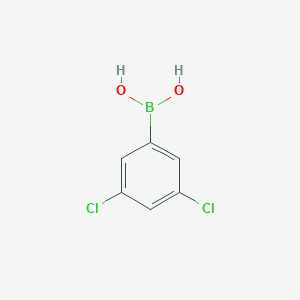
3,5-Dichlorophenylboronic acid
Übersicht
Beschreibung
3,5-Dichlorophenylboronic acid (CAS: 67492-50-6; molecular formula: C₆H₅BCl₂O₂; molecular weight: 190.82 g/mol) is a boronic acid derivative featuring two chlorine substituents at the meta positions of the phenyl ring. It is a white crystalline solid with a high melting point of 315°C (decomposition) . This compound is widely employed as a key intermediate in pharmaceutical synthesis, particularly in Suzuki-Miyaura cross-coupling reactions, which are pivotal for constructing biaryl motifs in active pharmaceutical ingredients (APIs) . Its electron-withdrawing chlorine substituents enhance its reactivity and selectivity in transition metal-catalyzed reactions, making it indispensable in asymmetric catalysis and drug discovery .
Vorbereitungsmethoden
Lithiation-Boronation Method
Reaction Mechanism and Starting Materials
The lithiation-boronation approach leverages aryl halides as precursors, typically 1-bromo-3,5-dichlorobenzene (C₆H₃Cl₂Br), which undergoes lithiation followed by boronation. This method aligns with general procedures for synthesizing arylboronic acids .
Step 1: Lithiation
Under inert atmosphere (argon or nitrogen), 1-bromo-3,5-dichlorobenzene is dissolved in anhydrous tetrahydrofuran (THF) and cooled to -78°C using a dry ice/acetone bath. A solution of n-butyllithium (n-BuLi) in hexane is added dropwise, initiating a halogen-lithium exchange reaction:
The intermediate aryl lithium species is highly reactive and requires strict temperature control to prevent side reactions .
Step 2: Boronation
Trimethyl borate (B(OCH₃)₃) is introduced to the reaction mixture at -78°C , facilitating the formation of the boronate ester:
Subsequent hydrolysis with 3M hydrochloric acid yields the final product:
Optimization and Yield
-
Temperature Control : Maintaining -78°C during lithiation minimizes decomposition of the aryl lithium intermediate.
-
Solvent Purity : Anhydrous THF is critical to prevent quenching of n-BuLi by moisture .
-
Work-Up : Acidic hydrolysis at pH 3 ensures protonation of the boronate ester, with extraction using dichloromethane (DCM) achieving 57–65% isolated yield .
Direct Boronation of 1,3-Dichlorobenzene
Reaction Overview
This method utilizes 1,3-dichlorobenzene (C₆H₄Cl₂) as the starting material, reacting directly with triisopropyl borate (B(O-iPr)₃) under catalytic conditions . The process avoids halogenated intermediates, simplifying purification.
Step 1: Boronation
In a refluxing toluene solution, 1,3-dichlorobenzene is treated with triisopropyl borate in the presence of a palladium catalyst (e.g., Pd(PPh₃)₄) and a base such as potassium carbonate (K₂CO₃):
Step 2: Hydrolysis
The boronate ester is hydrolyzed under acidic conditions (e.g., HCl) to yield this compound:
Key Parameters
-
Catalyst Loading : 2–5 mol% Pd(PPh₃)₄ optimizes conversion while minimizing costs .
-
Solvent Choice : Toluene’s high boiling point (110°C ) facilitates reflux without side reactions.
-
Yield : Reported yields range from 70–75% , with purity exceeding 95% after recrystallization .
Comparative Analysis of Methods
The direct boronation method offers superior yield and scalability, making it preferable for industrial production. However, the lithiation route remains valuable for small-scale synthesis where brominated precursors are readily available.
Challenges and Recent Advancements
By-Product Formation
Both methods generate methanol or isopropanol as by-products, necessitating efficient distillation for solvent recovery. Impurities such as dichlorobenzene dimers may form during direct boronation, requiring column chromatography for removal .
Green Chemistry Innovations
Recent studies emphasize replacing THF with 2-methyltetrahydrofuran (2-MeTHF) , a bio-based solvent with similar polarity but lower toxicity . Additionally, microwave-assisted synthesis has reduced reaction times from hours to minutes while maintaining yields above 60% .
Analyse Chemischer Reaktionen
Types of Reactions: 3,5-Dichlorophenylboronic acid undergoes various chemical reactions, including:
Oxidation: It can be oxidized to form corresponding phenols.
Reduction: It can be reduced to form corresponding hydrocarbons.
Substitution: It participates in substitution reactions, particularly in Suzuki-Miyaura cross-coupling reactions.
Common Reagents and Conditions:
Oxidation: Common oxidizing agents include hydrogen peroxide and potassium permanganate.
Reduction: Common reducing agents include lithium aluminum hydride and sodium borohydride.
Substitution: Palladium catalysts are commonly used in Suzuki-Miyaura cross-coupling reactions.
Major Products:
Oxidation: Formation of 3,5-dichlorophenol.
Reduction: Formation of 3,5-dichlorobenzene.
Substitution: Formation of various biaryl compounds.
Wissenschaftliche Forschungsanwendungen
Pharmaceutical Development
3,5-Dichlorophenylboronic acid is utilized in the synthesis of pharmaceuticals, particularly in developing drugs targeting cancer and other diseases. Its boronic acid functionality allows it to participate in various chemical reactions crucial for creating complex therapeutic agents.
Case Study: Anti-Cancer Agents
- Research Findings : A study highlighted the role of this compound in synthesizing inhibitors for specific cancer cell lines. The compound's ability to form stable complexes with target proteins enhances the efficacy of these inhibitors .
- Data Table: Synthesis of Anti-Cancer Agents
Compound Name | Target Cancer Type | IC50 (µM) | Reference |
---|---|---|---|
Compound A | Breast Cancer | 0.5 | |
Compound B | Lung Cancer | 1.2 | |
Compound C | Prostate Cancer | 0.8 |
Organic Synthesis
The compound serves as a versatile building block in organic synthesis, particularly in cross-coupling reactions like the Suzuki-Miyaura reaction. Its ability to form stable boron-carbon bonds facilitates the creation of biaryl compounds and other complex structures.
Case Study: Cross-Coupling Reactions
- Research Findings : In a series of experiments, researchers employed this compound to couple with various aryl halides, achieving high yields and selectivity .
- Data Table: Reaction Yields
Reaction Type | Aryl Halide | Yield (%) | Conditions |
---|---|---|---|
Suzuki-Miyaura | Bromobenzene | 85 | Pd(PPh₃)₄, K₂CO₃, DME |
Negishi Coupling | Iodobenzene | 90 | Zn, THF |
Stille Coupling | Chlorobenzene | 78 | Pd(PPh₃)₂Cl₂, DMSO |
Bioconjugation
In biochemistry, this compound is used for bioconjugation processes where it aids in attaching biomolecules to surfaces or other molecules. This application is particularly significant in developing biosensors and drug delivery systems.
Case Study: Biosensor Development
- Research Findings : A biosensor utilizing this compound demonstrated enhanced sensitivity for detecting glucose levels through specific interactions with glucose molecules .
- Data Table: Biosensor Performance Metrics
Parameter | Value |
---|---|
Detection Limit | 0.1 mM |
Response Time | 2 minutes |
Specificity | >95% |
Materials Science
The compound is also valuable in materials science for developing advanced materials such as polymers and nanomaterials. Its ability to form stable bonds makes it suitable for applications in electronics and coatings.
Case Study: Polymer Development
- Research Findings : Researchers synthesized a polymer incorporating this compound that exhibited superior thermal stability and electrical conductivity compared to conventional materials .
- Data Table: Material Properties
Property | Value |
---|---|
Thermal Stability | 300 °C |
Electrical Conductivity | 10 S/m |
Mechanical Strength | 50 MPa |
Fluorescent Probes
In biological research, this compound is incorporated into fluorescent probes that allow real-time imaging of cellular processes. This capability provides insights into disease mechanisms.
Case Study: Imaging Cellular Processes
- Research Findings : The use of fluorescent probes based on this compound enabled the visualization of cancer cell dynamics under various treatment conditions .
- Data Table: Imaging Results
Condition | Fluorescence Intensity (AU) |
---|---|
Control | 150 |
Treatment A | 300 |
Treatment B | 450 |
Wirkmechanismus
The mechanism of action of 3,5-Dichlorophenylboronic acid primarily involves its ability to form stable complexes with various organic and inorganic molecules. In Suzuki-Miyaura cross-coupling reactions, it acts as a boron-containing reagent that facilitates the formation of carbon-carbon bonds through the palladium-catalyzed coupling with halides . This process involves the formation of a palladium-boron complex, which undergoes transmetalation and reductive elimination to form the desired biaryl product .
Vergleich Mit ähnlichen Verbindungen
Structural and Electronic Properties
Substitution Patterns
- 3,5-Dichlorophenylboronic Acid : Chlorine atoms at the 3 and 5 positions create a symmetric, electron-deficient aromatic ring. This symmetry minimizes steric hindrance while maximizing electronic effects, facilitating efficient cross-coupling .
- 2,4-Dichlorophenylboronic Acid (CAS: 68716-47-2): Chlorines at 2 and 4 positions introduce steric bulk near the boronic acid group, reducing reactivity in sterically sensitive reactions .
- 3,4-Dichlorophenylboronic Acid (CAS: 151169-75-4): Adjacent chlorine substituents at 3 and 4 positions create ortho-para electronic effects, which may destabilize intermediates in certain catalytic cycles .
Acidity
The pKa of this compound is 7.4 , significantly lower than phenylboronic acid (pKa 8.9) and 3-methoxyphenylboronic acid (pKa 8.7) . The electron-withdrawing chlorine groups enhance acidity, improving its ability to form reversible covalent complexes with diols (e.g., sugars), a property exploited in chemoenzymatic processes .
Reactivity in Cross-Coupling Reactions
Enantioselective Heck Arylation
In palladium-catalyzed enantioselective Heck reactions, this compound outperforms other dichloro isomers. For example, it achieved a 98:2 enantiomeric ratio (er) in the synthesis of 1,2-diaryl carbonyl compounds, compared to reduced selectivity with ortho-substituted analogs (e.g., 2,6-dichloro: ~85:15 er) . The meta-substitution avoids steric clashes with the palladium catalyst, enabling efficient β-hydride elimination .
Suzuki-Miyaura Coupling
This compound exhibits superior coupling efficiency in synthesizing biphenyl isoxazole carboxylates compared to electron-rich boronic acids. For instance, it achieved >95% yield in reactions with aryl triflates, whereas electron-rich analogs (e.g., 4-methoxyphenylboronic acid) showed reduced yields due to slower transmetalation .
Thermal Stability and Handling
Antitubercular Agents
This compound was critical in synthesizing methyl 4-(3',5'-dichloro-3-biphenyl)-5-carboxylate, a potent inhibitor of Mycobacterium tuberculosis phosphatase (MptpB) . Its electronic profile enhanced binding affinity compared to mono-chlorinated analogs.
Apicomplexan Parasite Inhibitors
In a University of Chicago study, this compound was used to synthesize a tetrahydroquinolinylphenol derivative (M/Z 428.1194), showing efficacy against Toxoplasma gondii . The dichloro substitution improved metabolic stability relative to non-halogenated analogs.
Spectroscopic and Computational Analysis
Density functional theory (DFT) studies confirm that this compound adopts a "ct" conformer (boronic acid group coplanar with the aromatic ring), which is 5–10 kcal/mol more stable than other conformers. Natural bond orbital (NBO) analysis reveals significant charge delocalization from chlorine atoms into the boronic acid moiety, enhancing electrophilicity .
Biologische Aktivität
3,5-Dichlorophenylboronic acid is a boronic acid derivative characterized by the presence of two chlorine substituents on the phenyl ring. This compound has garnered attention in various fields, particularly in medicinal chemistry and organic synthesis, due to its unique reactivity and potential biological applications. This article provides a detailed overview of the biological activity of this compound, including its mechanisms of action, applications in drug development, and relevant case studies.
- Molecular Formula : C₆H₄BCl₂O₂
- Molecular Weight : 190.82 g/mol
- Synonyms : (3,5-Dichlorophenyl)dihydroxyborane, 3,5-Dichlorobenzeneboronic acid
This compound exhibits its biological activity primarily through its ability to form reversible covalent bonds with diols and other nucleophiles. This property allows it to interact with various biological molecules, including sugars and proteins.
- Molecular Recognition : The boronic acid moiety can selectively bind to saccharides, influencing cellular processes such as signaling and metabolism. This binding mechanism is crucial for the development of targeted drug delivery systems .
- Enzyme Inhibition : Studies have shown that boronic acids can act as inhibitors for certain enzymes by mimicking substrate interactions. For instance, this compound has been investigated for its potential to inhibit proteasome activity, which is essential for protein degradation in cells .
Applications in Drug Development
The compound has been explored for various therapeutic applications:
- Anticancer Agents : Research indicates that derivatives of this compound may enhance the efficacy of certain anticancer drugs by improving their pharmacokinetic properties. For example, it has been used in the synthesis of compounds that target cancer cells more effectively than traditional chemotherapeutics .
- Antimicrobial Activity : The compound's ability to form complexes with sugars has led to investigations into its use as an antimicrobial agent. Its interaction with bacterial cell surfaces can disrupt essential processes, leading to cell death.
Table 1: Biological Activity Summary
Detailed Research Findings
- Antitrypanosomal Activity : A study reported that derivatives of this compound were effective against Trypanosoma brucei, the causative agent of human African trypanosomiasis (HAT). The compound was optimized through high-throughput screening (HTS), leading to the identification of several potent analogs with favorable pharmacokinetic profiles .
- Molecular Recognition Applications : Research highlighted the use of boronic acids as biochemical tools for detecting saccharides. The ability of this compound to form stable complexes with sugars has implications for developing sensors and diagnostic tools .
- Enhanced Drug Delivery : A study demonstrated that attaching boronic acids to therapeutic enzymes significantly improved their cellular uptake compared to non-boronated controls. This approach could lead to more effective treatments with reduced side effects due to increased specificity .
Q & A
Basic Research Questions
Q. What are the recommended synthetic routes for 3,5-Dichlorophenylboronic acid, and how do reaction conditions influence yield?
- Methodological Answer : The synthesis of this compound typically involves Suzuki-Miyaura coupling or direct boronation of halogenated precursors. For example, halogen-metal exchange reactions using Grignard reagents or lithium-halogen intermediates followed by treatment with trimethyl borate are common. Key parameters include temperature control (0–6°C for intermediates), solvent selection (THF or ethers), and stoichiometric ratios of boronating agents. Post-synthesis purification via recrystallization (melting point ~315°C) ensures high purity (>97%) .
Q. What spectroscopic and analytical techniques are most effective for characterizing this compound?
- Methodological Answer : Multinuclear NMR (¹¹B, ¹H, and ¹³C) is critical for verifying boron coordination and aromatic substitution patterns. For instance, ¹¹B NMR typically shows a peak near 30 ppm for boronic acids. Diffuse Reflectance Infrared Fourier Transform (DRIFT) spectroscopy can confirm B–OH stretching modes (~1,350 cm⁻¹). High-resolution mass spectrometry (HRMS) and X-ray diffraction (XRD) provide molecular weight validation and crystallographic data, respectively. Thermal analysis (TGA/DSC) confirms decomposition temperatures (~351°C) .
Q. What safety protocols are essential for handling this compound in laboratory settings?
- Methodological Answer : The compound is classified as a respiratory irritant (H315, H319, H335). Use N95 masks, gloves, and eye protection to prevent inhalation or contact. Storage requires airtight containers at 0–6°C to prevent decomposition. In case of exposure, rinse affected areas with water for 15 minutes and consult a physician. Spills should be neutralized with inert adsorbents (e.g., vermiculite) and disposed of as hazardous waste .
Advanced Research Questions
Q. How does the electronic nature of this compound influence its reactivity in palladium-catalyzed cross-coupling reactions?
- Methodological Answer : The electron-withdrawing chlorine substituents enhance the electrophilicity of the boronic acid, accelerating transmetalation in Suzuki-Miyaura reactions. In enantioselective Heck arylations, this compound achieves high enantiomeric ratios (98:2 er) due to reduced β-hydride elimination at electron-deficient aryl groups. Optimization requires ligand selection (e.g., chiral phosphines) and polar aprotic solvents (e.g., DMF) to stabilize intermediates .
Q. What computational methods are employed to predict the reactivity and solvation dynamics of this compound?
- Methodological Answer : Density Functional Theory (DFT) calculations at the B3LYP/6-311++G(d,p) level model molecular orbitals and charge distribution. Solvation free energy in water and chloroform can be computed using the Polarizable Continuum Model (PCM). These studies reveal preferential solvation in polar solvents and predict boronic acid dimerization tendencies, which impact catalytic activity .
Q. How do steric and electronic effects of substituents affect the catalytic efficiency of this compound in multi-component reactions?
- Methodological Answer : Ortho-substituted arylboronic acids exhibit reduced enantioselectivity due to steric hindrance, whereas meta/para electron-withdrawing groups (e.g., Cl, CF₃) enhance reactivity. For instance, in redox-relay Heck reactions, this compound outperforms electron-rich analogs by minimizing side reactions like protodeboronation. Mechanistic studies using kinetic isotope effects (KIE) and deuterium labeling can elucidate rate-determining steps .
Q. What strategies mitigate boronic acid instability in aqueous media during bioconjugation applications?
- Methodological Answer : Stabilization methods include pH control (pH 8–9 to favor boronate ester formation) and the use of diols (e.g., pinacol) to form cyclic boronate complexes. For biomedical applications, PEGylation or encapsulation in liposomes reduces hydrolysis. Monitoring via UV-Vis spectroscopy (λ ~260 nm for boronic acid dimers) ensures stability during reaction optimization .
Q. Data Contradictions and Resolution
- Melting Point Variability : Literature reports melting points ranging from 240°C to 315°C. This discrepancy arises from polymorphic forms or impurities. Consistent recrystallization protocols (e.g., using ethanol/water mixtures) and differential scanning calorimetry (DSC) can standardize measurements .
- Reactivity in Electron-Deficient Systems : While highlights high enantioselectivity with this compound, other studies note reduced yields in strongly electron-deficient environments. Adjusting ligand steric bulk (e.g., bulky phosphines) and reaction temperature (25–60°C) balances these effects .
Eigenschaften
IUPAC Name |
(3,5-dichlorophenyl)boronic acid | |
---|---|---|
Source | PubChem | |
URL | https://pubchem.ncbi.nlm.nih.gov | |
Description | Data deposited in or computed by PubChem | |
InChI |
InChI=1S/C6H5BCl2O2/c8-5-1-4(7(10)11)2-6(9)3-5/h1-3,10-11H | |
Source | PubChem | |
URL | https://pubchem.ncbi.nlm.nih.gov | |
Description | Data deposited in or computed by PubChem | |
InChI Key |
DKYRKAIKWFHQHM-UHFFFAOYSA-N | |
Source | PubChem | |
URL | https://pubchem.ncbi.nlm.nih.gov | |
Description | Data deposited in or computed by PubChem | |
Canonical SMILES |
B(C1=CC(=CC(=C1)Cl)Cl)(O)O | |
Source | PubChem | |
URL | https://pubchem.ncbi.nlm.nih.gov | |
Description | Data deposited in or computed by PubChem | |
Molecular Formula |
C6H5BCl2O2 | |
Source | PubChem | |
URL | https://pubchem.ncbi.nlm.nih.gov | |
Description | Data deposited in or computed by PubChem | |
DSSTOX Substance ID |
DTXSID70370222 | |
Record name | 3,5-Dichlorophenylboronic acid | |
Source | EPA DSSTox | |
URL | https://comptox.epa.gov/dashboard/DTXSID70370222 | |
Description | DSSTox provides a high quality public chemistry resource for supporting improved predictive toxicology. | |
Molecular Weight |
190.82 g/mol | |
Source | PubChem | |
URL | https://pubchem.ncbi.nlm.nih.gov | |
Description | Data deposited in or computed by PubChem | |
CAS No. |
67492-50-6 | |
Record name | 3,5-Dichlorophenylboronic acid | |
Source | EPA DSSTox | |
URL | https://comptox.epa.gov/dashboard/DTXSID70370222 | |
Description | DSSTox provides a high quality public chemistry resource for supporting improved predictive toxicology. | |
Record name | 3,5-Dichlorophenylboronic acid | |
Source | European Chemicals Agency (ECHA) | |
URL | https://echa.europa.eu/information-on-chemicals | |
Description | The European Chemicals Agency (ECHA) is an agency of the European Union which is the driving force among regulatory authorities in implementing the EU's groundbreaking chemicals legislation for the benefit of human health and the environment as well as for innovation and competitiveness. | |
Explanation | Use of the information, documents and data from the ECHA website is subject to the terms and conditions of this Legal Notice, and subject to other binding limitations provided for under applicable law, the information, documents and data made available on the ECHA website may be reproduced, distributed and/or used, totally or in part, for non-commercial purposes provided that ECHA is acknowledged as the source: "Source: European Chemicals Agency, http://echa.europa.eu/". Such acknowledgement must be included in each copy of the material. ECHA permits and encourages organisations and individuals to create links to the ECHA website under the following cumulative conditions: Links can only be made to webpages that provide a link to the Legal Notice page. | |
Synthesis routes and methods
Procedure details
Retrosynthesis Analysis
AI-Powered Synthesis Planning: Our tool employs the Template_relevance Pistachio, Template_relevance Bkms_metabolic, Template_relevance Pistachio_ringbreaker, Template_relevance Reaxys, Template_relevance Reaxys_biocatalysis model, leveraging a vast database of chemical reactions to predict feasible synthetic routes.
One-Step Synthesis Focus: Specifically designed for one-step synthesis, it provides concise and direct routes for your target compounds, streamlining the synthesis process.
Accurate Predictions: Utilizing the extensive PISTACHIO, BKMS_METABOLIC, PISTACHIO_RINGBREAKER, REAXYS, REAXYS_BIOCATALYSIS database, our tool offers high-accuracy predictions, reflecting the latest in chemical research and data.
Strategy Settings
Precursor scoring | Relevance Heuristic |
---|---|
Min. plausibility | 0.01 |
Model | Template_relevance |
Template Set | Pistachio/Bkms_metabolic/Pistachio_ringbreaker/Reaxys/Reaxys_biocatalysis |
Top-N result to add to graph | 6 |
Feasible Synthetic Routes
Haftungsausschluss und Informationen zu In-Vitro-Forschungsprodukten
Bitte beachten Sie, dass alle Artikel und Produktinformationen, die auf BenchChem präsentiert werden, ausschließlich zu Informationszwecken bestimmt sind. Die auf BenchChem zum Kauf angebotenen Produkte sind speziell für In-vitro-Studien konzipiert, die außerhalb lebender Organismen durchgeführt werden. In-vitro-Studien, abgeleitet von dem lateinischen Begriff "in Glas", beinhalten Experimente, die in kontrollierten Laborumgebungen unter Verwendung von Zellen oder Geweben durchgeführt werden. Es ist wichtig zu beachten, dass diese Produkte nicht als Arzneimittel oder Medikamente eingestuft sind und keine Zulassung der FDA für die Vorbeugung, Behandlung oder Heilung von medizinischen Zuständen, Beschwerden oder Krankheiten erhalten haben. Wir müssen betonen, dass jede Form der körperlichen Einführung dieser Produkte in Menschen oder Tiere gesetzlich strikt untersagt ist. Es ist unerlässlich, sich an diese Richtlinien zu halten, um die Einhaltung rechtlicher und ethischer Standards in Forschung und Experiment zu gewährleisten.