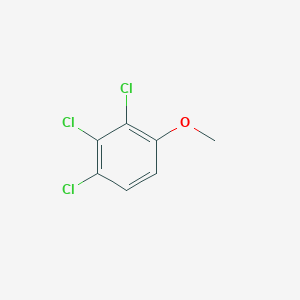
2,3,4-Trichloroanisole
Übersicht
Beschreibung
2,3,4-Trichloroanisole is an organic compound with the molecular formula C7H5Cl3O. It is a derivative of anisole, where three hydrogen atoms on the benzene ring are replaced by chlorine atoms. This compound is known for its role in causing musty odors in various products, including wine and food, due to its low sensory threshold.
Vorbereitungsmethoden
Synthetic Routes and Reaction Conditions: 2,3,4-Trichloroanisole can be synthesized through the methylation of 2,3,4-trichlorophenol. The reaction typically involves the use of a methylating agent such as dimethyl sulfate or methyl iodide in the presence of a base like sodium hydroxide. The reaction conditions usually require a controlled temperature to ensure the selective formation of the desired product.
Industrial Production Methods: In industrial settings, the production of this compound often involves the chlorination of anisole followed by purification processes to isolate the compound. The chlorination step can be carried out using chlorine gas or other chlorinating agents under controlled conditions to achieve the desired substitution pattern.
Analyse Chemischer Reaktionen
Types of Reactions: 2,3,4-Trichloroanisole undergoes various chemical reactions, including:
Substitution Reactions: The chlorine atoms on the benzene ring can be substituted by other nucleophiles under appropriate conditions.
Oxidation Reactions: The methoxy group can be oxidized to form corresponding phenolic compounds.
Reduction Reactions: The chlorine atoms can be reduced to form less chlorinated derivatives.
Common Reagents and Conditions:
Substitution: Reagents like sodium hydroxide or potassium hydroxide in the presence of heat can facilitate nucleophilic substitution.
Oxidation: Oxidizing agents such as potassium permanganate or chromium trioxide can be used.
Reduction: Reducing agents like lithium aluminum hydride or hydrogen gas in the presence of a catalyst can be employed.
Major Products Formed:
Substitution: Formation of various substituted anisoles depending on the nucleophile used.
Oxidation: Formation of trichlorophenol derivatives.
Reduction: Formation of less chlorinated anisoles or anisole itself.
Wissenschaftliche Forschungsanwendungen
Chemical Properties and Formation
TCA is formed through the chlorination of anisole or from precursors like 2,4,6-trichlorophenol (TCP) via microbial O-methylation. The compound is characterized by its potent off-flavor profile, which can significantly affect the quality of various food products. Understanding its chemical behavior is crucial for developing effective detection and remediation strategies.
Detection Techniques
The detection of TCA in food and beverages is critical due to its low sensory threshold. Various analytical methods have been developed:
- Gas Chromatography-Mass Spectrometry (GC-MS) : This method has been widely used for quantifying TCA levels in wine and other products. For instance, studies have shown that TCA can be detected at concentrations as low as 0.03 ng/L using advanced GC-MS techniques .
- Thermal Desorption Techniques : Recent studies have utilized thermal desorption to analyze TCA released from cork materials, confirming its presence and quantifying its concentration effectively .
- Vocus CI-MS : This method allows for rapid screening of TCA in various matrices with high sensitivity and specificity .
Impact on Sensory Perception
TCA is notorious for causing "cork taint" in wines, characterized by a wet cardboard or moldy flavor. Research indicates that even trace amounts of TCA can significantly alter the sensory profile of beverages:
- Threshold Levels : The perception thresholds for TCA vary between 0.03 to 1–2 ng/L, indicating its high potency as an off-flavor compound .
- Mechanism of Action : TCA suppresses olfactory transduction channels in sensory cells, affecting the ability to perceive other flavors. Studies suggest that it inhibits cyclic nucleotide-gated channels at extremely low concentrations (as low as 1 aM) .
Case Studies
Several case studies highlight the implications of TCA in various industries:
- Wine Industry : A comprehensive study detailed the formation pathways of TCA in wines and proposed mitigation strategies such as using alternative cork materials or treatments to remove TCA from contaminated wines . The study emphasized the need for effective monitoring techniques to ensure product quality.
- Coffee Production : Research has shown that TCA can also affect coffee quality, with specific screening methods developed to assess its presence in green coffee beans . The findings indicate that TCA levels can correlate with sensory defects in brewed coffee.
- Food Safety : A multi-residue profiling study demonstrated the occurrence of TCA across various food products, underscoring the importance of monitoring this compound not only in beverages but also in other consumables .
Mitigation Strategies
To address the challenges posed by TCA contamination:
- Material Selection : Using synthetic corks or alternative sealing methods can help prevent TCA ingress into wine.
- Decontamination Techniques : Advanced filtration and adsorption methods are being explored to remove TCA from contaminated products effectively.
- Regulatory Standards : Establishing stringent limits for acceptable TCA levels in food products can help safeguard consumer health and product integrity.
Wirkmechanismus
The primary mechanism by which 2,3,4-Trichloroanisole exerts its effects is through its interaction with olfactory receptors. It is known to attenuate olfactory transduction by suppressing cyclic nucleotide-gated channels, which leads to the characteristic musty odor. This interaction occurs at very low concentrations, making it a potent odorant.
Vergleich Mit ähnlichen Verbindungen
2,4,6-Trichloroanisole: Another chlorinated anisole known for causing cork taint in wine.
2,3,6-Trichloroanisole: Similar in structure but with different chlorine substitution pattern.
2,4,6-Tribromoanisole: A brominated analog with similar sensory properties.
Uniqueness: 2,3,4-Trichloroanisole is unique due to its specific chlorine substitution pattern, which influences its chemical reactivity and sensory properties. Its low sensory threshold makes it particularly significant in the context of odor contamination.
Biologische Aktivität
2,3,4-Trichloroanisole (TCA) is a chlorinated aromatic compound that has garnered attention due to its significant impact on the sensory qualities of various food and beverage products, particularly in wine and coffee. The compound is primarily known for its role as an off-flavor agent, leading to what is commonly referred to as "cork taint" in wines. This article explores the biological activity of this compound, focusing on its effects on sensory perception, potential toxicity, and mechanisms of action.
This compound is a derivative of anisole with three chlorine substituents at the 2, 3, and 4 positions. Its chemical structure can be represented as follows:
Sensory Effects
The primary biological activity of this compound is its ability to suppress olfactory signals. Research has shown that TCA can inhibit ciliary transduction channels in olfactory receptor neurons. This suppression occurs even at extremely low concentrations (as low as 1 aM), suggesting a high potency as an olfactory suppressor . The mechanism involves the partitioning of TCA into the lipid bilayer of plasma membranes, which affects the function of cyclic nucleotide-gated channels critical for olfactory signal transduction .
Table 1: Sensory Thresholds and Effects of TCA
Compound | Detection Threshold (ng/L) | Sensory Effect |
---|---|---|
This compound | 2-7.9 | Suppresses olfactory perception |
2,4,6-Trichloroanisole | Varies | Causes musty odor in wine |
Formation and Sources
TCA is formed through microbial processes involving chlorinated phenolic compounds. Fungi such as Penicillium and Aspergillus are known to produce TCA during their metabolic processes when exposed to chlorinated precursors . The presence of TCA in food products often results from contamination during production or storage.
Case Studies
- Wine Industry : A study conducted on various wine closures demonstrated that natural corks tend to retain TCA more effectively than synthetic alternatives. In tests where wines were spiked with TCA and stored under controlled conditions, natural corks showed a higher retention rate of TCA over time compared to synthetic corks .
- Coffee Contamination : Research into green coffee beans revealed that TCA could be detected at significant levels in beans exposed to contaminated storage environments. This contamination led to off-flavors that impacted consumer acceptance .
Remediation Techniques
Efforts to mitigate the effects of TCA in food products have included various remediation techniques:
- Plasma Activated Water : Recent studies have shown that treating contaminated corks with plasma-activated water can effectively reduce TCA levels by over 72% .
- Molecular Imprinted Polymers (MIPs) : MIPs have demonstrated efficacy in selectively binding and removing TCA from contaminated wines with recovery rates up to 99% .
Table 2: Remediation Techniques for TCA
Technique | Effectiveness (%) | Application Area |
---|---|---|
Plasma Activated Water | >72 | Cork decontamination |
Molecular Imprinted Polymers | 99 | Wine treatment |
Fibrafix TX-R Filters | <1.2 | Wine filtration |
Eigenschaften
IUPAC Name |
1,2,3-trichloro-4-methoxybenzene | |
---|---|---|
Source | PubChem | |
URL | https://pubchem.ncbi.nlm.nih.gov | |
Description | Data deposited in or computed by PubChem | |
InChI |
InChI=1S/C7H5Cl3O/c1-11-5-3-2-4(8)6(9)7(5)10/h2-3H,1H3 | |
Source | PubChem | |
URL | https://pubchem.ncbi.nlm.nih.gov | |
Description | Data deposited in or computed by PubChem | |
InChI Key |
FRQUNVLMWIYOLV-UHFFFAOYSA-N | |
Source | PubChem | |
URL | https://pubchem.ncbi.nlm.nih.gov | |
Description | Data deposited in or computed by PubChem | |
Canonical SMILES |
COC1=C(C(=C(C=C1)Cl)Cl)Cl | |
Source | PubChem | |
URL | https://pubchem.ncbi.nlm.nih.gov | |
Description | Data deposited in or computed by PubChem | |
Molecular Formula |
C7H5Cl3O | |
Source | PubChem | |
URL | https://pubchem.ncbi.nlm.nih.gov | |
Description | Data deposited in or computed by PubChem | |
DSSTOX Substance ID |
DTXSID10202477 | |
Record name | 2,3,4-Trichloroanisole | |
Source | EPA DSSTox | |
URL | https://comptox.epa.gov/dashboard/DTXSID10202477 | |
Description | DSSTox provides a high quality public chemistry resource for supporting improved predictive toxicology. | |
Molecular Weight |
211.5 g/mol | |
Source | PubChem | |
URL | https://pubchem.ncbi.nlm.nih.gov | |
Description | Data deposited in or computed by PubChem | |
CAS No. |
54135-80-7 | |
Record name | 2,3,4-Trichloroanisole | |
Source | CAS Common Chemistry | |
URL | https://commonchemistry.cas.org/detail?cas_rn=54135-80-7 | |
Description | CAS Common Chemistry is an open community resource for accessing chemical information. Nearly 500,000 chemical substances from CAS REGISTRY cover areas of community interest, including common and frequently regulated chemicals, and those relevant to high school and undergraduate chemistry classes. This chemical information, curated by our expert scientists, is provided in alignment with our mission as a division of the American Chemical Society. | |
Explanation | The data from CAS Common Chemistry is provided under a CC-BY-NC 4.0 license, unless otherwise stated. | |
Record name | 2,3,4-Trichloroanisole | |
Source | ChemIDplus | |
URL | https://pubchem.ncbi.nlm.nih.gov/substance/?source=chemidplus&sourceid=0054135807 | |
Description | ChemIDplus is a free, web search system that provides access to the structure and nomenclature authority files used for the identification of chemical substances cited in National Library of Medicine (NLM) databases, including the TOXNET system. | |
Record name | 2,3,4-Trichloroanisole | |
Source | EPA DSSTox | |
URL | https://comptox.epa.gov/dashboard/DTXSID10202477 | |
Description | DSSTox provides a high quality public chemistry resource for supporting improved predictive toxicology. | |
Record name | 2,3,4-trichloroanisole | |
Source | European Chemicals Agency (ECHA) | |
URL | https://echa.europa.eu/substance-information/-/substanceinfo/100.053.610 | |
Description | The European Chemicals Agency (ECHA) is an agency of the European Union which is the driving force among regulatory authorities in implementing the EU's groundbreaking chemicals legislation for the benefit of human health and the environment as well as for innovation and competitiveness. | |
Explanation | Use of the information, documents and data from the ECHA website is subject to the terms and conditions of this Legal Notice, and subject to other binding limitations provided for under applicable law, the information, documents and data made available on the ECHA website may be reproduced, distributed and/or used, totally or in part, for non-commercial purposes provided that ECHA is acknowledged as the source: "Source: European Chemicals Agency, http://echa.europa.eu/". Such acknowledgement must be included in each copy of the material. ECHA permits and encourages organisations and individuals to create links to the ECHA website under the following cumulative conditions: Links can only be made to webpages that provide a link to the Legal Notice page. | |
Record name | 2,3,4-TRICHLOROANISOLE | |
Source | FDA Global Substance Registration System (GSRS) | |
URL | https://gsrs.ncats.nih.gov/ginas/app/beta/substances/FT5R63843S | |
Description | The FDA Global Substance Registration System (GSRS) enables the efficient and accurate exchange of information on what substances are in regulated products. Instead of relying on names, which vary across regulatory domains, countries, and regions, the GSRS knowledge base makes it possible for substances to be defined by standardized, scientific descriptions. | |
Explanation | Unless otherwise noted, the contents of the FDA website (www.fda.gov), both text and graphics, are not copyrighted. They are in the public domain and may be republished, reprinted and otherwise used freely by anyone without the need to obtain permission from FDA. Credit to the U.S. Food and Drug Administration as the source is appreciated but not required. | |
Retrosynthesis Analysis
AI-Powered Synthesis Planning: Our tool employs the Template_relevance Pistachio, Template_relevance Bkms_metabolic, Template_relevance Pistachio_ringbreaker, Template_relevance Reaxys, Template_relevance Reaxys_biocatalysis model, leveraging a vast database of chemical reactions to predict feasible synthetic routes.
One-Step Synthesis Focus: Specifically designed for one-step synthesis, it provides concise and direct routes for your target compounds, streamlining the synthesis process.
Accurate Predictions: Utilizing the extensive PISTACHIO, BKMS_METABOLIC, PISTACHIO_RINGBREAKER, REAXYS, REAXYS_BIOCATALYSIS database, our tool offers high-accuracy predictions, reflecting the latest in chemical research and data.
Strategy Settings
Precursor scoring | Relevance Heuristic |
---|---|
Min. plausibility | 0.01 |
Model | Template_relevance |
Template Set | Pistachio/Bkms_metabolic/Pistachio_ringbreaker/Reaxys/Reaxys_biocatalysis |
Top-N result to add to graph | 6 |
Feasible Synthetic Routes
Q1: What makes 2,3,4-Trichloroanisole so important for researchers studying drinking water quality?
A1: this compound, along with other chloroanisoles, is a significant contributor to unpleasant earthy-musty odors in drinking water, even at extremely low concentrations. [, , , ] This can lead to consumer complaints and perceptions of unsafe water despite its relatively harmless nature at these levels. Understanding the presence, formation, and removal of this compound is crucial for ensuring palatable and high-quality drinking water.
Q2: How can we accurately measure the low levels of this compound found in water?
A2: Several analytical techniques have been developed to quantify trace amounts of this compound in water samples. Some of the commonly employed methods include:
- Headspace Solid Phase Microextraction (HS-SPME) coupled with Gas Chromatography-Mass Spectrometry (GC-MS): This method offers high sensitivity and allows for the simultaneous determination of multiple odorous compounds, including this compound, at nanogram-per-liter levels. [, ]
- Stir Bar Sorptive Extraction (SBSE) followed by Thermal Desorption (TD) and GC-MS: This technique provides efficient extraction and high sensitivity, making it suitable for analyzing a large number of samples in a short period. [, ]
Q3: How does the structure of this compound relate to its properties?
A3: this compound's structure has been investigated using Chlorine Nuclear Quadrupole Resonance (NQR) spectroscopy. [] This technique provides information about the chlorine atoms within the molecule, offering insights into its electronic environment and interactions with surrounding molecules. These structural features likely contribute to its volatility and its affinity for specific extraction phases used in analytical techniques.
Q4: Can you provide the molecular formula and weight of this compound?
A4: The molecular formula for this compound is C₇H₅Cl₃O. It has a molecular weight of 209.48 g/mol.
Q5: Is this compound only found in drinking water?
A5: No, this compound is not limited to drinking water. Research shows it can be found in various environments and matrices:
- Water Supply Reservoirs: Studies have investigated its occurrence and distribution in subtropical water supply reservoirs, highlighting its potential impact on drinking water sources. []
- Food Packaging Materials: The migration of this compound from multilayer plastic-paper food packaging materials has been studied, suggesting a potential source of contamination in food products. []
- Cork Products: Researchers have explored methods for analyzing this compound and other cork-taint compounds in bottled wines, demonstrating its relevance to the beverage industry. []
Q6: How is this compound formed in drinking water?
A6: While this compound itself is not a direct byproduct of water treatment, its precursor, 2,4,6-Trichlorophenol, can be formed during chlorine disinfection. [, ] This precursor can then be converted to this compound through microbial methylation processes, either during water treatment or within the distribution system. [, ]
Q7: What methods are used to remove this compound during drinking water treatment?
A7: Various treatment processes have been investigated for their effectiveness in removing this compound and other odorous compounds from drinking water. These include:
- Conventional Treatment: Research suggests that conventional treatment processes might not always be sufficient to entirely eliminate this compound and other taste and odor compounds. [, ]
- Advanced Treatment Technologies: Further research is necessary to assess the efficacy of advanced treatment technologies specifically targeting this compound and similar compounds. []
Q8: Is there any relationship between this compound and its isomer, 2,4,6-Trichloroanisole?
A8: While both this compound and 2,4,6-Trichloroanisole belong to the chloroanisole family and share a similar musty odor profile, they have distinct chemical structures due to the different positions of the chlorine atoms on the aromatic ring. [, ] This structural difference might lead to variations in their odor thresholds, environmental fate, and response to treatment processes.
Q9: Are there any known studies on the bioaccumulation of this compound in aquatic organisms?
A9: While the provided research papers do not specifically address bioaccumulation of this compound, a related study investigated the bioconcentration of various halogenated aromatics, including its isomer 2,3,6-Trichloroanisole, in rainbow trout. [] This research suggests that halogenated aromatics can accumulate in aquatic organisms, raising concerns about potential ecological impacts.
Haftungsausschluss und Informationen zu In-Vitro-Forschungsprodukten
Bitte beachten Sie, dass alle Artikel und Produktinformationen, die auf BenchChem präsentiert werden, ausschließlich zu Informationszwecken bestimmt sind. Die auf BenchChem zum Kauf angebotenen Produkte sind speziell für In-vitro-Studien konzipiert, die außerhalb lebender Organismen durchgeführt werden. In-vitro-Studien, abgeleitet von dem lateinischen Begriff "in Glas", beinhalten Experimente, die in kontrollierten Laborumgebungen unter Verwendung von Zellen oder Geweben durchgeführt werden. Es ist wichtig zu beachten, dass diese Produkte nicht als Arzneimittel oder Medikamente eingestuft sind und keine Zulassung der FDA für die Vorbeugung, Behandlung oder Heilung von medizinischen Zuständen, Beschwerden oder Krankheiten erhalten haben. Wir müssen betonen, dass jede Form der körperlichen Einführung dieser Produkte in Menschen oder Tiere gesetzlich strikt untersagt ist. Es ist unerlässlich, sich an diese Richtlinien zu halten, um die Einhaltung rechtlicher und ethischer Standards in Forschung und Experiment zu gewährleisten.