliche Forschungsanwendungen
Indium(111)-atn-2 has a wide range of scientific research applications, including:
Chemistry: Used in studies involving the synthesis and characterization of radiolabeled compounds.
Biology: Employed in tracking cellular processes and studying the biodistribution of labeled biomolecules.
Medicine: Widely used in diagnostic imaging for detecting tumors, infections, and other pathological conditions.
Industry: Utilized in the development of new radiopharmaceuticals and imaging agents.
Wirkmechanismus
Indium(111)-atn-2 can be compared with other radiopharmaceuticals that use different radioisotopes or ligands:
Gallium-68: A positron-emitting radionuclide used in positron emission tomography (PET) imaging.
Technetium-99m: A widely used gamma-emitting radionuclide with a half-life of 6 hours.
Fluorine-18: A positron-emitting radionuclide used in PET imaging with a half-life of 110 minutes.
Uniqueness of this compound: this compound is unique due to its relatively long half-life, which allows for extended imaging periods. Its ability to form stable complexes with various ligands makes it versatile for different diagnostic and therapeutic applications .
Vergleich Mit ähnlichen Verbindungen
- Gallium-68-labeled compounds
- Technetium-99m-labeled compounds
- Fluorine-18-labeled compounds
Indium(111)-atn-2 stands out for its specific applications in nuclear medicine, particularly in scenarios where longer imaging times are required.
Biologische Aktivität
2-(4-Ethoxyphenyl)imidazole is a heterocyclic compound that has garnered attention in medicinal chemistry due to its diverse biological activities. This compound features an imidazole ring substituted with a 4-ethoxyphenyl group, which enhances its lipophilicity and potential for biological interaction. Research has demonstrated its efficacy as an enzyme inhibitor, receptor modulator, and potential therapeutic agent against various diseases, including cancer and microbial infections.
The synthesis of 2-(4-Ethoxyphenyl)imidazole typically involves the cyclization of 4-ethoxybenzaldehyde with glyoxal and ammonium acetate under acidic conditions. This method allows for the formation of the imidazole ring through a condensation reaction, yielding a compound that exhibits significant biological activity due to its unique structural characteristics.
The biological activity of 2-(4-Ethoxyphenyl)imidazole is largely attributed to its ability to interact with various biological targets, including enzymes and receptors. The imidazole ring can coordinate with metal ions, influencing biochemical pathways, while the ethoxyphenyl group enhances solubility and membrane permeability, facilitating cellular uptake.
Anticancer Activity
Recent studies have highlighted the anticancer potential of 2-(4-Ethoxyphenyl)imidazole derivatives. For instance, a study demonstrated that compounds with similar structural motifs exhibited IC50 values ranging from 0.4 to 3.8 nM against multiple cancer cell lines, indicating potent antiproliferative effects . The presence of substituents on the phenyl ring significantly influenced the biological activity, with specific configurations leading to enhanced efficacy.
Table 1: Anticancer Activity of 2-(4-Ethoxyphenyl)imidazole Derivatives
Compound | Cancer Cell Line | IC50 (nM) |
---|---|---|
4o | A549 | 0.4 |
HeLa | 1.5 | |
MCF-7 | 3.8 | |
Jurkat | >10 |
The compound 4o , a derivative of 2-(4-Ethoxyphenyl)imidazole, showed promising results in vivo, significantly reducing tumor mass in mouse models at doses much lower than conventional treatments .
Antimicrobial Activity
In addition to anticancer properties, 2-(4-Ethoxyphenyl)imidazole has been investigated for its antimicrobial effects. Studies have shown that derivatives exhibit activity against various bacterial strains such as Staphylococcus aureus and Escherichia coli, with some compounds demonstrating comparable efficacy to established antibiotics .
Table 2: Antimicrobial Activity of Imidazole Derivatives
Compound | Bacterial Strain | Zone of Inhibition (mm) |
---|---|---|
1a | S. aureus | 15 |
E. coli | 12 | |
B. subtilis | 10 |
Case Studies
- Anticancer Efficacy : In a study evaluating the effects of various substituents on the phenyl ring of imidazole derivatives, it was found that compounds containing both chloro and ethoxy groups exhibited superior anticancer activity compared to their counterparts without these substitutions .
- Cytotoxicity Assessment : The cytotoxic effects of the most active derivative (compound 4o ) were assessed on peripheral blood lymphocytes (PBL). Results indicated an IC50 greater than 10 μM in quiescent cells but significantly lower (0.5 μM) in stimulated cells, suggesting selective toxicity towards rapidly proliferating cancer cells while sparing normal cells .
Eigenschaften
IUPAC Name |
gallium;3-[13-[1-[2-[2-[2-[2-[bis(carboxylatomethyl)amino]ethyl-(carboxylatomethyl)amino]ethyl-(carboxylatomethyl)amino]acetyl]oxyethoxy]ethyl]-18-(2-carboxylatoethyl)-8-ethenyl-3,7,12,17-tetramethylporphyrin-21,24-diid-2-yl]propanoate;hydron;indium-111(3+);hydroxide;hydrate | |
---|---|---|
Source | PubChem | |
URL | https://pubchem.ncbi.nlm.nih.gov | |
Description | Data deposited in or computed by PubChem | |
InChI |
InChI=1S/C50H61N7O15.Ga.In.2H2O/c1-7-32-27(2)35-18-36-28(3)33(8-10-43(58)59)40(52-36)21-41-34(9-11-44(60)61)29(4)37(53-41)20-42-50(30(5)38(54-42)19-39(32)51-35)31(6)71-16-17-72-49(70)26-57(25-48(68)69)15-13-55(22-45(62)63)12-14-56(23-46(64)65)24-47(66)67;;;;/h7,18-21,31H,1,8-17,22-26H2,2-6H3,(H8,51,52,53,54,58,59,60,61,62,63,64,65,66,67,68,69);;;2*1H2/q;2*+3;;/p-6/i;;1-4;; | |
Source | PubChem | |
URL | https://pubchem.ncbi.nlm.nih.gov | |
Description | Data deposited in or computed by PubChem | |
InChI Key |
UHBWZLWDCQKNAP-XVKFRELOSA-H | |
Source | PubChem | |
URL | https://pubchem.ncbi.nlm.nih.gov | |
Description | Data deposited in or computed by PubChem | |
Canonical SMILES |
[H+].[H+].[H+].CC1=C(C2=CC3=C(C(=C([N-]3)C=C4C(=C(C(=N4)C=C5C(=C(C(=N5)C=C1[N-]2)C)C=C)C)C(C)OCCOC(=O)CN(CCN(CCN(CC(=O)[O-])CC(=O)[O-])CC(=O)[O-])CC(=O)[O-])C)CCC(=O)[O-])CCC(=O)[O-].O.[OH-].[Ga+3].[In+3] | |
Source | PubChem | |
URL | https://pubchem.ncbi.nlm.nih.gov | |
Description | Data deposited in or computed by PubChem | |
Isomeric SMILES |
[H+].[H+].[H+].CC1=C(C2=CC3=C(C(=C([N-]3)C=C4C(=C(C(=N4)C=C5C(=C(C(=N5)C=C1[N-]2)C)C=C)C)C(C)OCCOC(=O)CN(CCN(CCN(CC(=O)[O-])CC(=O)[O-])CC(=O)[O-])CC(=O)[O-])C)CCC(=O)[O-])CCC(=O)[O-].O.[OH-].[Ga+3].[111In+3] | |
Source | PubChem | |
URL | https://pubchem.ncbi.nlm.nih.gov | |
Description | Data deposited in or computed by PubChem | |
Molecular Formula |
C50H59GaInN7O17 | |
Source | PubChem | |
URL | https://pubchem.ncbi.nlm.nih.gov | |
Description | Data deposited in or computed by PubChem | |
Molecular Weight |
1210.7 g/mol | |
Source | PubChem | |
URL | https://pubchem.ncbi.nlm.nih.gov | |
Description | Data deposited in or computed by PubChem | |
CAS No. |
119130-46-0 | |
Record name | ATN-2 | |
Source | ChemIDplus | |
URL | https://pubchem.ncbi.nlm.nih.gov/substance/?source=chemidplus&sourceid=0119130460 | |
Description | ChemIDplus is a free, web search system that provides access to the structure and nomenclature authority files used for the identification of chemical substances cited in National Library of Medicine (NLM) databases, including the TOXNET system. | |
Haftungsausschluss und Informationen zu In-Vitro-Forschungsprodukten
Bitte beachten Sie, dass alle Artikel und Produktinformationen, die auf BenchChem präsentiert werden, ausschließlich zu Informationszwecken bestimmt sind. Die auf BenchChem zum Kauf angebotenen Produkte sind speziell für In-vitro-Studien konzipiert, die außerhalb lebender Organismen durchgeführt werden. In-vitro-Studien, abgeleitet von dem lateinischen Begriff "in Glas", beinhalten Experimente, die in kontrollierten Laborumgebungen unter Verwendung von Zellen oder Geweben durchgeführt werden. Es ist wichtig zu beachten, dass diese Produkte nicht als Arzneimittel oder Medikamente eingestuft sind und keine Zulassung der FDA für die Vorbeugung, Behandlung oder Heilung von medizinischen Zuständen, Beschwerden oder Krankheiten erhalten haben. Wir müssen betonen, dass jede Form der körperlichen Einführung dieser Produkte in Menschen oder Tiere gesetzlich strikt untersagt ist. Es ist unerlässlich, sich an diese Richtlinien zu halten, um die Einhaltung rechtlicher und ethischer Standards in Forschung und Experiment zu gewährleisten.