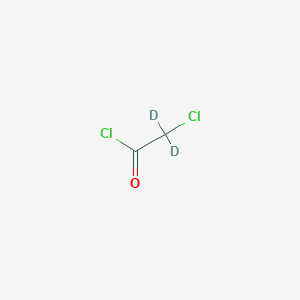
Chloracetyl-D2-chlorid
Übersicht
Beschreibung
Chloroacetyl-D2 chloride is a deuterated derivative of chloroacetyl chloride, with the molecular formula C2D2Cl2O. This compound is characterized by the substitution of hydrogen atoms with deuterium, a stable isotope of hydrogen. Chloroacetyl-D2 chloride is a colorless to yellow liquid that is primarily used as a reagent in organic synthesis.
Wissenschaftliche Forschungsanwendungen
Pharmaceutical Applications
Chloroacetyl-D2 chloride plays a crucial role in the synthesis of pharmaceutical compounds, particularly those aimed at treating cancer and neurological disorders.
Synthesis of Anti-Tumor Drugs
Research has demonstrated that chloroacetyl-D2 chloride can be effectively used to synthesize novel anti-tumor agents. For instance, a study reported the synthesis of 12 derivatives of 25-hydroxyprotopanaxadiol (25-OH-PPD) through acylation with chloroacetyl-D2 chloride. These derivatives were evaluated for their cytotoxicity against various human tumor cell lines using the MTT assay. Notably, compounds 4, 6, and 7 exhibited significant cytotoxic activity, with IC50 values indicating strong inhibition against MCF-7 and HCT-116 cells (IC50 values of 1.7 μM and 1.6 μM respectively) .
Neuroprotective Agents
Chloroacetyl-D2 chloride has also been utilized in the development of bivalent dopamine agonists that show enhanced affinity for dopamine D2 receptors. These compounds are designed to provide neuroprotective effects, particularly in conditions like Parkinson's disease. The structural modifications involving chloroacetyl-D2 chloride have resulted in compounds with significantly improved binding affinities compared to traditional ligands .
Agrochemical Applications
In addition to its pharmaceutical uses, chloroacetyl-D2 chloride is employed in the synthesis of agrochemicals.
Herbicide Development
Chloroacetyl-D2 chloride has been used in the study of chloroacetamide herbicides, where it serves as a precursor for synthesizing active ingredients that inhibit weed growth. Research involving rat and human liver microsomes has provided insights into the metabolism of these herbicides, enhancing our understanding of their environmental impact and efficacy .
Synthesis of Surfactants
Chloroacetyl-D2 chloride is also significant in the preparation of surfactants that improve drug delivery systems.
Drug Delivery Enhancements
A notable application involves linking chloroacetyl-D2 chloride to poly(ethylene glycols) (PEG) to create prodrugs like Metaxalone-PEG conjugates. These conjugates have shown improved pharmacokinetics, including extended half-life and controlled release profiles, thereby enhancing therapeutic efficacy .
Data Summary Table
Case Study 1: Antitumor Activity Evaluation
In a comprehensive study on the antitumor activity of synthesized compounds from chloroacetyl-D2 chloride, researchers conducted in vitro assays on six human tumor cell lines. The results indicated that certain derivatives not only inhibited cell proliferation but also induced apoptosis in cancer cells, showcasing potential as therapeutic agents.
Case Study 2: Neuroprotective Compound Development
Another study focused on the development of new bivalent dopamine agonists utilizing chloroacetyl-D2 chloride as a key intermediate. The structural modifications led to compounds that demonstrated cooperative binding at D2 receptors, resulting in a significant increase in neuroprotective activity compared to existing treatments.
Wirkmechanismus
Target of Action
Chloroacetyl-D2 chloride is a chlorinated acyl chloride . It is a bifunctional compound, making it a useful building block chemical . It can react with other compounds to form acylated derivatives, which undergo further chemical reactions .
Mode of Action
The mode of action of Chloroacetyl-D2 chloride is primarily through its ability to form acyl chloride groups . It can react with other compounds to form acylated products such as acyl chloride derivatives, amides, esters, etc., and participate in further chemical reactions such as nucleophilic substitution, condensation, etc .
Biochemical Pathways
Chloroacetyl-D2 chloride is used as a chlorinating agent, acylating agent, precursor to monochloroketene, and as a building block for cyclization . Chloroacetyl-D2 chloride causes the acylation of cyano-pyrazoline during the synthesis of secondary amine-substituted cyano-pyrazoline derivatives .
Pharmacokinetics
It is known that this compound is utilized in the preparation of surfactants, enhancing drug delivery and pharmacokinetics .
Result of Action
The major use of Chloroacetyl-D2 chloride is as an intermediate in the production of herbicides in the chloroacetanilide family including metolachlor, acetochlor, alachlor and butachlor . Some Chloroacetyl-D2 chloride is also used to produce phenacyl chloride, another chemical intermediate, also used as a tear gas .
Action Environment
Like other acyl chlorides, reaction with other protic compounds such as amines, alcohols, and water generates hydrochloric acid, making it a lachrymator . There is no regulated permissible exposure limit set by the Occupational Safety and Health Administration . Therefore, it is essential to note that this compound poses significant toxicity risks, causing eye irritation and organ damage upon exposure . Strict safety precautions must be implemented when handling Chloroacetyl-D2 chloride to safeguard human health and the environment .
Vorbereitungsmethoden
Synthetic Routes and Reaction Conditions
Chloroacetyl-D2 chloride can be synthesized through several methods:
Carbonylation of Methylene Chloride: This method involves the reaction of methylene chloride with carbon monoxide in the presence of a catalyst.
Oxidation of Vinylidene Chloride: Vinylidene chloride is oxidized to produce chloroacetyl chloride, which can then be deuterated.
Addition of Chlorine to Ketene: Ketene reacts with chlorine to form chloroacetyl chloride, which is subsequently deuterated.
Industrial Production Methods
Industrially, chloroacetyl chloride is produced by the carbonylation of methylene chloride, oxidation of vinylidene chloride, or the addition of chlorine to ketene. The deuteration process involves the replacement of hydrogen atoms with deuterium, typically using deuterium gas or deuterated reagents.
Analyse Chemischer Reaktionen
Types of Reactions
Chloroacetyl-D2 chloride undergoes various chemical reactions, including:
Nucleophilic Substitution: The chlorine atom can be replaced by nucleophiles such as amines, alcohols, or thiols, forming corresponding amides, esters, or thioesters.
Hydrolysis: Reaction with water results in the formation of chloroacetic acid and hydrochloric acid.
Esterification: Reaction with alcohols to form esters.
Amidation: Reaction with amines to form amides.
Common Reagents and Conditions
Nucleophiles: Amines, alcohols, thiols.
Catalysts: Aluminum chloride for Friedel-Crafts acylation.
Solvents: Tetrahydrofuran, dichloromethane, toluene.
Major Products
Amides: Formed from reaction with amines.
Esters: Formed from reaction with alcohols.
Chloroacetic Acid: Formed from hydrolysis.
Vergleich Mit ähnlichen Verbindungen
Chloroacetyl-D2 chloride is compared with other similar compounds such as:
Chloroacetyl Chloride: The non-deuterated form, which is more commonly used but lacks the stability and tracing capabilities of the deuterated form.
Acetyl Chloride: Lacks the chlorine atom, making it less reactive in nucleophilic substitution reactions.
Bromoacetyl Chloride: Contains a bromine atom instead of chlorine, leading to different reactivity and applications.
Chloroacetyl-D2 chloride is unique due to its deuterium content, which provides enhanced stability and allows for its use in isotope labeling studies.
Biologische Aktivität
Chloroacetyl-D2 chloride (CAS 159301-43-6) is a derivative of chloroacetyl chloride, a highly reactive acylating agent used in various chemical syntheses. This compound has garnered attention for its potential biological activities, particularly in the context of medicinal chemistry and toxicology. This article explores the biological activity of Chloroacetyl-D2 chloride, supported by relevant case studies, research findings, and data tables.
Chloroacetyl-D2 chloride is characterized by its chlorinated acetyl group, which enhances its reactivity. It is primarily utilized in the synthesis of various pharmaceutical compounds, notably praziquantel, an antihelminthic drug effective against schistosomiasis and other parasitic infections. The synthesis involves acylation reactions where chloroacetyl-D2 chloride acts as an acylating agent.
Antimicrobial Properties
Research indicates that chloroacetyl derivatives exhibit significant antimicrobial activity. For instance, azetidin-2-one fused 2-chloro-3-formyl quinoline derivatives synthesized using chloroacetyl chloride demonstrated potent antibacterial and antifungal effects against pathogens such as Staphylococcus aureus and Candida albicans . The following table summarizes the antimicrobial activity of selected chloroacetyl derivatives:
Compound Name | Pathogen Tested | Inhibition Zone (mm) | IC50 (µg/mL) |
---|---|---|---|
AZT b2 | Staphylococcus aureus | 22 | 5.0 |
AZT g3 | Candida albicans | 20 | 4.5 |
AZT e2 | Escherichia coli | 18 | 6.0 |
These findings underscore the potential of chloroacetyl derivatives in developing new antimicrobial agents.
Toxicological Effects
Chloroacetyl chloride and its derivatives are known for their toxicity. A notable case study reported an occupational poisoning incident involving chloroacetyl chloride, where exposure led to severe neurological damage and multiple organ failure in affected individuals . Symptoms included vomiting, irritability, and respiratory distress, highlighting the compound's corrosive nature and potential for causing serious health issues upon exposure.
The biological activity of chloroacetyl-D2 chloride can be attributed to its ability to modify proteins through acylation, impacting various biochemical pathways. For instance, it has been shown to disrupt calcium homeostasis in helminths, leading to paralysis and death of the parasites . This mechanism is critical for its application in synthesizing praziquantel.
Case Studies
- Occupational Exposure : An incident involving a leak of chloroacetyl chloride resulted in severe health complications for workers due to skin contact and inhalation. The corrosive nature of the compound caused burns and respiratory issues, emphasizing the need for stringent safety measures in handling such chemicals .
- Synthesis of Praziquantel : The use of chloroacetyl-D2 chloride in synthesizing praziquantel illustrates its importance in pharmaceutical applications. Praziquantel's efficacy against schistosomiasis is partly due to the disruption of calcium channels in parasitic membranes, facilitated by the acylation process involving chloroacetyl derivatives .
Eigenschaften
IUPAC Name |
2-chloro-2,2-dideuterioacetyl chloride | |
---|---|---|
Source | PubChem | |
URL | https://pubchem.ncbi.nlm.nih.gov | |
Description | Data deposited in or computed by PubChem | |
InChI |
InChI=1S/C2H2Cl2O/c3-1-2(4)5/h1H2/i1D2 | |
Source | PubChem | |
URL | https://pubchem.ncbi.nlm.nih.gov | |
Description | Data deposited in or computed by PubChem | |
InChI Key |
VGCXGMAHQTYDJK-DICFDUPASA-N | |
Source | PubChem | |
URL | https://pubchem.ncbi.nlm.nih.gov | |
Description | Data deposited in or computed by PubChem | |
Canonical SMILES |
C(C(=O)Cl)Cl | |
Source | PubChem | |
URL | https://pubchem.ncbi.nlm.nih.gov | |
Description | Data deposited in or computed by PubChem | |
Isomeric SMILES |
[2H]C([2H])(C(=O)Cl)Cl | |
Source | PubChem | |
URL | https://pubchem.ncbi.nlm.nih.gov | |
Description | Data deposited in or computed by PubChem | |
Molecular Formula |
C2H2Cl2O | |
Source | PubChem | |
URL | https://pubchem.ncbi.nlm.nih.gov | |
Description | Data deposited in or computed by PubChem | |
Molecular Weight |
114.95 g/mol | |
Source | PubChem | |
URL | https://pubchem.ncbi.nlm.nih.gov | |
Description | Data deposited in or computed by PubChem | |
Synthesis routes and methods
Procedure details
Haftungsausschluss und Informationen zu In-Vitro-Forschungsprodukten
Bitte beachten Sie, dass alle Artikel und Produktinformationen, die auf BenchChem präsentiert werden, ausschließlich zu Informationszwecken bestimmt sind. Die auf BenchChem zum Kauf angebotenen Produkte sind speziell für In-vitro-Studien konzipiert, die außerhalb lebender Organismen durchgeführt werden. In-vitro-Studien, abgeleitet von dem lateinischen Begriff "in Glas", beinhalten Experimente, die in kontrollierten Laborumgebungen unter Verwendung von Zellen oder Geweben durchgeführt werden. Es ist wichtig zu beachten, dass diese Produkte nicht als Arzneimittel oder Medikamente eingestuft sind und keine Zulassung der FDA für die Vorbeugung, Behandlung oder Heilung von medizinischen Zuständen, Beschwerden oder Krankheiten erhalten haben. Wir müssen betonen, dass jede Form der körperlichen Einführung dieser Produkte in Menschen oder Tiere gesetzlich strikt untersagt ist. Es ist unerlässlich, sich an diese Richtlinien zu halten, um die Einhaltung rechtlicher und ethischer Standards in Forschung und Experiment zu gewährleisten.