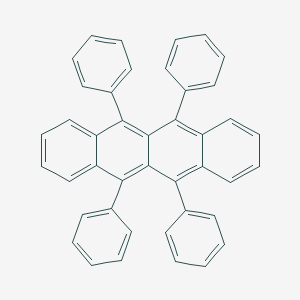
Rubren
Übersicht
Beschreibung
It is well-known for its distinctive optical and electrical properties, making it a significant compound in the field of organic electronics . Rubrene has been extensively studied for its high charge-carrier mobility, which is crucial for applications in organic light-emitting diodes (OLEDs) and organic field-effect transistors (OFETs) .
Wissenschaftliche Forschungsanwendungen
Organic Electronics
Rubrene is primarily utilized in organic field-effect transistors (OFETs) due to its high hole mobility, which can exceed 20 cm²/V·s. This property makes it a suitable candidate for high-performance electronic devices. Recent studies have focused on enhancing the performance of rubrene-based OFETs through surface doping techniques, which optimize the electronic band structure and charge carrier dynamics.
Table 1: Properties of Rubrene in OFETs
Property | Value |
---|---|
Hole Mobility | >20 cm²/V·s |
Bandgap | ~2.5 eV |
Crystal Structure | Face-centered orthorhombic |
Thermal Stability | Stable up to 300 °C |
Case Study : A recent investigation into the surface doping of rubrene single crystals demonstrated that molecular electron donors and acceptors can modify the near-surface charge density while maintaining high charge-carrier mobility, crucial for developing efficient organic electronic devices .
Optoelectronic Devices
Rubrene's photoluminescent properties make it ideal for applications in light-emitting diodes (LEDs) and organic solar cells (OSCs). The material exhibits strong photoluminescence, which is essential for converting electrical energy into light.
Table 2: Optical Properties of Rubrene
Property | Value |
---|---|
Photoluminescence Peak | ~570 nm |
Absorption Spectrum | Broad range (300-600 nm) |
Quantum Efficiency | High (~80%) |
Case Study : Research on rubrene-based organic solar cells has shown that the integration of rubrene can enhance the efficiency of charge separation and transport, leading to improved power conversion efficiencies .
Photonic Applications
Rubrene's unique optical characteristics have led to its use in various photonic applications, including sensors and lasers. Its ability to form thin films with controlled thickness allows for precise manipulation of light.
Case Study : The investigation into the role of molecular conformations in rubrene films revealed that the twisted and planar forms of rubrene significantly influence its optical properties. By adjusting substrate temperatures during film deposition, researchers achieved distinct optical responses that can be harnessed for photonic devices .
Thermal and Mechanical Stability
Research indicates that rubrene exhibits remarkable thermal stability, making it suitable for applications requiring high operational temperatures. Its mechanical properties also allow for flexibility in device fabrication.
Table 3: Thermal Properties of Rubrene
Property | Value |
---|---|
Glass Transition Temp | ~70 °C |
Decomposition Temp | ~300 °C |
Wirkmechanismus
Target of Action
Rubrene, a polycyclic aromatic hydrocarbon, is primarily targeted in the field of organic semiconductors . It is extensively used in the development of organic light-emitting diodes (OLEDs) and organic field-effect transistors . The primary role of rubrene in these applications is to facilitate the movement of charge carriers, contributing to the overall performance of the electronic devices .
Mode of Action
Rubrene interacts with its targets through its distinctive optical and electrical properties . As an organic semiconductor, rubrene exhibits high carrier mobility, reaching 40 cm²/(V·s) for holes . This property allows rubrene to facilitate efficient charge transport in electronic devices . Furthermore, rubrene undergoes redox reactions in solution, oxidizing and reducing reversibly at 0.95 V and -1.37 V, respectively vs SCE .
Biochemical Pathways
Rubrene’s high carrier mobility allows for efficient charge transport, which is crucial for the functioning of organic optoelectronic devices .
Result of Action
The action of rubrene results in enhanced performance of organic electronic devices. Its high carrier mobility allows for efficient charge transport, improving the performance of devices such as OLEDs and organic field-effect transistors . Furthermore, rubrene’s ability to undergo redox reactions contributes to its utility in these applications .
Action Environment
The action of rubrene can be influenced by environmental factors. For instance, the crystal structure of rubrene, which can be monoclinic, triclinic, or orthorhombic, can be influenced by the conditions under which it is grown . These different crystal structures can have varying effects on rubrene’s electronic properties and, consequently, on its performance in electronic devices .
Vorbereitungsmethoden
Rubrene can be synthesized through various methods. One common synthetic route involves treating 1,1,3-triphenyl-2-propyn-1-ol with thionyl chloride. The resulting chloroallene undergoes dimerization and dehydrochlorination to yield rubrene . Another method involves the physical vapor transport (PVT) technique, which is used to grow rubrene crystals. This method involves sublimation and recrystallization under controlled temperature gradients .
Analyse Chemischer Reaktionen
Rubrene undergoes several types of chemical reactions, including oxidation and reduction. It oxidizes and reduces reversibly at 0.95 V and -1.37 V, respectively, versus the standard calomel electrode (SCE) . When both the cation and anion are co-generated in an electrochemical cell, they can combine, resulting in the annihilation of their charges and the production of an excited rubrene molecule that emits at 540 nm . Rubrene also reacts with potassium, leading to the formation of various potassium-intercalated compounds .
Vergleich Mit ähnlichen Verbindungen
Rubrene is often compared to other polycyclic aromatic hydrocarbons, such as tetracene and pentacene. While tetracene and pentacene also exhibit high charge-carrier mobility, rubrene is unique due to its higher stability and distinctive optical properties . Additionally, rubrene’s ability to form various polymorphs, such as orthorhombic, monoclinic, and triclinic crystals, further distinguishes it from similar compounds .
Biologische Aktivität
Rubrene, chemically known as 5,6,11,12-tetraphenyltetracene, is a well-studied organic semiconductor renowned for its significant biological and electronic properties. This article delves into the biological activity of rubrene, highlighting its interactions, applications, and research findings from various studies.
Overview of Rubrene
Rubrene is primarily recognized for its high charge carrier mobility and photoluminescent properties. It has been extensively researched for applications in organic electronics, including organic light-emitting diodes (OLEDs) and organic solar cells. Additionally, rubrene's biological activity has garnered attention in fields such as phototherapy and sensor technology.
Biological Activity and Mechanisms
-
Photophysical Properties :
Rubrene exhibits strong photoluminescence due to its extended π-conjugated system. This property is crucial for applications in optoelectronics and phototherapy. Studies demonstrate that rubrene can generate singlet excitons efficiently, which can lead to applications in photodynamic therapy (PDT) for cancer treatment . -
Charge Transport :
The charge transport properties of rubrene are enhanced by its crystalline structure, which facilitates efficient carrier mobility. Research indicates that the intermolecular interactions in rubrene crystals significantly contribute to its electronic properties. For instance, C...π interactions between neighboring tetracene backbones enhance the stability and mobility of charge carriers . -
Biocompatibility :
Preliminary studies suggest that rubrene exhibits low toxicity levels in biological systems, making it a candidate for biomedical applications. Its compatibility with biological tissues is essential for potential uses in drug delivery systems and biosensing technologies .
1. Photodynamic Therapy (PDT)
A study explored the efficacy of rubrene as a photosensitizer in PDT. The research demonstrated that rubrene could effectively generate reactive oxygen species (ROS) upon light activation, leading to apoptosis in cancer cells. The results indicated a significant reduction in cell viability when treated with rubrene under light exposure compared to controls.
2. Sensor Applications
Rubrene has been utilized in developing chemosensors due to its luminescent properties. A notable study reported the synthesis of rubrene-based sensors capable of detecting hydrogen peroxide levels in biological samples. The sensors exhibited high sensitivity and selectivity, indicating the potential for real-time monitoring of oxidative stress in cells .
Table 1: Summary of Rubrene's Biological Activities
Research Findings
- Liquid Phase Exfoliation : Recent studies have shown that liquid phase exfoliation techniques can produce stable nanostructures of rubrene, enhancing its optical properties while maintaining biocompatibility . This opens avenues for integrating rubrene into flexible electronic devices and biomedical applications.
- Surface Doping Effects : Research on surface doping of rubrene single crystals indicates that doping can modify the electronic band structure without significantly affecting charge carrier mobility. This finding is crucial for optimizing rubrene's performance in electronic devices .
- Molecular Interactions : Investigations into the intermolecular interactions within rubrene crystals reveal that both C...π and H—H interactions play vital roles in stabilizing the crystal structure and enhancing charge transport properties .
Eigenschaften
IUPAC Name |
5,6,11,12-tetraphenyltetracene | |
---|---|---|
Source | PubChem | |
URL | https://pubchem.ncbi.nlm.nih.gov | |
Description | Data deposited in or computed by PubChem | |
InChI |
InChI=1S/C42H28/c1-5-17-29(18-6-1)37-33-25-13-14-26-34(33)39(31-21-9-3-10-22-31)42-40(32-23-11-4-12-24-32)36-28-16-15-27-35(36)38(41(37)42)30-19-7-2-8-20-30/h1-28H | |
Source | PubChem | |
URL | https://pubchem.ncbi.nlm.nih.gov | |
Description | Data deposited in or computed by PubChem | |
InChI Key |
YYMBJDOZVAITBP-UHFFFAOYSA-N | |
Source | PubChem | |
URL | https://pubchem.ncbi.nlm.nih.gov | |
Description | Data deposited in or computed by PubChem | |
Canonical SMILES |
C1=CC=C(C=C1)C2=C3C=CC=CC3=C(C4=C(C5=CC=CC=C5C(=C24)C6=CC=CC=C6)C7=CC=CC=C7)C8=CC=CC=C8 | |
Source | PubChem | |
URL | https://pubchem.ncbi.nlm.nih.gov | |
Description | Data deposited in or computed by PubChem | |
Molecular Formula |
C42H28 | |
Source | PubChem | |
URL | https://pubchem.ncbi.nlm.nih.gov | |
Description | Data deposited in or computed by PubChem | |
DSSTOX Substance ID |
DTXSID8060161 | |
Record name | Naphthacene, 5,6,11,12-tetraphenyl- | |
Source | EPA DSSTox | |
URL | https://comptox.epa.gov/dashboard/DTXSID8060161 | |
Description | DSSTox provides a high quality public chemistry resource for supporting improved predictive toxicology. | |
Molecular Weight |
532.7 g/mol | |
Source | PubChem | |
URL | https://pubchem.ncbi.nlm.nih.gov | |
Description | Data deposited in or computed by PubChem | |
Physical Description |
Dark brown or red powder; [Sigma-Aldrich MSDS] | |
Record name | Rubrene | |
Source | Haz-Map, Information on Hazardous Chemicals and Occupational Diseases | |
URL | https://haz-map.com/Agents/15279 | |
Description | Haz-Map® is an occupational health database designed for health and safety professionals and for consumers seeking information about the adverse effects of workplace exposures to chemical and biological agents. | |
Explanation | Copyright (c) 2022 Haz-Map(R). All rights reserved. Unless otherwise indicated, all materials from Haz-Map are copyrighted by Haz-Map(R). No part of these materials, either text or image may be used for any purpose other than for personal use. Therefore, reproduction, modification, storage in a retrieval system or retransmission, in any form or by any means, electronic, mechanical or otherwise, for reasons other than personal use, is strictly prohibited without prior written permission. | |
CAS No. |
517-51-1 | |
Record name | Rubrene | |
Source | CAS Common Chemistry | |
URL | https://commonchemistry.cas.org/detail?cas_rn=517-51-1 | |
Description | CAS Common Chemistry is an open community resource for accessing chemical information. Nearly 500,000 chemical substances from CAS REGISTRY cover areas of community interest, including common and frequently regulated chemicals, and those relevant to high school and undergraduate chemistry classes. This chemical information, curated by our expert scientists, is provided in alignment with our mission as a division of the American Chemical Society. | |
Explanation | The data from CAS Common Chemistry is provided under a CC-BY-NC 4.0 license, unless otherwise stated. | |
Record name | Rubrene | |
Source | ChemIDplus | |
URL | https://pubchem.ncbi.nlm.nih.gov/substance/?source=chemidplus&sourceid=0000517511 | |
Description | ChemIDplus is a free, web search system that provides access to the structure and nomenclature authority files used for the identification of chemical substances cited in National Library of Medicine (NLM) databases, including the TOXNET system. | |
Record name | Rubrene | |
Source | DTP/NCI | |
URL | https://dtp.cancer.gov/dtpstandard/servlet/dwindex?searchtype=NSC&outputformat=html&searchlist=16080 | |
Description | The NCI Development Therapeutics Program (DTP) provides services and resources to the academic and private-sector research communities worldwide to facilitate the discovery and development of new cancer therapeutic agents. | |
Explanation | Unless otherwise indicated, all text within NCI products is free of copyright and may be reused without our permission. Credit the National Cancer Institute as the source. | |
Record name | Naphthacene, 5,6,11,12-tetraphenyl- | |
Source | EPA Chemicals under the TSCA | |
URL | https://www.epa.gov/chemicals-under-tsca | |
Description | EPA Chemicals under the Toxic Substances Control Act (TSCA) collection contains information on chemicals and their regulations under TSCA, including non-confidential content from the TSCA Chemical Substance Inventory and Chemical Data Reporting. | |
Record name | Naphthacene, 5,6,11,12-tetraphenyl- | |
Source | EPA DSSTox | |
URL | https://comptox.epa.gov/dashboard/DTXSID8060161 | |
Description | DSSTox provides a high quality public chemistry resource for supporting improved predictive toxicology. | |
Record name | 5,6,11,12-tetraphenylnaphthacene | |
Source | European Chemicals Agency (ECHA) | |
URL | https://echa.europa.eu/substance-information/-/substanceinfo/100.007.494 | |
Description | The European Chemicals Agency (ECHA) is an agency of the European Union which is the driving force among regulatory authorities in implementing the EU's groundbreaking chemicals legislation for the benefit of human health and the environment as well as for innovation and competitiveness. | |
Explanation | Use of the information, documents and data from the ECHA website is subject to the terms and conditions of this Legal Notice, and subject to other binding limitations provided for under applicable law, the information, documents and data made available on the ECHA website may be reproduced, distributed and/or used, totally or in part, for non-commercial purposes provided that ECHA is acknowledged as the source: "Source: European Chemicals Agency, http://echa.europa.eu/". Such acknowledgement must be included in each copy of the material. ECHA permits and encourages organisations and individuals to create links to the ECHA website under the following cumulative conditions: Links can only be made to webpages that provide a link to the Legal Notice page. | |
Retrosynthesis Analysis
AI-Powered Synthesis Planning: Our tool employs the Template_relevance Pistachio, Template_relevance Bkms_metabolic, Template_relevance Pistachio_ringbreaker, Template_relevance Reaxys, Template_relevance Reaxys_biocatalysis model, leveraging a vast database of chemical reactions to predict feasible synthetic routes.
One-Step Synthesis Focus: Specifically designed for one-step synthesis, it provides concise and direct routes for your target compounds, streamlining the synthesis process.
Accurate Predictions: Utilizing the extensive PISTACHIO, BKMS_METABOLIC, PISTACHIO_RINGBREAKER, REAXYS, REAXYS_BIOCATALYSIS database, our tool offers high-accuracy predictions, reflecting the latest in chemical research and data.
Strategy Settings
Precursor scoring | Relevance Heuristic |
---|---|
Min. plausibility | 0.01 |
Model | Template_relevance |
Template Set | Pistachio/Bkms_metabolic/Pistachio_ringbreaker/Reaxys/Reaxys_biocatalysis |
Top-N result to add to graph | 6 |
Feasible Synthetic Routes
Q1: How do we know about the different conformations of rubrene?
A1: X-ray diffraction studies, alongside computational chemistry techniques like Density Functional Theory (DFT), have been instrumental in understanding the different conformations rubrene and its derivatives can adopt [, ].
Q2: Is rubrene stable under ambient conditions?
A2: While rubrene exhibits good stability in inert environments, it is known to degrade under ambient conditions due to photo-oxidation, particularly in the presence of oxygen and light []. This sensitivity necessitates careful handling and processing techniques to preserve its desirable electronic properties.
Q3: How does rubrene's stability affect its applications?
A3: Rubrene's susceptibility to oxidation poses a challenge for its implementation in organic electronic devices. Researchers are exploring various strategies, including encapsulation and the development of more stable rubrene derivatives, to overcome this limitation and enhance its practical applicability [, ].
Q4: How does rubrene interact with different substrates?
A4: Rubrene's interaction with substrates is crucial for its performance in devices. For instance, rubrene deposited on cadmium arachidate multilayers shows enhanced crystalline growth, which is attributed to interactions between rubrene molecules and the substrate's surface morphology []. Conversely, rubrene interacts weakly with CH3NH3PbI3 perovskite substrates, leading to favorable energy level alignment for hole extraction in solar cells [].
Q5: What are the effects of doping rubrene with other materials?
A5: Doping rubrene with other materials like gold nanoparticles can enhance its optical and structural properties. For example, embedding gold nanoparticles in rubrene nanofibers via electrospinning resulted in a 2.5 times increase in photoluminescence, likely due to localized surface plasmon resonance [].
Q6: How does rubrene behave in mixed-host systems for OLEDs?
A6: In organic light-emitting diodes (OLEDs), rubrene doped into mixed hosts, like Alq3:rubrene, has demonstrated interesting electroluminescence properties. The rubrene molecules act as traps for charge carriers, leading to efficient energy transfer to red fluorescent dyes like DCJTB [, ].
Q7: What role does rubrene play in perovskite solar cells?
A7: Rubrene's favorable energy level alignment with perovskite materials makes it a promising hole transport layer in perovskite solar cells. Studies have shown that incorporating rubrene or rubrene-based bilayers can enhance the efficiency of these solar cells [, ].
Q8: Can rubrene be used in spintronic devices?
A8: Rubrene has garnered interest for spintronic applications due to its potential for long spin relaxation lengths. Research on rubrene nanowire spin valves has revealed intriguing high-field magnetoresistance effects and temperature-dependent spin relaxation, offering valuable insights for future device development [].
Q9: How is computational chemistry used to study rubrene?
A9: Computational methods like DFT are crucial for understanding rubrene's properties. For instance, DFT calculations revealed that conventional DFT methods overestimate the energetic preference for a twisted tetracene core in rubrene derivatives, highlighting the need for higher-level calculations to accurately predict the stability of different rubrene polymorphs [].
Q10: Are there any QSAR models for rubrene and its derivatives?
A10: While specific Quantitative Structure-Activity Relationship (QSAR) models for rubrene and its derivatives are not extensively discussed in the provided research, the studies highlighting the impact of chemical modifications on rubrene's crystal structure and electronic properties lay the groundwork for developing such models in the future []. These models could be valuable tools for predicting the properties of new rubrene derivatives.
Haftungsausschluss und Informationen zu In-Vitro-Forschungsprodukten
Bitte beachten Sie, dass alle Artikel und Produktinformationen, die auf BenchChem präsentiert werden, ausschließlich zu Informationszwecken bestimmt sind. Die auf BenchChem zum Kauf angebotenen Produkte sind speziell für In-vitro-Studien konzipiert, die außerhalb lebender Organismen durchgeführt werden. In-vitro-Studien, abgeleitet von dem lateinischen Begriff "in Glas", beinhalten Experimente, die in kontrollierten Laborumgebungen unter Verwendung von Zellen oder Geweben durchgeführt werden. Es ist wichtig zu beachten, dass diese Produkte nicht als Arzneimittel oder Medikamente eingestuft sind und keine Zulassung der FDA für die Vorbeugung, Behandlung oder Heilung von medizinischen Zuständen, Beschwerden oder Krankheiten erhalten haben. Wir müssen betonen, dass jede Form der körperlichen Einführung dieser Produkte in Menschen oder Tiere gesetzlich strikt untersagt ist. Es ist unerlässlich, sich an diese Richtlinien zu halten, um die Einhaltung rechtlicher und ethischer Standards in Forschung und Experiment zu gewährleisten.