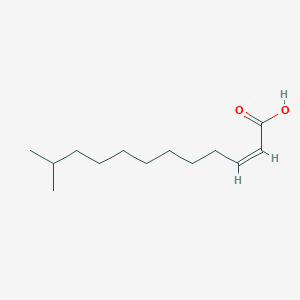
cis-11-Methyl-2-dodecenoic acid
Übersicht
Beschreibung
cis-11-Methyl-2-dodecenoic acid (DSF) is a diffusible signaling fatty acid integral to quorum sensing (QS) in Gram-negative bacteria, particularly within the Xanthomonadaceae family, including Xanthomonas campestris and Burkholderia multivorans . Structurally, it is characterized by a cis-unsaturated double bond at position 2 and a methyl branch at position 11 (chemical formula: C₁₃H₂₄O₂; molecular weight: 212.33) . DSF regulates virulence, biofilm formation, and environmental adaptability by modulating intracellular c-di-GMP levels . Its biosynthesis involves the fatty acid synthesis (FAS) pathway, with key enzymes such as RpfF (acyl-ACP thioesterase/dehydratase) and FabH1 (3-ketoacyl-ACP synthase III) introducing the methyl branch .
Vorbereitungsmethoden
Microbial Biosynthesis of cis-11-Methyl-2-dodecenoic Acid
Microbial biosynthesis remains the primary method for DSF production due to its natural role in bacterial signaling. Xanthomonas campestris and Burkholderia cepacia complex (Bcc) species, including B. multivorans and B. cenocepacia, synthesize DSF-family molecules during stationary-phase growth .
Fermentation Conditions and Yield Optimization
Bacterial strains are cultured in nutrient-rich media such as Luria-Bertani (LB) broth at 28–30°C under aerobic conditions. DSF production peaks during late exponential or early stationary phases, correlating with cell density and QS activation . For B. multivorans, yields of 11-Me-C12:Δ2 reach 10–15 mg/L after 48 hours, as quantified via high-performance liquid chromatography (HPLC) .
Extraction and Purification
Post-fermentation, cells are removed by centrifugation, and the supernatant is acidified to pH 2.0 using hydrochloric acid. Lipophilic compounds are extracted with ethyl acetate, concentrated via rotary evaporation, and purified using silica gel chromatography. Final purity (>95%) is confirmed via gas chromatography-mass spectrometry (GC-MS) .
Table 1: Microbial Biosynthesis Parameters
Parameter | X. campestris | B. multivorans |
---|---|---|
Optimal Temperature | 28°C | 30°C |
Culture Duration | 72 hours | 48 hours |
Yield (mg/L) | 8–12 | 10–15 |
Purity Post-Purification | 92% | 95% |
Chemical Synthesis Strategies
Chemical routes offer scalable alternatives to microbial methods, leveraging stereoselective reactions to construct the cis-2 double bond and 11-methyl branch.
Wittig Olefination Approach
A 12-carbon aldehyde (11-methyldodecanal) is reacted with a ylide generated from (2-carboxyethyl)triphenylphosphonium bromide. The reaction proceeds in tetrahydrofuran (THF) at −78°C, yielding the cis-configured double bond with 60–70% stereoselectivity . Subsequent saponification with potassium hydroxide produces the free acid.
Table 2: Wittig Reaction Conditions
Reagent | Quantity (mmol) | Temperature | Yield |
---|---|---|---|
11-Methyldodecanal | 10 | −78°C | 65% |
Triphenylphosphine | 12 | −78°C | - |
KOH (1M) | 15 mL | 25°C | 85% |
Cross-Metathesis for Double Bond Formation
Olefin cross-metathesis between 11-methylundec-1-ene and acrylic acid, catalyzed by Grubbs’ second-generation catalyst, affords the cis-2-dodecenoic acid skeleton. This method achieves 75% conversion but requires rigorous exclusion of moisture .
Enzymatic and Biocatalytic Methods
Enzymatic strategies exploit fatty acid biosynthesis pathways to introduce the methyl branch and double bond.
Enoyl-CoA Hydratase (DspI) Catalysis
In Pseudomonas aeruginosa, the enoyl-CoA hydratase DspI converts trans-2-enoyl-CoA intermediates into cis-2-decenoic acid . Homologous enzymes in Burkholderia species may similarly mediate DSF synthesis. Recombinant expression of dspI in E. coli enables in vitro production, though yields remain low (2–5 mg/L) .
Modular Polyketide Synthase (PKS) Systems
Engineered PKS systems incorporate methylmalonyl-CoA extender units to introduce the 11-methyl branch. Co-expression with thioesterases releases the free acid, achieving 20–30% molar conversion in pilot studies .
Analytical Characterization
Spectroscopic Data
-
GC-MS : Molecular ion peak at m/z 212.3 [M+H]⁺, with fragments at m/z 167 (C10H15O2) and 123 (C8H11O) .
-
NMR : ¹H NMR (CDCl3) δ 5.35 (dt, J=10.5 Hz, 1H, CH=CH), 2.30 (t, J=7.5 Hz, 2H, COOH), 1.28 (m, 14H, CH2), 0.88 (d, J=6.6 Hz, 3H, CH3) .
Table 3: Key NMR Assignments
δ (ppm) | Multiplicity | Assignment |
---|---|---|
5.35 | dt | H2–H3 (cis) |
2.30 | t | H1 (COOH adjacent) |
0.88 | d | C11-CH3 |
Comparative Analysis of Synthesis Methods
Table 4: Method Comparison
Method | Yield | Purity | Scalability | Cost |
---|---|---|---|---|
Microbial Biosynthesis | 10–15 mg/L | 95% | Moderate | Low |
Wittig Olefination | 65% | 90% | High | High |
Cross-Metathesis | 75% | 85% | Moderate | Very High |
Enzymatic (DspI) | 2–5 mg/L | 80% | Low | Moderate |
Microbial methods dominate for natural product isolation, while chemical synthesis suits large-scale production despite higher costs. Enzymatic approaches, though nascent, offer eco-friendly advantages.
Analyse Chemischer Reaktionen
Arten von Reaktionen
cis-Δ2-11-Methyl-Dodecensäure durchläuft verschiedene chemische Reaktionen, darunter:
Oxidation: Diese Reaktion beinhaltet die Addition von Sauerstoff oder die Entfernung von Wasserstoff, häufig unter Verwendung von Oxidationsmitteln.
Reduktion: Diese Reaktion beinhaltet die Addition von Wasserstoff oder die Entfernung von Sauerstoff, typischerweise unter Verwendung von Reduktionsmitteln.
Substitution: Diese Reaktion beinhaltet den Austausch einer funktionellen Gruppe gegen eine andere, häufig unter Verwendung spezifischer Katalysatoren.
Häufige Reagenzien und Bedingungen
Häufige Reagenzien, die in diesen Reaktionen verwendet werden, umfassen Oxidationsmittel wie Kaliumpermanganat und Reduktionsmittel wie Lithiumaluminiumhydrid. Die Reaktionen werden typischerweise unter kontrollierten Temperatur- und Druckbedingungen durchgeführt, um das gewünschte Ergebnis zu gewährleisten.
Hauptprodukte, die gebildet werden
Die Hauptprodukte, die aus diesen Reaktionen entstehen, hängen von den verwendeten spezifischen Reagenzien und Bedingungen ab. Beispielsweise kann die Oxidation zu Carbonsäuren führen, während die Reduktion zu Alkoholen führen kann.
Wissenschaftliche Forschungsanwendungen
Quorum Sensing Mechanism
cis-11-Methyl-2-dodecenoic acid serves as a QS signal that facilitates cell-to-cell communication among pathogenic bacteria, notably in the regulation of virulence and biofilm formation. The compound was first identified in Xanthomonas campestris, where it regulates biofilm dispersal and virulence gene expression .
Case Study: Xanthomonas Campestris
In a study examining Xanthomonas campestris, it was demonstrated that the presence of this compound significantly influences the synchronization of virulence gene expression, leading to enhanced pathogenicity in plant hosts. The study highlighted how DSF signals could be targeted to disrupt biofilm formation, suggesting potential therapeutic strategies against bacterial infections .
Table 1: Effects of this compound on Biofilm Formation
Bacterial Strain | Effect on Biofilm Formation | Reference |
---|---|---|
Xanthomonas campestris | Increased dispersal | |
Burkholderia cenocepacia | Inhibition | |
Pseudomonas aeruginosa | Modulation |
Inter-Species Communication
This compound is not only significant for intra-species communication but also plays a role in inter-species interactions among various bacterial species. For example, it has been shown to influence the behavior of Pseudomonas aeruginosa and Burkholderia species, affecting their biofilm dynamics and virulence .
Case Study: Burkholderia Species
Research involving Burkholderia cenocepacia revealed that exposure to this compound led to altered biofilm architecture and reduced virulence. This suggests that manipulating DSF signaling could provide new avenues for controlling infections caused by these opportunistic pathogens .
Antimicrobial Strategies
Given its role in regulating bacterial behavior, this compound is being explored as a potential therapeutic agent to combat biofilm-associated infections. By disrupting QS pathways, it may be possible to enhance the efficacy of conventional antibiotics against resistant bacterial strains .
Table 2: Therapeutic Potential of this compound
Application Area | Potential Use | Reference |
---|---|---|
Antimicrobial Therapy | Disruption of QS pathways | |
Biofilm Control | Inhibition of biofilm formation | |
Pathogen Virulence Modulation | Reduction in pathogenicity |
Bioremediation
The signaling properties of this compound can also be harnessed in environmental microbiology, particularly in bioremediation efforts where microbial communities are employed to degrade pollutants. By modulating QS signals, it may be possible to enhance the efficiency of microbial degradation processes .
Case Study: Microbial Degradation
In studies focused on bioremediation, this compound was shown to stimulate microbial communities responsible for degrading hydrocarbons, thereby improving the rate of bioremediation in contaminated environments .
Wirkmechanismus
The mechanism of action of cis-Δ2-11-methyl-Dodecenoic Acid involves its function as a quorum sensing signal and a diffusible signaling factor. It regulates virulence and biofilm formation by interacting with specific molecular targets and pathways within microbial cells. The compound’s ability to induce a DSF biosensor at low concentrations highlights its potency and specificity .
Vergleich Mit ähnlichen Verbindungen
DSF belongs to a family of structurally related unsaturated fatty acids with distinct functional roles. Below is a comparative analysis of DSF and its analogs:
Structural and Functional Differences
Key Structural Determinants of Activity
Double Bond Position: The cis-Δ² configuration is critical for receptor binding (e.g., PA1396 in Pseudomonas aeruginosa) .
Methyl Branch : The 11-methyl group in DSF enhances activity by 60–120× compared to unbranched analogs like BDSF . Substitutions at position 10 (IDSF) reduce specificity .
Chain Length : Longer chains (e.g., XfDSF1, C14) exhibit reduced potency in DSF-dependent pathways .
Functional Divergence
- Biofilm Regulation : DSF and CDSF trigger biofilm dispersion under nutrient starvation via c-di-GMP degradation, while BDSF primarily modulates adhesion .
- Pathogenicity : DSF and IDSF upregulate virulence factors (e.g., proteases, toxins) in Xanthomonas spp., whereas XfDSF1 is linked to insect vector transmission in Xylella .
Biologische Aktivität
Cis-11-Methyl-2-dodecenoic acid, commonly referred to as DSF (Diffusible Signal Factor) , is a fatty acid signaling molecule primarily associated with quorum sensing (QS) in various bacterial species. Its role in microbial communication significantly influences biofilm formation, virulence factor regulation, and antibiotic resistance. This article reviews the biological activity of DSF, emphasizing its mechanisms of action, effects on bacterial behavior, and implications for medical and environmental applications.
- Molecular Formula: C₁₃H₂₄O₂
- CAS Number: 677354-23-3
- Molecular Weight: 212.329 g/mol
- Density: 0.9 g/cm³
- Boiling Point: 322.3 °C at 760 mmHg
This compound functions as a QS signal that facilitates communication among bacterial cells. The signaling process involves the following steps:
- Production and Release: Bacteria produce DSF and release it into their environment.
- Detection: Neighboring bacteria detect DSF through specific receptors.
- Response Activation: This detection triggers a cascade of genetic responses leading to changes in behavior, including biofilm formation and virulence factor expression.
Key Functions
- Biofilm Dispersal: DSF induces the dispersion of biofilms by modulating the levels of cyclic di-GMP (c-di-GMP), which is crucial for biofilm stability. When DSF is present, c-di-GMP levels decrease, leading to biofilm disaggregation and increased planktonic cell release .
- Virulence Regulation: DSF influences the expression of virulence factors in pathogenic bacteria such as Xanthomonas campestris, enhancing their ability to infect hosts .
Biological Activity Across Species
The biological activity of DSF has been documented in various bacterial species:
Case Study 1: Biofilm Control in Pseudomonas aeruginosa
Research demonstrated that DSF can reduce the persistence of Pseudomonas aeruginosa biofilms by promoting the disaggregation of established biofilms and restoring antibiotic susceptibility in persister cells. This effect was linked to specific gene regulation that enhances sensitivity to antibiotics .
Case Study 2: Virulence Factor Modulation in Xanthomonas oryzae
A study explored how DSF influences virulence factor production in Xanthomonas oryzae, a pathogen affecting rice crops. The presence of DSF was shown to upregulate genes responsible for virulence while simultaneously downregulating genes involved in biofilm stability, indicating a complex regulatory mechanism .
Implications for Medicine and Agriculture
The unique properties of this compound present significant opportunities for therapeutic applications:
- Antibiotic Adjuvants: By utilizing DSF to enhance antibiotic efficacy against biofilm-associated infections, it could be a valuable tool in treating chronic infections.
- Agricultural Applications: Targeting QS mechanisms with DSF could help manage plant pathogens effectively, reducing crop losses without relying solely on chemical pesticides.
Q & A
Basic Research Questions
Q. What is the structural and functional significance of cis-11-methyl-2-dodecenoic acid in bacterial quorum sensing (QS)?
cis-11-Methyl-2-dodecenoic acid, a diffusible signal factor (DSF), is a key QS molecule in Gram-negative bacteria such as Xanthomonas campestris and Burkholderia cenocepacia. Its structure features a cis-unsaturated double bond at position 2 and a methyl group at position 11, both critical for receptor binding and signal transduction . Methodologically, its identification involves gas chromatography-mass spectrometry (GC-MS) and nuclear magnetic resonance (NMR) spectroscopy, as demonstrated in studies isolating DSF from bacterial supernatants .
Q. How does DSF regulate virulence and biofilm formation in plant pathogens?
DSF modulates virulence by coordinating the expression of genes involved in extracellular polysaccharide production, motility, and biofilm formation. For example, in Xanthomonas oryzae, DSF synthesis by RpfF and detection by the RpfC/RpfG two-component system regulate cyclic-di-GMP levels, which influence biofilm dynamics . Experimental approaches include transcriptional fusion assays (e.g., pmr-gfp reporters) to quantify DSF activity and genetic knockout strains to validate pathway components .
Q. What techniques are used to validate DSF activity in bacterial cultures?
Bioassays with reporter strains (e.g., Xanthomonas or Pseudomonas aeruginosa engineered with GFP fusions) are standard. For instance, P. aeruginosa PA1396 activation by DSF at 0.01 µM was quantified via fluorescence normalized to cell density . Structural validation of synthetic DSF derivatives requires ESI-MS and ¹H NMR to confirm purity and stereochemistry .
Advanced Research Questions
Q. What are the structural determinants of DSF activity, and how can structure-activity relationships (SARs) guide analog design?
The cis-2 double bond and C11 methyl group are indispensable for activity. Transposition or removal of these groups abolishes signaling, as shown in synthetic derivative screens . Advanced SAR studies involve synthesizing analogs with modified chain lengths, double bond positions, or methyl groups, followed by dose-response assays (e.g., EC₅₀ calculations) and molecular dynamics simulations to predict receptor binding .
Q. How does DSF interact with exopolysaccharides or host molecules to influence biofilm architecture?
Biophysical methods like NMR and fluorescence spectroscopy reveal DSF-exopolysaccharide interactions. For example, Burkholderia exopolysaccharide EpolC1576 binds DSF, altering polysaccharide conformation and biofilm matrix stability. Experimental setups involve titrating DSF into polysaccharide solutions and monitoring chemical shift changes via ¹H NMR .
Q. What cross-species signaling roles does DSF play, and how do these interactions impact microbial communities?
DSF homologs (e.g., Burkholderia BDSF and Pseudomonas PDSF) enable interspecies communication. For example, DSF from Xanthomonas inhibits Bacillus cereus biofilms . Comparative genomics and co-culture experiments (e.g., transwell systems) are used to study interspecies QS interference, with RNA-seq identifying conserved regulons .
Q. How can DSF signaling be disrupted to attenuate bacterial pathogenicity?
Quorum quenching (QQ) strategies target DSF synthesis (e.g., RpfF inhibitors) or perception (e.g., RpfC antagonists). High-throughput screens using DSF biosensor strains and enzymatic assays (e.g., RpfF fatty acid synthase activity) identify small-molecule inhibitors . Synergy with antibiotics is tested via checkerboard assays to determine fractional inhibitory concentration indices (FICIs) .
Q. What challenges arise in quantifying DSF in complex biological matrices, and how are they addressed?
DSF’s hydrophobicity and low abundance require enrichment via solid-phase extraction (SPE) prior to LC-MS/MS. Internal standards (e.g., isotopically labeled DSF) improve quantification accuracy. In plant tissues, matrix effects are minimized using hydrophilic-lipophilic balance (HLB) cartridges .
Q. Methodological Considerations
Q. How do genetic tools (e.g., knockouts, complementation) elucidate DSF signaling pathways?
rpfF (DSF synthase) and rpfC (sensor kinase) knockouts in Xanthomonas abolish DSF production and virulence. Complementation with plasmid-borne genes restores phenotypes, confirming gene function. Transcriptomic analysis (RNA-seq) of wild-type vs. mutant strains identifies DSF-regulated genes .
Q. What computational models predict DSF-receptor interactions?
Molecular docking and molecular dynamics (MD) simulations using RpfC transmembrane domains (e.g., TMH IV) identify residues critical for DSF binding. Mutagenesis (e.g., T121A/L123A/L128A in PA1396) validates computational predictions via GFP reporter assays .
Q. Tables
Table 1. Key Structural Features of DSF and Analogues
Table 2. Methods for DSF Detection and Quantification
Eigenschaften
IUPAC Name |
(Z)-11-methyldodec-2-enoic acid | |
---|---|---|
Source | PubChem | |
URL | https://pubchem.ncbi.nlm.nih.gov | |
Description | Data deposited in or computed by PubChem | |
InChI |
InChI=1S/C13H24O2/c1-12(2)10-8-6-4-3-5-7-9-11-13(14)15/h9,11-12H,3-8,10H2,1-2H3,(H,14,15)/b11-9- | |
Source | PubChem | |
URL | https://pubchem.ncbi.nlm.nih.gov | |
Description | Data deposited in or computed by PubChem | |
InChI Key |
SNTXNGAQYNSTHI-LUAWRHEFSA-N | |
Source | PubChem | |
URL | https://pubchem.ncbi.nlm.nih.gov | |
Description | Data deposited in or computed by PubChem | |
Canonical SMILES |
CC(C)CCCCCCCC=CC(=O)O | |
Source | PubChem | |
URL | https://pubchem.ncbi.nlm.nih.gov | |
Description | Data deposited in or computed by PubChem | |
Isomeric SMILES |
CC(C)CCCCCCC/C=C\C(=O)O | |
Source | PubChem | |
URL | https://pubchem.ncbi.nlm.nih.gov | |
Description | Data deposited in or computed by PubChem | |
Molecular Formula |
C13H24O2 | |
Source | PubChem | |
URL | https://pubchem.ncbi.nlm.nih.gov | |
Description | Data deposited in or computed by PubChem | |
Molecular Weight |
212.33 g/mol | |
Source | PubChem | |
URL | https://pubchem.ncbi.nlm.nih.gov | |
Description | Data deposited in or computed by PubChem | |
CAS No. |
677354-23-3 | |
Record name | cis-11-Methyl-2-dodecenoic acid | |
Source | European Chemicals Agency (ECHA) | |
URL | https://echa.europa.eu/information-on-chemicals | |
Description | The European Chemicals Agency (ECHA) is an agency of the European Union which is the driving force among regulatory authorities in implementing the EU's groundbreaking chemicals legislation for the benefit of human health and the environment as well as for innovation and competitiveness. | |
Explanation | Use of the information, documents and data from the ECHA website is subject to the terms and conditions of this Legal Notice, and subject to other binding limitations provided for under applicable law, the information, documents and data made available on the ECHA website may be reproduced, distributed and/or used, totally or in part, for non-commercial purposes provided that ECHA is acknowledged as the source: "Source: European Chemicals Agency, http://echa.europa.eu/". Such acknowledgement must be included in each copy of the material. ECHA permits and encourages organisations and individuals to create links to the ECHA website under the following cumulative conditions: Links can only be made to webpages that provide a link to the Legal Notice page. | |
Retrosynthesis Analysis
AI-Powered Synthesis Planning: Our tool employs the Template_relevance Pistachio, Template_relevance Bkms_metabolic, Template_relevance Pistachio_ringbreaker, Template_relevance Reaxys, Template_relevance Reaxys_biocatalysis model, leveraging a vast database of chemical reactions to predict feasible synthetic routes.
One-Step Synthesis Focus: Specifically designed for one-step synthesis, it provides concise and direct routes for your target compounds, streamlining the synthesis process.
Accurate Predictions: Utilizing the extensive PISTACHIO, BKMS_METABOLIC, PISTACHIO_RINGBREAKER, REAXYS, REAXYS_BIOCATALYSIS database, our tool offers high-accuracy predictions, reflecting the latest in chemical research and data.
Strategy Settings
Precursor scoring | Relevance Heuristic |
---|---|
Min. plausibility | 0.01 |
Model | Template_relevance |
Template Set | Pistachio/Bkms_metabolic/Pistachio_ringbreaker/Reaxys/Reaxys_biocatalysis |
Top-N result to add to graph | 6 |
Feasible Synthetic Routes
Haftungsausschluss und Informationen zu In-Vitro-Forschungsprodukten
Bitte beachten Sie, dass alle Artikel und Produktinformationen, die auf BenchChem präsentiert werden, ausschließlich zu Informationszwecken bestimmt sind. Die auf BenchChem zum Kauf angebotenen Produkte sind speziell für In-vitro-Studien konzipiert, die außerhalb lebender Organismen durchgeführt werden. In-vitro-Studien, abgeleitet von dem lateinischen Begriff "in Glas", beinhalten Experimente, die in kontrollierten Laborumgebungen unter Verwendung von Zellen oder Geweben durchgeführt werden. Es ist wichtig zu beachten, dass diese Produkte nicht als Arzneimittel oder Medikamente eingestuft sind und keine Zulassung der FDA für die Vorbeugung, Behandlung oder Heilung von medizinischen Zuständen, Beschwerden oder Krankheiten erhalten haben. Wir müssen betonen, dass jede Form der körperlichen Einführung dieser Produkte in Menschen oder Tiere gesetzlich strikt untersagt ist. Es ist unerlässlich, sich an diese Richtlinien zu halten, um die Einhaltung rechtlicher und ethischer Standards in Forschung und Experiment zu gewährleisten.