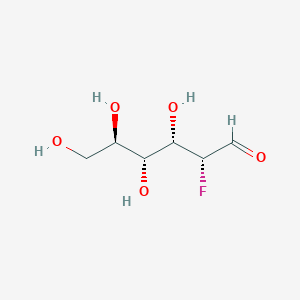
2-Fluor-2-Desoxy-D-Glucose
Übersicht
Beschreibung
Fludeoxyglucose, also known as 2-deoxy-2-fluoro-D-glucose, is a radiopharmaceutical compound used primarily in positron emission tomography (PET) imaging. It is a glucose analog where the hydroxyl group at the C-2 position is replaced by a fluorine-18 atom. This compound is instrumental in diagnosing and monitoring various medical conditions, including cancer, heart disease, and neurological disorders .
Wissenschaftliche Forschungsanwendungen
Fludeoxyglucose has a wide range of applications in scientific research:
Chemistry: Used as a tracer in metabolic studies to understand glucose metabolism.
Biology: Helps in studying cellular processes and metabolic pathways.
Medicine: Essential in PET imaging for diagnosing and monitoring cancer, heart disease, and neurological disorders. .
Industry: Used in the development and testing of new diagnostic imaging techniques and radiopharmaceuticals.
Wirkmechanismus
Target of Action
2-Fluoro-2-deoxy-D-glucose (FDG) is a structural analog of glucose . Its primary targets are cells that have a high rate of glucose consumption, such as brain and heart cells . It is also taken up by cancer cells, which often have an increased rate of glucose metabolism .
Mode of Action
FDG mimics glucose chemically and structurally . It is taken up by cells via the GLUT-1 glucose transporter . Once inside the cell, FDG is phosphorylated by hexokinase to FDG-6-phosphate . This results in the accumulation of FDG-6-phosphate within the cell .
Biochemical Pathways
The primary biochemical pathway affected by FDG is glycolysis . FDG inhibits glycolysis by competing with glucose for uptake and phosphorylation . This can be visualized using positron emission tomography (PET), providing a map of glucose consumption within the body .
Pharmacokinetics
FDG is rapidly taken up by cells with high glucose consumption, such as brain and heart cells
Biochemische Analyse
Biochemical Properties
2-Fluoro-2-deoxy-D-glucose mimics glucose chemically and structurally . It is taken up by cells but does not undergo metabolic glycolysis . It inhibits glycolysis due to the formation and intracellular accumulation of 2-deoxy-D-glucose-6-phosphate , inhibiting the function of hexokinase and glucose-6-phosphate isomerase .
Cellular Effects
The uptake and distribution of 2-Fluoro-2-deoxy-D-glucose are found to be similar to those of glucose in animal models . It is rapidly taken up by brain and heart cells . In cancer cells, the acceleration of glycolytic flux and its independence of oxygen tension have been described .
Molecular Mechanism
2-Fluoro-2-deoxy-D-glucose is taken up by cells via the GLUT-1 glucose transporter and phosphorylated by hexokinase to FDG-6-phosphate . There is no further metabolism, and in most tissues, there is negligible dephosphorylation .
Temporal Effects in Laboratory Settings
The effects of 2-Fluoro-2-deoxy-D-glucose over time in laboratory settings have been studied. For instance, increased radioactivity translocation can be attributed to the high osmotic pressure and ionic strength of the applied FDG and glucose mixture solution .
Dosage Effects in Animal Models
The effects of 2-Fluoro-2-deoxy-D-glucose vary with different dosages in animal models. For instance, the brain SUV was found to be significantly and inversely related to glycemia .
Metabolic Pathways
2-Fluoro-2-deoxy-D-glucose is involved in the glycolysis pathway . It can also enter the pentose phosphate pathway to some extent, where glucose-6-phosphate is converted to ribulose-5-phosphate, generating NADPH .
Transport and Distribution
2-Fluoro-2-deoxy-D-glucose is transported and distributed within cells and tissues. It is taken up by cells via the GLUT-1 glucose transporter .
Subcellular Localization
The subcellular localization of 2-Fluoro-2-deoxy-D-glucose is similar to that of glucose, given its structural similarity. It is taken up by cells and can be found in the cytoplasm .
Vorbereitungsmethoden
Synthetic Routes and Reaction Conditions: Fludeoxyglucose is synthesized through a nucleophilic substitution reaction. The process begins with the production of fluorine-18 in a cyclotron, typically from oxygen-18 enriched water. The fluorine-18 fluoride ion is then reacted with a mannose triflate precursor under basic conditions to produce fludeoxyglucose .
Industrial Production Methods: In industrial settings, the synthesis of fludeoxyglucose involves automated synthesis modules such as the GE FASTLab 2. This system allows for high-yield production with enhanced radiochemical purity. The process includes the use of a cassette-based automated synthesis module, which ensures compliance with current Good Manufacturing Practice (cGMP) and radiation safety standards .
Analyse Chemischer Reaktionen
Types of Reactions: Fludeoxyglucose primarily undergoes phosphorylation reactions in biological systems. It is phosphorylated by hexokinase to form fludeoxyglucose-6-phosphate, which is then trapped within cells due to its inability to undergo further glycolysis .
Common Reagents and Conditions:
Reagents: Fluorine-18 fluoride, mannose triflate precursor, potassium carbonate, kryptofix 2.2.2.
Conditions: The reaction is typically carried out in anhydrous acetonitrile under basic conditions at elevated temperatures.
Major Products: The primary product of the reaction is fludeoxyglucose-6-phosphate, which accumulates in tissues with high glucose metabolism, such as cancer cells .
Vergleich Mit ähnlichen Verbindungen
2-Deoxy-D-glucose: Another glucose analog used in metabolic studies but lacks the radioactive fluorine-18.
Fluorothymidine (FLT): A thymidine analog used in PET imaging to assess cellular proliferation.
Fluoromisonidazole (FMISO): Used in PET imaging to detect hypoxic regions within tumors.
Uniqueness: Fludeoxyglucose is unique due to its incorporation of fluorine-18, which allows for high-resolution PET imaging. Its ability to mimic glucose metabolism makes it an invaluable tool in diagnosing and monitoring various diseases, particularly cancer .
Eigenschaften
IUPAC Name |
(2R,3S,4R,5R)-2-fluoro-3,4,5,6-tetrahydroxyhexanal | |
---|---|---|
Source | PubChem | |
URL | https://pubchem.ncbi.nlm.nih.gov | |
Description | Data deposited in or computed by PubChem | |
InChI |
InChI=1S/C6H11FO5/c7-3(1-8)5(11)6(12)4(10)2-9/h1,3-6,9-12H,2H2/t3-,4+,5+,6+/m0/s1 | |
Source | PubChem | |
URL | https://pubchem.ncbi.nlm.nih.gov | |
Description | Data deposited in or computed by PubChem | |
InChI Key |
AOYNUTHNTBLRMT-SLPGGIOYSA-N | |
Source | PubChem | |
URL | https://pubchem.ncbi.nlm.nih.gov | |
Description | Data deposited in or computed by PubChem | |
Canonical SMILES |
C(C(C(C(C(C=O)F)O)O)O)O | |
Source | PubChem | |
URL | https://pubchem.ncbi.nlm.nih.gov | |
Description | Data deposited in or computed by PubChem | |
Isomeric SMILES |
C([C@H]([C@H]([C@@H]([C@H](C=O)F)O)O)O)O | |
Source | PubChem | |
URL | https://pubchem.ncbi.nlm.nih.gov | |
Description | Data deposited in or computed by PubChem | |
Molecular Formula |
C6H11FO5 | |
Source | PubChem | |
URL | https://pubchem.ncbi.nlm.nih.gov | |
Description | Data deposited in or computed by PubChem | |
DSSTOX Substance ID |
DTXSID701311592 | |
Record name | 2-Fluoro-2-deoxy-D-glucose | |
Source | EPA DSSTox | |
URL | https://comptox.epa.gov/dashboard/DTXSID701311592 | |
Description | DSSTox provides a high quality public chemistry resource for supporting improved predictive toxicology. | |
Molecular Weight |
182.15 g/mol | |
Source | PubChem | |
URL | https://pubchem.ncbi.nlm.nih.gov | |
Description | Data deposited in or computed by PubChem | |
CAS No. |
29702-43-0, 38440-79-8, 86783-82-6 | |
Record name | 2-Fluoro-2-deoxy-D-glucose | |
Source | CAS Common Chemistry | |
URL | https://commonchemistry.cas.org/detail?cas_rn=29702-43-0 | |
Description | CAS Common Chemistry is an open community resource for accessing chemical information. Nearly 500,000 chemical substances from CAS REGISTRY cover areas of community interest, including common and frequently regulated chemicals, and those relevant to high school and undergraduate chemistry classes. This chemical information, curated by our expert scientists, is provided in alignment with our mission as a division of the American Chemical Society. | |
Explanation | The data from CAS Common Chemistry is provided under a CC-BY-NC 4.0 license, unless otherwise stated. | |
Record name | Fludeoxyglucose | |
Source | ChemIDplus | |
URL | https://pubchem.ncbi.nlm.nih.gov/substance/?source=chemidplus&sourceid=0029702430 | |
Description | ChemIDplus is a free, web search system that provides access to the structure and nomenclature authority files used for the identification of chemical substances cited in National Library of Medicine (NLM) databases, including the TOXNET system. | |
Record name | 2-Deoxy-2-fluoromannose | |
Source | ChemIDplus | |
URL | https://pubchem.ncbi.nlm.nih.gov/substance/?source=chemidplus&sourceid=0038440798 | |
Description | ChemIDplus is a free, web search system that provides access to the structure and nomenclature authority files used for the identification of chemical substances cited in National Library of Medicine (NLM) databases, including the TOXNET system. | |
Record name | Fludeoxyglucose | |
Source | DrugBank | |
URL | https://www.drugbank.ca/drugs/DB15107 | |
Description | The DrugBank database is a unique bioinformatics and cheminformatics resource that combines detailed drug (i.e. chemical, pharmacological and pharmaceutical) data with comprehensive drug target (i.e. sequence, structure, and pathway) information. | |
Explanation | Creative Common's Attribution-NonCommercial 4.0 International License (http://creativecommons.org/licenses/by-nc/4.0/legalcode) | |
Record name | 2-Fluoro-2-deoxy-D-glucose | |
Source | EPA DSSTox | |
URL | https://comptox.epa.gov/dashboard/DTXSID701311592 | |
Description | DSSTox provides a high quality public chemistry resource for supporting improved predictive toxicology. | |
Record name | 2-Fluoro-2-deoxy-D-glucose | |
Source | European Chemicals Agency (ECHA) | |
URL | https://echa.europa.eu/information-on-chemicals | |
Description | The European Chemicals Agency (ECHA) is an agency of the European Union which is the driving force among regulatory authorities in implementing the EU's groundbreaking chemicals legislation for the benefit of human health and the environment as well as for innovation and competitiveness. | |
Explanation | Use of the information, documents and data from the ECHA website is subject to the terms and conditions of this Legal Notice, and subject to other binding limitations provided for under applicable law, the information, documents and data made available on the ECHA website may be reproduced, distributed and/or used, totally or in part, for non-commercial purposes provided that ECHA is acknowledged as the source: "Source: European Chemicals Agency, http://echa.europa.eu/". Such acknowledgement must be included in each copy of the material. ECHA permits and encourages organisations and individuals to create links to the ECHA website under the following cumulative conditions: Links can only be made to webpages that provide a link to the Legal Notice page. | |
Record name | 2-FLUORO-2-DEOXY-D-GLUCOSE | |
Source | European Chemicals Agency (ECHA) | |
URL | https://echa.europa.eu/information-on-chemicals | |
Description | The European Chemicals Agency (ECHA) is an agency of the European Union which is the driving force among regulatory authorities in implementing the EU's groundbreaking chemicals legislation for the benefit of human health and the environment as well as for innovation and competitiveness. | |
Explanation | Use of the information, documents and data from the ECHA website is subject to the terms and conditions of this Legal Notice, and subject to other binding limitations provided for under applicable law, the information, documents and data made available on the ECHA website may be reproduced, distributed and/or used, totally or in part, for non-commercial purposes provided that ECHA is acknowledged as the source: "Source: European Chemicals Agency, http://echa.europa.eu/". Such acknowledgement must be included in each copy of the material. ECHA permits and encourages organisations and individuals to create links to the ECHA website under the following cumulative conditions: Links can only be made to webpages that provide a link to the Legal Notice page. | |
Record name | FLUDEOXYGLUCOSE | |
Source | FDA Global Substance Registration System (GSRS) | |
URL | https://gsrs.ncats.nih.gov/ginas/app/beta/substances/ZO2JV75L55 | |
Description | The FDA Global Substance Registration System (GSRS) enables the efficient and accurate exchange of information on what substances are in regulated products. Instead of relying on names, which vary across regulatory domains, countries, and regions, the GSRS knowledge base makes it possible for substances to be defined by standardized, scientific descriptions. | |
Explanation | Unless otherwise noted, the contents of the FDA website (www.fda.gov), both text and graphics, are not copyrighted. They are in the public domain and may be republished, reprinted and otherwise used freely by anyone without the need to obtain permission from FDA. Credit to the U.S. Food and Drug Administration as the source is appreciated but not required. | |
Retrosynthesis Analysis
AI-Powered Synthesis Planning: Our tool employs the Template_relevance Pistachio, Template_relevance Bkms_metabolic, Template_relevance Pistachio_ringbreaker, Template_relevance Reaxys, Template_relevance Reaxys_biocatalysis model, leveraging a vast database of chemical reactions to predict feasible synthetic routes.
One-Step Synthesis Focus: Specifically designed for one-step synthesis, it provides concise and direct routes for your target compounds, streamlining the synthesis process.
Accurate Predictions: Utilizing the extensive PISTACHIO, BKMS_METABOLIC, PISTACHIO_RINGBREAKER, REAXYS, REAXYS_BIOCATALYSIS database, our tool offers high-accuracy predictions, reflecting the latest in chemical research and data.
Strategy Settings
Precursor scoring | Relevance Heuristic |
---|---|
Min. plausibility | 0.01 |
Model | Template_relevance |
Template Set | Pistachio/Bkms_metabolic/Pistachio_ringbreaker/Reaxys/Reaxys_biocatalysis |
Top-N result to add to graph | 6 |
Feasible Synthetic Routes
Haftungsausschluss und Informationen zu In-Vitro-Forschungsprodukten
Bitte beachten Sie, dass alle Artikel und Produktinformationen, die auf BenchChem präsentiert werden, ausschließlich zu Informationszwecken bestimmt sind. Die auf BenchChem zum Kauf angebotenen Produkte sind speziell für In-vitro-Studien konzipiert, die außerhalb lebender Organismen durchgeführt werden. In-vitro-Studien, abgeleitet von dem lateinischen Begriff "in Glas", beinhalten Experimente, die in kontrollierten Laborumgebungen unter Verwendung von Zellen oder Geweben durchgeführt werden. Es ist wichtig zu beachten, dass diese Produkte nicht als Arzneimittel oder Medikamente eingestuft sind und keine Zulassung der FDA für die Vorbeugung, Behandlung oder Heilung von medizinischen Zuständen, Beschwerden oder Krankheiten erhalten haben. Wir müssen betonen, dass jede Form der körperlichen Einführung dieser Produkte in Menschen oder Tiere gesetzlich strikt untersagt ist. Es ist unerlässlich, sich an diese Richtlinien zu halten, um die Einhaltung rechtlicher und ethischer Standards in Forschung und Experiment zu gewährleisten.