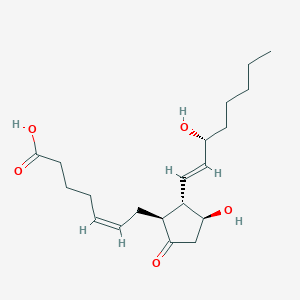
ent-PGE2
Übersicht
Beschreibung
ent-Prostaglandin E2 is a stereoisomer of Prostaglandin E2, a naturally occurring prostaglandin with significant biological activities. Prostaglandins are lipid compounds derived from fatty acids and have diverse roles in the human body, including the regulation of inflammation, blood flow, and the formation of blood clots. ent-Prostaglandin E2, specifically, is known for its involvement in various physiological processes and potential therapeutic applications.
Wissenschaftliche Forschungsanwendungen
ent-Prostaglandin E2 hat eine große Bandbreite an Anwendungen in der wissenschaftlichen Forschung, darunter:
Chemie: Wird als Standard für die Untersuchung der Stereochemie und Reaktivität von Prostaglandinen verwendet.
Biologie: Wird auf seine Rolle bei der zellulären Signalübertragung und der Regulierung physiologischer Prozesse untersucht.
Medizin: Wird auf seine potenziellen therapeutischen Anwendungen bei der Behandlung von Entzündungen, Schmerzen und anderen Erkrankungen untersucht.
Industrie: Wird bei der Entwicklung von Pharmazeutika und als Referenzsubstanz in analytischen Methoden eingesetzt
5. Wirkmechanismus
Der Wirkmechanismus von ent-Prostaglandin E2 beinhaltet seine Interaktion mit spezifischen Prostaglandinrezeptoren, einschließlich EP1, EP2, EP3 und EP4. Diese Rezeptoren sind G-Protein-gekoppelte Rezeptoren, die verschiedene Signalwege vermitteln. Nach Bindung an diese Rezeptoren kann ent-Prostaglandin E2 zelluläre Prozesse wie Entzündungen, Vasodilatation und glatte Muskelkontraktion beeinflussen .
Ähnliche Verbindungen:
Prostaglandin E2: Die natürlich vorkommende Form mit ähnlichen biologischen Aktivitäten.
Prostaglandin F2α: Ein weiteres Prostaglandin mit unterschiedlichen Rollen bei der Kontraktion der glatten Muskulatur und reproduktiven Prozessen.
Prostaglandin D2: An allergischen Reaktionen und der Schlafregulation beteiligt.
Einzigartigkeit: ent-Prostaglandin E2 ist aufgrund seiner spezifischen Stereochemie einzigartig, die zu unterschiedlichen biologischen Aktivitäten im Vergleich zu seinem natürlich vorkommenden Gegenstück führen kann. Dies macht es wertvoll für die Untersuchung der Auswirkungen von Stereochemie auf die Prostaglandinfunktion und für die Entwicklung gezielter Therapeutika .
Wirkmechanismus
Target of Action
The primary targets of ent-PGE2 are the prostaglandin E2 receptors (EP1-4) . These receptors are G protein-coupled receptors with distinct signaling properties . EP2 receptor, in particular, has been shown to mediate the effects of PGE2 on macrophages .
Mode of Action
This compound interacts with its targets, the EP receptors, to induce various downstream effects. For instance, PGE2-EP2 signaling activates PKB, leading to the activation of glycogen synthase and thus glycogen synthesis . Moreover, PGE2 can influence blood pressure both positively and negatively. Centrally administered PGE2 induces hypertension, whereas systemic administration of PGE2 produces a hypotensive effect .
Biochemical Pathways
PGE2 is derived from arachidonic acid (AA) via the actions of the enzyme cytosolic phospholipase A2 (cPLA2), which generates AA. Free AA can then be metabolized via the enzymatic activity of cyclooxygenase 1 or 2 (COX-1 or COX-2) and PGE synthase 1 . PGE2 seems to act on monocyte-derived macrophages (MDMs) in an autocrine fashion to alter metabolism .
Result of Action
The action of this compound leads to various molecular and cellular effects. For instance, it has been shown to increase neurite length and the proportion of neurites that form axonal loops in differentiating NE4C cells . Additionally, PGE2 has been found to reduce oxidative phosphorylation and glycolysis in MDMs , and to promote macrophage recruitment and neovascularization in murine wet-type AMD models .
Action Environment
The action of this compound can be influenced by various environmental factors. For example, abnormal levels of PGE2 due to environmental insults during prenatal development have been linked to brain pathologies . Furthermore, the level of PGE2 expression was found to be high in laser-induced CNV lesions, suggesting that the local environment can influence the action and efficacy of PGE2 .
Biochemische Analyse
Biochemical Properties
ent-Prostaglandin E2 plays a significant role in biochemical reactions. It interacts with various enzymes, proteins, and other biomolecules. For instance, it is involved in the biosynthesis of PGE2, a crucial biochemical signal mediating immune and reproductive physiology . The biosynthesis of ent-Prostaglandin E2 begins with the hydrolysis of arachidonic acid from cellular phospholipids by a phospholipase A2 .
Cellular Effects
ent-Prostaglandin E2 has profound effects on various types of cells and cellular processes. It influences cell function, including impacting cell signaling pathways, gene expression, and cellular metabolism . For example, it plays a relevant role in the ovulatory cascade, including meiotic maturation, cumulus expansion, and follicle rupture .
Molecular Mechanism
The molecular mechanism of ent-Prostaglandin E2 involves its binding interactions with biomolecules, enzyme inhibition or activation, and changes in gene expression . It mediates the biosynthesis of PGE2 through its cognate protein .
Metabolic Pathways
ent-Prostaglandin E2 is involved in several metabolic pathways. It interacts with enzymes or cofactors, and it can affect metabolic flux or metabolite levels .
Vorbereitungsmethoden
Synthesewege und Reaktionsbedingungen: Die Synthese von ent-Prostaglandin E2 erfolgt in der Regel nach der Corey-Synthesemethode, die weit verbreitet für die Herstellung von Prostaglandinen eingesetzt wird. Das Schlüsselzwischenprodukt in dieser Synthese ist das Corey-Lacton, aus dem die Omega- und Alpha-Seitenketten von Prostaglandinen aufgebaut werden können. Der Prozess umfasst mehrere Schritte, darunter die Bildung eines Lactonrings, gefolgt von der Addition von Seitenketten durch verschiedene chemische Reaktionen .
Industrielle Produktionsverfahren: Die industrielle Produktion von ent-Prostaglandin E2 erfolgt im großen Maßstab durch Synthese mit ähnlichen Methoden wie oben beschrieben. Der Prozess wird auf höhere Ausbeuten und Reinheit optimiert und beinhaltet oft die chirale Hochleistungsflüssigkeitschromatographie (HPLC) zur Trennung der Enantiomere . Der Einsatz fortschrittlicher Techniken gewährleistet die Produktion von qualitativ hochwertigem ent-Prostaglandin E2 für Forschungs- und therapeutische Zwecke.
Analyse Chemischer Reaktionen
Arten von Reaktionen: ent-Prostaglandin E2 unterliegt verschiedenen chemischen Reaktionen, darunter:
Oxidation: Umwandlung von Hydroxylgruppen in Ketone oder Aldehyde.
Reduktion: Reduktion von Ketonen zu Hydroxylgruppen.
Substitution: Austausch von funktionellen Gruppen durch andere Substituenten.
Häufige Reagenzien und Bedingungen:
Oxidation: Häufige Oxidationsmittel sind Kaliumpermanganat und Chromtrioxid.
Reduktion: Reduktionsmittel wie Natriumborhydrid und Lithiumaluminiumhydrid werden verwendet.
Substitution: Die Bedingungen variieren je nach einzuführendem Substituenten, beinhalten aber oft die Verwendung von Katalysatoren und spezifischen Lösungsmitteln.
Hauptprodukte: Die bei diesen Reaktionen gebildeten Hauptprodukte hängen von den spezifischen Bedingungen und Reagenzien ab, die verwendet werden. Beispielsweise kann die Oxidation von ent-Prostaglandin E2 zur Bildung von Ketonen führen, während die Reduktion Hydroxyl-Derivate ergeben kann.
Vergleich Mit ähnlichen Verbindungen
Prostaglandin E2: The naturally occurring form with similar biological activities.
Prostaglandin F2α: Another prostaglandin with distinct roles in smooth muscle contraction and reproductive processes.
Prostaglandin D2: Involved in allergic reactions and sleep regulation.
Uniqueness: ent-Prostaglandin E2 is unique due to its specific stereochemistry, which can result in different biological activities compared to its naturally occurring counterpart. This makes it valuable for studying the effects of stereochemistry on prostaglandin function and for developing targeted therapeutic agents .
Eigenschaften
IUPAC Name |
(Z)-7-[(1S,2S,3S)-3-hydroxy-2-[(E,3R)-3-hydroxyoct-1-enyl]-5-oxocyclopentyl]hept-5-enoic acid | |
---|---|---|
Source | PubChem | |
URL | https://pubchem.ncbi.nlm.nih.gov | |
Description | Data deposited in or computed by PubChem | |
InChI |
InChI=1S/C20H32O5/c1-2-3-6-9-15(21)12-13-17-16(18(22)14-19(17)23)10-7-4-5-8-11-20(24)25/h4,7,12-13,15-17,19,21,23H,2-3,5-6,8-11,14H2,1H3,(H,24,25)/b7-4-,13-12+/t15-,16+,17+,19+/m1/s1 | |
Source | PubChem | |
URL | https://pubchem.ncbi.nlm.nih.gov | |
Description | Data deposited in or computed by PubChem | |
InChI Key |
XEYBRNLFEZDVAW-OBUVHCMGSA-N | |
Source | PubChem | |
URL | https://pubchem.ncbi.nlm.nih.gov | |
Description | Data deposited in or computed by PubChem | |
Canonical SMILES |
CCCCCC(C=CC1C(CC(=O)C1CC=CCCCC(=O)O)O)O | |
Source | PubChem | |
URL | https://pubchem.ncbi.nlm.nih.gov | |
Description | Data deposited in or computed by PubChem | |
Isomeric SMILES |
CCCCC[C@H](/C=C/[C@@H]1[C@H](CC(=O)[C@H]1C/C=C\CCCC(=O)O)O)O | |
Source | PubChem | |
URL | https://pubchem.ncbi.nlm.nih.gov | |
Description | Data deposited in or computed by PubChem | |
Molecular Formula |
C20H32O5 | |
Source | PubChem | |
URL | https://pubchem.ncbi.nlm.nih.gov | |
Description | Data deposited in or computed by PubChem | |
Molecular Weight |
352.5 g/mol | |
Source | PubChem | |
URL | https://pubchem.ncbi.nlm.nih.gov | |
Description | Data deposited in or computed by PubChem | |
Retrosynthesis Analysis
AI-Powered Synthesis Planning: Our tool employs the Template_relevance Pistachio, Template_relevance Bkms_metabolic, Template_relevance Pistachio_ringbreaker, Template_relevance Reaxys, Template_relevance Reaxys_biocatalysis model, leveraging a vast database of chemical reactions to predict feasible synthetic routes.
One-Step Synthesis Focus: Specifically designed for one-step synthesis, it provides concise and direct routes for your target compounds, streamlining the synthesis process.
Accurate Predictions: Utilizing the extensive PISTACHIO, BKMS_METABOLIC, PISTACHIO_RINGBREAKER, REAXYS, REAXYS_BIOCATALYSIS database, our tool offers high-accuracy predictions, reflecting the latest in chemical research and data.
Strategy Settings
Precursor scoring | Relevance Heuristic |
---|---|
Min. plausibility | 0.01 |
Model | Template_relevance |
Template Set | Pistachio/Bkms_metabolic/Pistachio_ringbreaker/Reaxys/Reaxys_biocatalysis |
Top-N result to add to graph | 6 |
Feasible Synthetic Routes
Q1: What is the significance of developing a chiral HPLC method for separating ent-Prostaglandin E2 enantiomers?
A1: [] Chiral HPLC separation is crucial for obtaining enantiomerically pure ent-Prostaglandin E2 ((+)-2), which is essential for several reasons. Firstly, it allows for the verification of enantiopurity in synthetically produced Prostaglandin E2. This is important because different enantiomers of a molecule can have different biological activities. Secondly, having pure ent-Prostaglandin E2 as an analytical standard enables researchers to study the oxidation processes of Prostaglandin E2 in vivo and potentially understand its metabolic pathways. []
Q2: How does the luteolytic potential of ent-Prostaglandin E2 compare to other prostaglandin analogues?
A2: While the provided abstracts don't directly compare the luteolytic activity of ent-Prostaglandin E2 to other analogues, they do highlight the effects of similar compounds. Notably, PGF2alpha-1,15-lactone, 11alpha(15S)-17-phenyl-18,19,20-trinor-ent-Prostaglandin E2 methyl ester, and 17-phenyl-18,19,20-trinor-PGF2alpha all demonstrated luteolytic potential in chacma baboons. [] Further research is needed to directly compare the potency and efficacy of ent-Prostaglandin E2 in this context.
Haftungsausschluss und Informationen zu In-Vitro-Forschungsprodukten
Bitte beachten Sie, dass alle Artikel und Produktinformationen, die auf BenchChem präsentiert werden, ausschließlich zu Informationszwecken bestimmt sind. Die auf BenchChem zum Kauf angebotenen Produkte sind speziell für In-vitro-Studien konzipiert, die außerhalb lebender Organismen durchgeführt werden. In-vitro-Studien, abgeleitet von dem lateinischen Begriff "in Glas", beinhalten Experimente, die in kontrollierten Laborumgebungen unter Verwendung von Zellen oder Geweben durchgeführt werden. Es ist wichtig zu beachten, dass diese Produkte nicht als Arzneimittel oder Medikamente eingestuft sind und keine Zulassung der FDA für die Vorbeugung, Behandlung oder Heilung von medizinischen Zuständen, Beschwerden oder Krankheiten erhalten haben. Wir müssen betonen, dass jede Form der körperlichen Einführung dieser Produkte in Menschen oder Tiere gesetzlich strikt untersagt ist. Es ist unerlässlich, sich an diese Richtlinien zu halten, um die Einhaltung rechtlicher und ethischer Standards in Forschung und Experiment zu gewährleisten.