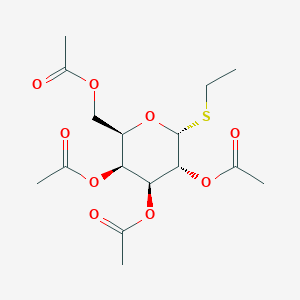
Ethyl-2,3,4,6-Tetra-O-acetyl-α-D-thiogalactopyranosid
- Klicken Sie auf QUICK INQUIRY, um ein Angebot von unserem Expertenteam zu erhalten.
- Mit qualitativ hochwertigen Produkten zu einem WETTBEWERBSFÄHIGEN Preis können Sie sich mehr auf Ihre Forschung konzentrieren.
Übersicht
Beschreibung
Ethly 2,3,4,6-tetra-O-acetyl-a-D-thiogalactopyranoside, also known as Ethly 2,3,4,6-tetra-O-acetyl-a-D-thiogalactopyranoside, is a useful research compound. Its molecular formula is C16H24O9S and its molecular weight is 392.4 g/mol. The purity is usually 95%.
BenchChem offers high-quality Ethly 2,3,4,6-tetra-O-acetyl-a-D-thiogalactopyranoside suitable for many research applications. Different packaging options are available to accommodate customers' requirements. Please inquire for more information about Ethly 2,3,4,6-tetra-O-acetyl-a-D-thiogalactopyranoside including the price, delivery time, and more detailed information at info@benchchem.com.
Wissenschaftliche Forschungsanwendungen
Synthese pharmazeutischer Wirkstoffe
Ethyl-2,3,4,6-Tetra-O-acetyl-α-D-thiogalactopyranosid: ist ein Schlüsselzwischenprodukt bei der Synthese von pharmazeutischen Wirkstoffen . Seine Struktur ermöglicht die Einführung eines Schwefelatoms in Zuckermoleküle, ein entscheidender Schritt bei der Herstellung von Thioglykosiden. Diese Verbindungen werden aufgrund ihrer gegenüber sauerstoffhaltigen Zuckern erhöhten Stabilität häufig in Medikamenten eingesetzt, die eine Reihe von Krankheiten bekämpfen.
Entwicklung enzymatischer Assays
Diese Verbindung dient als Substrat in enzymatischen Assays, insbesondere in solchen, die mit dem Laktose-Stoffwechsel zusammenhängen . Durch die Funktion als Analogon zu natürlichen Zuckern ermöglicht es die Detektion und Quantifizierung von Enzymen, die Laktose verarbeiten, und hilft so bei der Untersuchung von Laktoseintoleranz und der Entwicklung verwandter Behandlungen.
Glykosylierungsreaktionen
In der chemischen Synthese wird This compound in Glykosylierungsreaktionen eingesetzt . Diese Reaktionen sind grundlegend für die Anbindung von Zuckermolekülen an andere Moleküle, was für die Produktion von Glykoproteinen und anderen Glykokonjugaten essenziell ist, die in der Therapie und Forschung eingesetzt werden.
Forschung in der Kohlenhydratchemie
Die Verbindung wird in der Kohlenhydratchemie ausgiebig verwendet, um die Eigenschaften und Reaktivität von Zuckern zu untersuchen . Es liefert Einblicke in die Synthese komplexer Kohlenhydrate und die Entwicklung von Kohlenhydrat-basierten Molekülen mit potenziellen therapeutischen Anwendungen.
Entwicklung biomedizinischer Materialien
Forscher verwenden diese Verbindung bei der Entwicklung biomedizinischer Materialien . Seine Fähigkeit, mit biologischen Systemen zu interagieren, ohne Schaden zu verursachen, macht es zu einem exzellenten Kandidaten für die Herstellung biokompatibler Materialien, die in medizinischen Implantaten oder Medikamenten-Abgabesystemen verwendet werden können.
Analytische Chemie
In der analytischen Chemie wird This compound als Standard- oder Referenzverbindung verwendet . Seine klar definierte Struktur und seine Eigenschaften ermöglichen die Kalibrierung von Instrumenten und die Validierung analytischer Methoden, um die Genauigkeit bei der Messung anderer Substanzen sicherzustellen.
Landwirtschaftliche Forschung
Die Rolle der Verbindung in der landwirtschaftlichen Forschung ist im Entstehen, insbesondere im Studium von Pflanzen-Pathogen-Wechselwirkungen . Seine strukturelle Ähnlichkeit zu natürlichen Pflanzenzuckern macht es zu einem wertvollen Werkzeug, um zu verstehen, wie Krankheitserreger Zuckerrezeptoren auf Pflanzenzellen ausnutzen, was zur Entwicklung krankheitsresistenter Nutzpflanzen führt.
Lebensmittelwissenschaft und -technologie
Schließlich wird diese Verbindung in der Lebensmittelwissenschaft und -technologie auf ihr Potenzial als Lebensmittelzusatzstoff untersucht . Seine Stabilität unter verschiedenen Bedingungen könnte zu Anwendungen bei der Verbesserung der Haltbarkeit von Lebensmitteln oder als Bestandteil in funktionellen Lebensmitteln mit gesundheitlichen Vorteilen führen.
Wirkmechanismus
Target of Action
Ethyl 2,3,4,6-tetra-O-acetyl-a-D-thiogalactopyranoside primarily targets enzymes involved in glycosylation reactions . These enzymes play a crucial role in the metabolism of lactose and other carbohydrates .
Mode of Action
This compound acts as a substrate in enzymatic assays . It interacts with its target enzymes, impeding their activity . This interaction leads to changes in the enzymes’ function, affecting the glycosylation reactions they catalyze .
Biochemical Pathways
The compound affects the biochemical pathways related to carbohydrate metabolism, specifically those involving glycosylation reactions . The downstream effects of this interaction include potential therapeutic interventions against glycosylation-related afflictions like Gaucher’s disease and lysosomal storage disorders .
Result of Action
The molecular and cellular effects of the compound’s action primarily involve the inhibition of glycosidase activity . This inhibition can disrupt normal carbohydrate metabolism, potentially leading to therapeutic effects in the treatment of certain diseases .
Action Environment
Environmental factors such as pH, temperature, and the presence of other molecules can influence the compound’s action, efficacy, and stability. For instance, the compound is sensitive to temperature and should be stored at -20°C . Additionally, it is known to be reactive, and its interactions with other molecules could affect its function .
Eigenschaften
IUPAC Name |
[(2R,3S,4S,5R,6R)-3,4,5-triacetyloxy-6-ethylsulfanyloxan-2-yl]methyl acetate |
Source
|
---|---|---|
Source | PubChem | |
URL | https://pubchem.ncbi.nlm.nih.gov | |
Description | Data deposited in or computed by PubChem | |
InChI |
InChI=1S/C16H24O9S/c1-6-26-16-15(24-11(5)20)14(23-10(4)19)13(22-9(3)18)12(25-16)7-21-8(2)17/h12-16H,6-7H2,1-5H3/t12-,13+,14+,15-,16-/m1/s1 |
Source
|
Source | PubChem | |
URL | https://pubchem.ncbi.nlm.nih.gov | |
Description | Data deposited in or computed by PubChem | |
InChI Key |
YPNFVZQPWZMHIF-LYYZXLFJSA-N |
Source
|
Source | PubChem | |
URL | https://pubchem.ncbi.nlm.nih.gov | |
Description | Data deposited in or computed by PubChem | |
Canonical SMILES |
CCSC1C(C(C(C(O1)COC(=O)C)OC(=O)C)OC(=O)C)OC(=O)C |
Source
|
Source | PubChem | |
URL | https://pubchem.ncbi.nlm.nih.gov | |
Description | Data deposited in or computed by PubChem | |
Isomeric SMILES |
CCS[C@@H]1[C@@H]([C@H]([C@H]([C@H](O1)COC(=O)C)OC(=O)C)OC(=O)C)OC(=O)C |
Source
|
Source | PubChem | |
URL | https://pubchem.ncbi.nlm.nih.gov | |
Description | Data deposited in or computed by PubChem | |
Molecular Formula |
C16H24O9S |
Source
|
Source | PubChem | |
URL | https://pubchem.ncbi.nlm.nih.gov | |
Description | Data deposited in or computed by PubChem | |
Molecular Weight |
392.4 g/mol |
Source
|
Source | PubChem | |
URL | https://pubchem.ncbi.nlm.nih.gov | |
Description | Data deposited in or computed by PubChem | |
Haftungsausschluss und Informationen zu In-Vitro-Forschungsprodukten
Bitte beachten Sie, dass alle Artikel und Produktinformationen, die auf BenchChem präsentiert werden, ausschließlich zu Informationszwecken bestimmt sind. Die auf BenchChem zum Kauf angebotenen Produkte sind speziell für In-vitro-Studien konzipiert, die außerhalb lebender Organismen durchgeführt werden. In-vitro-Studien, abgeleitet von dem lateinischen Begriff "in Glas", beinhalten Experimente, die in kontrollierten Laborumgebungen unter Verwendung von Zellen oder Geweben durchgeführt werden. Es ist wichtig zu beachten, dass diese Produkte nicht als Arzneimittel oder Medikamente eingestuft sind und keine Zulassung der FDA für die Vorbeugung, Behandlung oder Heilung von medizinischen Zuständen, Beschwerden oder Krankheiten erhalten haben. Wir müssen betonen, dass jede Form der körperlichen Einführung dieser Produkte in Menschen oder Tiere gesetzlich strikt untersagt ist. Es ist unerlässlich, sich an diese Richtlinien zu halten, um die Einhaltung rechtlicher und ethischer Standards in Forschung und Experiment zu gewährleisten.