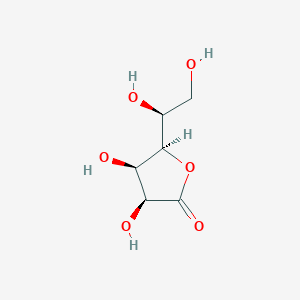
L-Gulonolactone
Ăbersicht
Beschreibung
L-Gulonolactone (CâHââOâ; CAS 1128-23-0) is a cyclic keto-hexose and a critical precursor in the biosynthesis of L-ascorbic acid (vitamin C) in most vertebrates . It is synthesized from D-glucose via a multi-step pathway involving oxidation and lactonization. However, humans and certain primates lack the enzyme this compound oxidase (GULO), rendering them unable to convert this compound to vitamin C, necessitating dietary intake . Structurally, this compound features a lactone ring and five hydroxyl groups but lacks the enediol configuration (âCOH=COH) essential for antioxidant activity .
Vorbereitungsmethoden
Enzymatic Biosynthesis of L-Gulonolactone
Source Organisms and Enzyme Isolation
This compound biosynthesis is predominantly mediated by GULO, a flavin adenine dinucleotide (FAD)-dependent enzyme. Early studies identified GULO activity in the fruit bodies of Grifola frondosa (maitake mushroom), where a 766-fold purification was achieved through a seven-step protocol involving ammonium sulfate fractionation, ion-exchange chromatography, and gel filtration . The purified enzyme exhibited a molecular mass of 250 kDa via gel filtration, with subunits of 69 kDa observed under denaturing conditions . Similar enzymatic activity was detected in the brain and kidney of the African lungfish (Protopterus annectens), where GULO expression persists despite the organismâs inability to synthesize vitamin C de novo .
Substrate Specificity and Catalytic Mechanisms
GULO demonstrates stringent substrate specificity, preferentially oxidizing L-gulono-1,4-lactone (24 ± 1 mM K<sub>m</sub>) over structurally similar aldono-1,4-lactones . Comparative analyses of ancestral GULO enzymes resurrected from chordates and mammals revealed a conserved catalytic mechanism involving hydride transfer from the substrateâs C2 position to FADâs N5 atom, followed by oxygen-dependent reoxidation of the flavin cofactor . Stopped-flow kinetics of mammalian GULO (mGULO) indicated a reductive half-reaction rate (k<sub>red</sub>) of 89.8 ± 2.5 s<sup>â1</sup> for L-gulono-1,4-lactone, with oxidative half-reaction rates (k<sub>ox</sub>) limited by oxygen availability .
Table 1: Kinetic Parameters of Selected Aldonolactone Oxidoreductases
Purification Strategies for GULO and this compound
Multi-Step Chromatographic Purification
The purification of GULO from G. frondosa involves sequential steps:
-
Homogenization : Fresh fruit bodies are homogenized in buffer (pH 7.0) containing protease inhibitors.
-
Ammonium Sulfate Precipitation : Proteins are fractionated at 30â60% saturation.
-
Ion-Exchange Chromatography : DEAE-cellulose columns elute GULO activity at 0.2 M NaCl.
-
Hydroxyapatite Chromatography : Further purification achieves 90% homogeneity.
-
Gel Filtration : Sephacryl S-300 resolves the native enzyme (250 kDa) .
This protocol yields a 14% recovery of enzyme activity, with a specific activity of 1.2 ”mol·min<sup>â1</sup>·mg<sup>â1</sup> .
Recombinant Enzyme Production
Recent advances utilize recombinant DNA technology to express ancestral GULOs in Escherichia coli. Membrane-associated enzymes (e.g., mammalian GULO) require extraction with 0.5% Triton X-100, followed by nickel-affinity chromatography using Ni Sepharose Excel resin . The covalent FAD linkage (8α-N1-histidyl) in GULO necessitates in-gel fluorescence assays for cofactor verification .
Table 2: Purification Summary of G. frondosa GULO
Purification Step | Total Protein (mg) | Total Activity (U) | Specific Activity (U/mg) | Purification (Fold) | Yield (%) |
---|---|---|---|---|---|
Crude Extract | 12,500 | 1,890 | 0.15 | 1 | 100 |
Final Preparation | 22 | 265 | 12.0 | 766 | 14 |
Structural and Functional Insights into GULO
Flavin Binding and Oxygen Reactivity
GULOâs FAD cofactor is covalently bound via an 8α-N1-histidyl linkage, a feature shared with fungal D-arabinonolactone oxidase (ALO) . This covalent attachment stabilizes the flavin during catalytic turnover, particularly under oxidative stress. Structural studies of ancestral GULOs reveal a conserved active site cleft on the si side of the isoalloxazine ring, accommodating L-gulono-1,4-lactoneâs C6 dihydroxyethyl moiety .
Evolutionary Adaptations in Substrate Recognition
Challenges and Innovations in this compound Production
Enzyme Instability and Membrane Association
GULOâs membrane association complicates purification, necessitating detergent solubilization (e.g., 0.05% Triton X-100) . Ancestral GULOs exhibit enhanced thermal stability (T<sub>m</sub> = 66â80°C), enabling their use in high-temperature bioprocessing .
Alternative Biosynthetic Pathways
Non-deuterostome organisms, such as Caenorhabditis elegans and Drosophila melanogaster, synthesize L-ascorbate via GULO-independent pathways, possibly involving symbiotic bacteria . These systems offer novel routes for heterologous this compound production but remain underexplored.
Analyse Chemischer Reaktionen
Arten von Reaktionen: L-Gulono-1,4-lacton durchlÀuft verschiedene Arten von chemischen Reaktionen, darunter:
Oxidation: Es wird von L-Gulono-1,4-lacton-Oxidoreduktase oxidiert, um L-AscorbinsÀure zu produzieren.
Reduktion: Es kann unter bestimmten Bedingungen zu L-GulonsÀure reduziert werden.
Substitution: Es kann an Substitutionsreaktionen teilnehmen, bei denen Hydroxylgruppen durch andere funktionelle Gruppen ersetzt werden.
HĂ€ufige Reagenzien und Bedingungen:
Oxidation: HĂ€ufige Oxidationsmittel umfassen Sauerstoff und 2,6-Dichlorindophenol.
Reduktion: Reduktionsmittel wie Natriumborhydrid können verwendet werden.
Substitution: Verschiedene Reagenzien, darunter SÀuren und Basen, können Substitutionsreaktionen fördern.
Hauptprodukte:
Oxidation: Das Hauptprodukt ist L-AscorbinsÀure.
Reduktion: Das Hauptprodukt ist L-GulonsÀure.
Substitution: Die Produkte variieren abhĂ€ngig von den eingefĂŒhrten Substituenten.
Wissenschaftliche Forschungsanwendungen
Biosynthesis of Ascorbic Acid
L-Gulonolactone as a Precursor:
this compound is a key substrate in the biosynthetic pathway leading to ascorbic acid. The enzyme GULO catalyzes the final step in this pathway, converting L-GulL into ascorbic acid. This process is vital for many organisms, particularly those unable to synthesize vitamin C due to genetic mutations affecting GULO expression .
Enzymatic Activity:
Research has demonstrated that recombinant forms of GULO can exhibit enzymatic activity, suggesting potential applications in biotechnology for producing vitamin C from glucose or other substrates. For instance, endothelial cells can be stimulated to synthesize ascorbic acid intracellularly by expressing GULO and providing L-GulL .
Nutritional Applications
Dietary Supplementation:
this compound is explored as a dietary supplement to enhance vitamin C levels in individuals with limited dietary intake. Its supplementation could help manage conditions related to vitamin C deficiency, such as scurvy and oxidative stress-related disorders .
Food Industry:
In food technology, this compound is being investigated for its potential use as a natural preservative due to its antioxidant properties. Its ability to stabilize ascorbic acid can help prolong the shelf life of various food products .
Medicinal Research
Antioxidant Properties:
Ascorbic acid, derived from this compound, is well-known for its antioxidant capabilities. Research indicates that it plays a protective role against oxidative stress by scavenging reactive oxygen species (ROS) and may reduce the risk of chronic diseases such as cancer and cardiovascular diseases .
Therapeutic Uses:
Studies have shown that vitamin C can enhance the efficacy of certain chemotherapeutic agents and may mitigate their side effects. This has led to interest in using this compound in therapeutic protocols aimed at improving cancer treatment outcomes through enhanced vitamin C production .
Genetic Studies
Gene Expression Profiling:
Research involving gene expression profiling has revealed insights into how the deletion of the GULO gene affects metabolic pathways in various organisms. Such studies provide a deeper understanding of vitamin C metabolism and its implications for health and disease management .
Animal Models:
Animal studies have been instrumental in elucidating the physiological roles of this compound and its derivatives. For example, mouse models lacking the GULO gene have been used to study the consequences of vitamin C deficiency on overall health and gene expression profiles .
Wirkmechanismus
L-Gulono-1,4-lactone exerts its effects primarily through its conversion to L-ascorbic acid by the enzyme L-gulono-1,4-lactone oxidoreductase . This enzyme catalyzes the oxidation of L-Gulono-1,4-lactone, resulting in the formation of L-ascorbic acid, which is a potent antioxidant. The molecular targets and pathways involved include the reduction of reactive oxygen species and the regeneration of other antioxidants .
Vergleich Mit Àhnlichen Verbindungen
L-Ascorbic Acid (Vitamin C)
- Structural Differences: L-Ascorbic acid contains an unsaturated Îł-lactone ring with an enediol group, enabling redox activity, whereas L-gulonolactone lacks this conjugated system .
Dehydro-L-Ascorbic Acid (DHA)
- Oxidized Form : DHA is the oxidized derivative of vitamin C, retaining partial antioxidant activity.
- Bioactivity: In endothelial cells, DHA increases ionomycin-stimulated citrulline and cGMP production by 62â73%, outperforming this compound but underperforming ascorbic acid .
- Stability: DHA is less stable than this compound in aqueous solutions, limiting its therapeutic applications .
Ascorbate 2-Phosphate and Ascorbate 2-Sulfate
- Derivatives : These analogs feature phosphate or sulfate substitutions on the enediol lactone ring.
- Activity : Ascorbate 2-phosphate mimics ascorbic acidâs effects (100% citrulline/cGMP enhancement), while ascorbate 2-sulfate shows only 20â27% efficacy, highlighting the importance of the unmodified enediol structure .
- Applications: Ascorbate 2-phosphate is used in cell culture for its stability, whereas this compound is primarily a research intermediate .
D-Gluconolactone
- Pathway Role: A precursor in the gluconate pathway, D-gluconolactone (CAS 90-80-2) shares the molecular formula CâHââOâ with this compound but differs in lactone ring position (1,5 vs. 1,4) .
- Enzymatic Conversion: In rats, D-glucuronolactone is converted to this compound, but this pathway is absent in humans and guinea pigs due to missing enzymes .
- Industrial Use: D-Gluconolactone is utilized as a food acidulant (E575), contrasting with this compoundâs niche role in vitamin C research .
Data Tables
Table 1: Structural and Functional Comparison
Table 2: Enzymatic and Species-Specific Activity
Species | GULO Expression | This compound Conversion Efficiency |
---|---|---|
Rat | Liver | 95â100% |
Human | Non-functional | 0% |
Guinea Pig | Non-functional | 0% |
Chicken (Gallus) | Kidney | 70â85% |
Research Findings and Implications
- Enzyme Dependency: GULOâs presence dictates this compoundâs utility. Transgenic organisms (e.g., GULO-expressing Bacillus subtilis) can bypass human GULO deficiency, offering aquaculture and nutraceutical applications .
- Therapeutic Potential: this compoundâs safety profile (LDâ â > 5,000 mg/kg in rats) supports its use as a stabilizer or excipient, though it cannot replace ascorbic acid in antioxidant therapies .
- Evolutionary Context: GULO gene loss in primates (~60 million years ago) underscores this compoundâs vestigial role in humans, contrasting with its centrality in most vertebrates .
Biologische AktivitÀt
L-Gulonolactone (L-Gul) is a significant compound in the biosynthesis of L-ascorbic acid (vitamin C) through its role as a substrate for the enzyme L-gulono-Îł-lactone oxidase (GULO). This article delves into the biological activity of this compound, highlighting its enzymatic roles, physiological implications, and research findings.
1. Enzymatic Role in Vitamin C Biosynthesis
L-Gul is a crucial intermediate in the pathway that synthesizes vitamin C, particularly in species capable of producing it. The conversion of this compound to ascorbic acid is catalyzed by GULO, which is the final step in this biosynthetic pathway. In mammals, GULO activity is absent in certain species, including humans and guinea pigs, leading to a reliance on dietary sources of vitamin C.
Table 1: Comparison of GULO Activity Across Species
Species | GULO Activity | Vitamin C Synthesis Capability |
---|---|---|
Humans | Absent | No |
Guinea Pigs | Absent | No |
Mice | Present | Yes |
Rats | Present | Yes |
Bats | Absent | No |
Zebrafish | Present | Yes |
2. Physiological Implications
The absence of GULO and subsequent inability to synthesize vitamin C has significant physiological implications. Vitamin C plays vital roles in antioxidant defense, collagen synthesis, and immune function. In species lacking GULO, such as humans, scurvy can occur due to insufficient vitamin C intake.
Case Study: Scurvy in Pigs
Recent studies have shown that certain pig breeds exhibit mutations leading to the loss of GULO activity. These pigs have been documented to develop scurvy-like symptoms when deprived of dietary vitamin C, demonstrating the critical role of this compound in maintaining ascorbate levels .
3. Research Findings on this compound Activity
Research has demonstrated that this compound not only serves as a substrate for GULO but also exhibits antioxidant properties. Studies indicate that L-Gul can scavenge free radicals, potentially contributing to cellular protection against oxidative stress.
Table 2: Antioxidant Properties of this compound
Study Reference | Antioxidant Activity Observed | Methodology |
---|---|---|
Yes | In vitro assays | |
Yes | Cellular models | |
Yes | Animal studies |
4. Genetic Studies and Evolutionary Perspectives
The evolutionary loss of GULO activity in certain mammals has been a topic of interest. Genetic studies reveal that mutations in the GULO gene have rendered it non-functional in humans and some other species. This phenomenon highlights an evolutionary trade-off where dietary sources became sufficient for vitamin C intake .
Evolutionary Implications
The loss of GULO activity is believed to be linked to dietary shifts during evolution. For instance, species that adapted to diets rich in fruits and vegetables may have lost the necessity for endogenous vitamin C synthesis.
5. Conclusion
This compound plays a pivotal role in the biosynthesis of vitamin C and exhibits significant biological activity through its antioxidant properties. Understanding its enzymatic functions and physiological implications is crucial for further research into nutritional requirements and metabolic pathways across different species.
This comprehensive overview underscores the importance of this compound not only as a biochemical precursor but also as a compound with potential health benefits due to its antioxidant capabilities. Further studies are warranted to explore its applications in nutrition and medicine.
Q & A
Basic Research Questions
Q. What are the established laboratory synthesis pathways for L-Gulonolactone, and what are their key intermediates?
this compound is synthesized from D-glucose via a multi-step process:
- Step 1 : Oxidation of D-glucose to D-gluconic acid.
- Step 2 : Conversion to D-glucono-1,5-lactone.
- Step 3 : Reduction to L-gluconic acid.
- Step 4 : Dehydration to L-glucono-1,4-lactone.
- Step 5 : Oxidation to yield this compound . Key intermediates include D-glucono-1,5-lactone and L-glucono-1,4-lactone. Researchers should monitor reaction conditions (e.g., pH, temperature) to optimize yields.
Q. What analytical techniques are most reliable for characterizing this compound purity and structure?
- HPLC : High sensitivity for quantification in biological matrices .
- NMR Spectroscopy : Confirms structural integrity, particularly the lactone ring and hydroxyl groups .
- FT-IR : Identifies functional groups (e.g., carbonyl groups in the lactone ring) .
- GC : Useful for volatile derivatives but requires derivatization .
Q. Why is this compound absent in humans, and what evolutionary implications does this have?
Humans lack the enzyme this compound oxidase, which converts this compound to vitamin C. This deficiency stems from a genetic mutation ~60 million years ago in primates, leading to reliance on dietary vitamin C. Comparative genomic studies highlight pseudogenization of the GULO gene in humans .
Advanced Research Questions
Q. How can bioinformatics tools like BLAST resolve contradictions in the evolutionary conservation of this compound-related enzymes?
- Use BLASTp to compare this compound oxidase (GULO) sequences across species. For example:
- E-value thresholds : <1eâ»âŽ indicates significant homology .
- Score metrics : Higher scores (>200 bits) suggest conserved enzymatic domains .
Q. What experimental strategies address the cost inefficiency of this compound synthesis?
- Enzymatic catalysis : Use recombinant gulonolactone dehydrogenase to bypass multi-step chemical synthesis .
- Alternative substrates : Test cheaper precursors (e.g., L-sorbose) via microbial fermentation .
- Process optimization : Apply continuous-flow reactors to reduce purification steps and improve scalability .
Q. How can researchers assess the long-term toxicity of this compound in preclinical models?
- In vitro models : Use hepatocyte cell lines to evaluate metabolic stability and oxidative stress markers (e.g., glutathione depletion) .
- In vivo studies : Conduct chronic dosing in GULOâ»/â» knockout mice, monitoring renal function and histopathology .
- Omics approaches : Proteomics and metabolomics can identify off-target effects in vitamin C-dependent pathways .
Q. What role does this compound play in non-vitamin C biosynthetic pathways, such as zinc homeostasis?
- Zinc-dependent gluconolactonase activity : this compound interacts with senescence marker protein-30 (SMP-30) to regulate zinc levels, influencing cellular aging and apoptosis .
- Experimental validation : Knockdown SMP-30 in cell cultures and quantify zinc flux via inductively coupled plasma mass spectrometry (ICP-MS) .
Q. How does this compound modulate the gut microbiome, and what methodologies are suitable for probing this interaction?
- Metagenomic sequencing : Profile microbial communities in this compound-supplemented vs. control cohorts (e.g., 16S rRNA sequencing) .
- Short-chain fatty acid (SCFA) analysis : Use GC-MS to measure butyrate/propionate levels, linking this compound metabolism to prebiotic effects .
Q. Methodological Considerations
Q. What protocols ensure reproducibility in this compound-based antioxidant assays?
- Standardize oxygen radical absorbance capacity (ORAC) assays with Trolox equivalents.
- Control for pH (6â8) and temperature (25°C) to prevent lactone ring hydrolysis .
Q. How can isotopic labeling resolve discrepancies in this compound metabolic flux studies?
- Use ÂčÂłC-labeled this compound in tracer experiments, followed by LC-MS/MS to track incorporation into vitamin C or urinary metabolites .
Q. Emerging Research Directions
Q. Can this compound enhance iron bioavailability in anemia models, and what experimental designs validate this?
- Iron absorption assays : Co-administer this compound with ferric citrate in Caco-2 cell monolayers, quantifying ferritin levels via ELISA .
- In vivo validation : Use iron-deficient rat models to measure hemoglobin recovery rates .
Q. What novel drug delivery systems improve this compound stability in therapeutic applications?
Eigenschaften
IUPAC Name |
(3S,4R,5R)-5-[(1S)-1,2-dihydroxyethyl]-3,4-dihydroxyoxolan-2-one | |
---|---|---|
Source | PubChem | |
URL | https://pubchem.ncbi.nlm.nih.gov | |
Description | Data deposited in or computed by PubChem | |
InChI |
InChI=1S/C6H10O6/c7-1-2(8)5-3(9)4(10)6(11)12-5/h2-5,7-10H,1H2/t2-,3+,4-,5+/m0/s1 | |
Source | PubChem | |
URL | https://pubchem.ncbi.nlm.nih.gov | |
Description | Data deposited in or computed by PubChem | |
InChI Key |
SXZYCXMUPBBULW-SKNVOMKLSA-N | |
Source | PubChem | |
URL | https://pubchem.ncbi.nlm.nih.gov | |
Description | Data deposited in or computed by PubChem | |
Canonical SMILES |
C(C(C1C(C(C(=O)O1)O)O)O)O | |
Source | PubChem | |
URL | https://pubchem.ncbi.nlm.nih.gov | |
Description | Data deposited in or computed by PubChem | |
Isomeric SMILES |
C([C@@H]([C@@H]1[C@@H]([C@@H](C(=O)O1)O)O)O)O | |
Source | PubChem | |
URL | https://pubchem.ncbi.nlm.nih.gov | |
Description | Data deposited in or computed by PubChem | |
Molecular Formula |
C6H10O6 | |
Source | PubChem | |
URL | https://pubchem.ncbi.nlm.nih.gov | |
Description | Data deposited in or computed by PubChem | |
DSSTOX Substance ID |
DTXSID00331407 | |
Record name | L-Gulonolactone | |
Source | EPA DSSTox | |
URL | https://comptox.epa.gov/dashboard/DTXSID00331407 | |
Description | DSSTox provides a high quality public chemistry resource for supporting improved predictive toxicology. | |
Molecular Weight |
178.14 g/mol | |
Source | PubChem | |
URL | https://pubchem.ncbi.nlm.nih.gov | |
Description | Data deposited in or computed by PubChem | |
Physical Description |
Solid | |
Record name | L-Gulonolactone | |
Source | Human Metabolome Database (HMDB) | |
URL | http://www.hmdb.ca/metabolites/HMDB0003466 | |
Description | The Human Metabolome Database (HMDB) is a freely available electronic database containing detailed information about small molecule metabolites found in the human body. | |
Explanation | HMDB is offered to the public as a freely available resource. Use and re-distribution of the data, in whole or in part, for commercial purposes requires explicit permission of the authors and explicit acknowledgment of the source material (HMDB) and the original publication (see the HMDB citing page). We ask that users who download significant portions of the database cite the HMDB paper in any resulting publications. | |
CAS No. |
1128-23-0 | |
Record name | Reduced ascorbate | |
Source | CAS Common Chemistry | |
URL | https://commonchemistry.cas.org/detail?cas_rn=1128-23-0 | |
Description | CAS Common Chemistry is an open community resource for accessing chemical information. Nearly 500,000 chemical substances from CAS REGISTRY cover areas of community interest, including common and frequently regulated chemicals, and those relevant to high school and undergraduate chemistry classes. This chemical information, curated by our expert scientists, is provided in alignment with our mission as a division of the American Chemical Society. | |
Explanation | The data from CAS Common Chemistry is provided under a CC-BY-NC 4.0 license, unless otherwise stated. | |
Record name | L-Gulono-gamma-lactone | |
Source | ChemIDplus | |
URL | https://pubchem.ncbi.nlm.nih.gov/substance/?source=chemidplus&sourceid=0001128230 | |
Description | ChemIDplus is a free, web search system that provides access to the structure and nomenclature authority files used for the identification of chemical substances cited in National Library of Medicine (NLM) databases, including the TOXNET system. | |
Record name | L-Gulonolactone | |
Source | EPA DSSTox | |
URL | https://comptox.epa.gov/dashboard/DTXSID00331407 | |
Description | DSSTox provides a high quality public chemistry resource for supporting improved predictive toxicology. | |
Record name | L-GULONO-.GAMMA.-LACTONE | |
Source | FDA Global Substance Registration System (GSRS) | |
URL | https://gsrs.ncats.nih.gov/ginas/app/beta/substances/56830VTX9G | |
Description | The FDA Global Substance Registration System (GSRS) enables the efficient and accurate exchange of information on what substances are in regulated products. Instead of relying on names, which vary across regulatory domains, countries, and regions, the GSRS knowledge base makes it possible for substances to be defined by standardized, scientific descriptions. | |
Explanation | Unless otherwise noted, the contents of the FDA website (www.fda.gov), both text and graphics, are not copyrighted. They are in the public domain and may be republished, reprinted and otherwise used freely by anyone without the need to obtain permission from FDA. Credit to the U.S. Food and Drug Administration as the source is appreciated but not required. | |
Record name | L-Gulonolactone | |
Source | Human Metabolome Database (HMDB) | |
URL | http://www.hmdb.ca/metabolites/HMDB0003466 | |
Description | The Human Metabolome Database (HMDB) is a freely available electronic database containing detailed information about small molecule metabolites found in the human body. | |
Explanation | HMDB is offered to the public as a freely available resource. Use and re-distribution of the data, in whole or in part, for commercial purposes requires explicit permission of the authors and explicit acknowledgment of the source material (HMDB) and the original publication (see the HMDB citing page). We ask that users who download significant portions of the database cite the HMDB paper in any resulting publications. | |
Synthesis routes and methods
Procedure details
Retrosynthesis Analysis
AI-Powered Synthesis Planning: Our tool employs the Template_relevance Pistachio, Template_relevance Bkms_metabolic, Template_relevance Pistachio_ringbreaker, Template_relevance Reaxys, Template_relevance Reaxys_biocatalysis model, leveraging a vast database of chemical reactions to predict feasible synthetic routes.
One-Step Synthesis Focus: Specifically designed for one-step synthesis, it provides concise and direct routes for your target compounds, streamlining the synthesis process.
Accurate Predictions: Utilizing the extensive PISTACHIO, BKMS_METABOLIC, PISTACHIO_RINGBREAKER, REAXYS, REAXYS_BIOCATALYSIS database, our tool offers high-accuracy predictions, reflecting the latest in chemical research and data.
Strategy Settings
Precursor scoring | Relevance Heuristic |
---|---|
Min. plausibility | 0.01 |
Model | Template_relevance |
Template Set | Pistachio/Bkms_metabolic/Pistachio_ringbreaker/Reaxys/Reaxys_biocatalysis |
Top-N result to add to graph | 6 |
Feasible Synthetic Routes
Haftungsausschluss und Informationen zu In-Vitro-Forschungsprodukten
Bitte beachten Sie, dass alle Artikel und Produktinformationen, die auf BenchChem prĂ€sentiert werden, ausschlieĂlich zu Informationszwecken bestimmt sind. Die auf BenchChem zum Kauf angebotenen Produkte sind speziell fĂŒr In-vitro-Studien konzipiert, die auĂerhalb lebender Organismen durchgefĂŒhrt werden. In-vitro-Studien, abgeleitet von dem lateinischen Begriff "in Glas", beinhalten Experimente, die in kontrollierten Laborumgebungen unter Verwendung von Zellen oder Geweben durchgefĂŒhrt werden. Es ist wichtig zu beachten, dass diese Produkte nicht als Arzneimittel oder Medikamente eingestuft sind und keine Zulassung der FDA fĂŒr die Vorbeugung, Behandlung oder Heilung von medizinischen ZustĂ€nden, Beschwerden oder Krankheiten erhalten haben. Wir mĂŒssen betonen, dass jede Form der körperlichen EinfĂŒhrung dieser Produkte in Menschen oder Tiere gesetzlich strikt untersagt ist. Es ist unerlĂ€sslich, sich an diese Richtlinien zu halten, um die Einhaltung rechtlicher und ethischer Standards in Forschung und Experiment zu gewĂ€hrleisten.